Introduction
For thousands of years, plants from the Sonoran Desert in Northwestern Mexico have adapted to survive the hostile climatic conditions, including long droughts (Sánchez-Escalante, 2007b). The number of plant species in this place is higher than in temperate ecosystems (Sánchez-Escalante, 2007b), being considered a subtropical desert that promotes the development of a great number of plants with pharmacological potential, as evidenced by research carried out in the last years (Torres-Moreno et al., 2015; Vidal Gutiérrez et al., 2020). Among the great diversity of plants in the Sonoran Desert, we also find some species of the genus Parkinsonia L. such as P. microphylla Torr. (palo verde), P. florida (Benth. ex A. Gray) S. Watson (palo verde, palo azul), P. aculeata L. (bagote, bacaporo or guaco) and P. praecox (Ruiz & Pav.) Hawkins (brea, palo brea o árbol del manteco) are extensively distributed in this area (Sánchez-Escalante, 2007a).
The genus Parkinsonia has been studied for its biological properties. Parkinsonia aculeata has been recognized for its antioxidant, antimutagenic, antibacterial, antifungal, anti-inflammatory and antitumor effects (Saha et al., 2010a; Singh et al., 2013; Al-Youssef and Hassan, 2015; Sharma et al., 2016). Chemical studies on P. aculeata showed that this plant is constituted by essential oils (Al-Youssef and Hassan, 2015), flavonoids and polyphenolic compounds (Abdelaziz et al., 2020), with pharmacological potential. On the other hand, gold and silver nanoparticles made from leaves extracts of P. florida have shown antibacterial activity against Staphylococcus aureus Rosenbach 1884 and Escherichia coli (Escherich, 1885) (López-Millán et al., 2019). This suggests that Parkinsonia spp. are a cost-effective option for the development of new pharmaceutical formulations, due to their wide distribution throughout the American continent, their accelerated growth, as well as the large variety of bioactive compounds associated with several biological activities found in representatives of this genus (Sánchez-Escalante, 2007a; Al-Youssef and Hassan, 2015; Abdelaziz et al., 2020; Romão and Mansano, 2021).
Parkinsonia praecox (Fabaceae) is a plant of a great interest for the variety of uses and goods it provides (Fig. 1). Traditionally, it is used as forage, cnstruction basis and fuel. Furthermore, its vascular exudates called “gums” are commonly utilized as a substitute of arabic gum, glue, as well as in traditional medicine for treatment of bronchial conditions (Labrada-Aranda, 2005). In Argentina, P. praecox brea gum commonly named “woodland candy, dulce del bosque” has been used by villagers since pre-Colombian time without any harmful consequences produced in health (von Müller et al., 2009).
Studies on P. praecox showed that this plant has antibacterial, antifungal, and cytotoxic effect on Artemia salina (Linneaus 1758) (Torrealba, 2008). The toxicological evaluation showed that brea gum does not induce toxicological effects in mice when it is administered as a food additive (von Müller et al., 2009). Phytochemical analysis demonstrated that P. praecox is constituted by alkaloids, triterpenes, sterols, saponins, flavonoids and polyphenolics (Torrealba, 2008). However, its antiproliferative and antioxidant effect remains unexplored. In this study, with the aim to generate new information about the chemical composition and the biological potential of P. praecox, phytochemical screening, phenolic profile, and the antioxidant and antiproliferative effect of its stems, berries and flowers were investigated. The results reported here position P. praecox as a new option for the search of new compounds with antiproliferative and antioxidant potential.
Materials and Methods
Plant and extract elaboration
Parkinsonia praecox stems, berries and flowers were collected in ejido San Pablo, Sonora, Mexico, in April 2019 (28°48'43.4''N 110°57'36.8''W). Samples were identified (voucher number 102769) by Dr. José Angel Villareal Quintana (specialist on plant taxonomy) and deposited in the Herbarium of the Universidad Autonóma Agraria Antonio Narro (ANSM), Saltillo, Coahuila, Mexico. The samples were dried at room temperature and extracted with methanol (1:10 w/v) during four days with occasional stirring. Obtained extracts were filtered (Whatman No. 4) and the solvent was removed under vacuum (IKA, RV 10 digital, Wilmington, NC, USA) at 40 °C, followed by storage at -20 °C.
Phytochemical screenings
Phytochemical screenings of P. praecox extracts were performed to determine the presence of terpenes, flavonoids, tannins, saponins, cardiac glycosides, sugars, free aminoacids, alkaloids, phenolic compounds, coumarin and quinones following published methods.
Terpene identification
Identification of these compounds was carried out through the Lieberman-Burchard test (Burke et al., 1974; Xiong et al., 2007; Adu et al., 2019; Wutsqa et al., 2021). Briefly, 0.05 g of P. praecox extracts were dissolved in 1 ml of chloroform with a further addition of 1 ml of acetic anhydride. Subsequently, two drops of concentrated H2SO4 (97%, MerckMillipore, Darmstadt, Germany) were added to the sample. A green color change indicated the presence of terpenes (Akter et al., 2016; Suurbaar et al., 2017).
Flavonoids identification
Flavonoids identification was carried out through the Shinoda reaction (Cheng-Yong et al., 2016; Ribeiro et al., 2017; Musman et al., 2019, Kumkhale et al., 2020). An aliquot (0.05 g) of P. praecox extracts was dissolved in ethanol (absolute, MerckMillipore, Darmstadt, Germany). Two drops of concentrated HCl (37%, MerckMillipore, Darmstadt, Germany) were added followed by a chip of magnesium metal. Appearance of a magenta color indicated the presence of flavonoids (Akter et al., 2016; Suurbaar et al., 2017; Gollo et al., 2020).
Tannins identification
An amount (0.05 g) of P. praecox extracts was dissolved in 2 ml of Milli-Q-water and filtered (Whatman No. 4). A couple of drops of 10% ferric chloride (Iron (III), chloride 97%, Sigma Aldrich, St. Louis, USA) solution were added to the sample and the formation of a blue-black color indicated the presence of tannins (Akter et al., 2016; Suurbaar et al., 2017; Gollo et al., 2020).
Saponins identification
Saponins were identified using the Rosenthaler’s reaction (Borkar et al., 2016; Le et al., 2018). Briefly, 0.02 g of P. praecox extracts were placed in a watch glass and two drops of Rosenthaler’s reagent and two drops of concentrated H2SO4 (97%, MerckMillipore, Darmstadt, Germany) were added. Formation of red to violet color in the sample indicated the presence of saponins (Suurbaar et al., 2017).
Cardiac glycosides identification
Identification of cardiac glycosides was performed following the Keller-Killiani reaction (DagerAlbalawi, 2016; Oladele et al., 2021). Briefly, 0.05 g of P. praecox extracts were dissolved in a solution formed by 1 ml of glacial acetic acid (>99%, Sigma Aldrich, St. Louis, USA) and two drops of 2% ferric chloride (Iron (III) chloride 97%, MerckMillipore, Darmstadt, Germany). Afterwards, 1 ml of H2SO4 was slowly added and the formation of a red ring indicated the presence of cardiac glycosides (Suurbaar et al., 2017).
Alkaloids identification
An aliquot (0.05 g) of P. praecox extracts with 10 ml of 1% HCl (37%, MerckMillipore, Darmstadt, Germany) was heated to boil and then filtered. Then, two drops of Wagner’s reagent were added to 2 ml of filtrate (Whatman No. 4). A brown precipitate indicated the presence of alkaloids. Afterwards, two drops of acidified Mayer’s reagent were treated with 2 ml of filtrate and yellowish cloudy precipitate indicated the presence of alkaloids (Akter et al., 2016; Suurbaar et al., 2017).
Phenolic compounds identification
Briefly, 0.05 g of P. praecox extracts were dissolved in 1 ml of Milli-Q-water, followed by two drops of 2% ferric chloride (Iron (III) chloride 97%, Sigma Aldrich, St. Louis, USA) solution. Positive results were associated with blue, green, red o purple colorations (Suurbaar et al., 2017).
Coumarin identification
The identification of coumarins was carried out with a reaction of potassium hydroxide. Briefly, P. praecox extracts (0.05 g) were dissolved in 1 ml of boiling Milli-Q-water. Later, a portion of this solution was taken with a capillary and placed on a paper filter piece and allowed to dry. Once the sample was dried on the filter paper, one drop of potassium hydroxide (99.95%, Sigma Aldrich, St. Louis, USA) solution (0.5 M) was added and observed under a UV lamp at 366 nm (CAMAG UV 4 lamp, Muttenz, Switzerland). Yellow fluorescence coloration was considered positive for coumarine (Suurbaar et al., 2017).
Quinones identification
One drop of concentrated sulfuric acid (97%, MerckMillipore, Darmstadt, Germany) was added to 0.02 g of P. praecox extracts. The formation of red color indicated the presence of anthraquinones (Suurbaar et al., 2017).
Sugars identification
Identification of glycosides in the extract was carried out using a reaction with sodium hydroxide. For that, 0.05 g of P. praecox extracts were dissolved in 1 ml of Milli-Q-water and two drops of the sodium hydroxide (98%, Sigma Aldrich, St. Louis, USA) solution were also added. A yellow coloration indicated the presence of glycosides in the sample (Suurbaar et al., 2017).
Free aminoacids identification
Briefly, 0.05 g of P. praecox extracts were dissolved in 1 ml of ninhydrin reagent (Sigma Aldrich, St. Louis, USA), and the solution was boiled in a water bath for 10 min. Color-change expectations were according with the presence of certain type of amino group: bluish-violet color changes for those with primary amino group, yellow for proline and hydroxyproline, and brown for asparagine which has an amino group in the side chain (Suurbaar et al., 2017).
Folin Ciocalteu assay
Parkinsonia praecox extracts (10 µl:1 mg/ml) were combined with distilled water (80 μl), Folin-Ciocalteu reagent (2 N, Sigma-Aldrich, St. Louis, USA) 0.25 N (40 μl), sodium carbonate (>99.5%, Sigma-Aldrich, St. Louis, USA) (60 μl, 5% in distilled water), and distilled water (80 μl). Samples were stored for 1 h (darkness) at 25 °C. Finally, absorbance was read on a microplate reader (750 nm) (ThermoScientific Multiskan GO, Osterode, Germany). Results were expressed as mg of gallic acid equivalent (GAE)/g dried weight (d.w.) (Velazquez et al., 2007).
UPLC-DAD analysis
Analysis of phenolic compounds of P. praecox extracts were determined by the UPLC™ H-class system (Acquity, Waters Co., Milford, USA) equipped with a photodiode array extended λ (PDA eλ) detector, an Acquity UPLC™ BEH C18 VanGuard precolumn (130 Å, 1.7 µm, 2.1 mm × 5 mm), and an UPLC™ BEH C18 column (1.7 µm, 3.0 × 100 mm) with a column temperature of 60 °C and an auto sampler set at 5 °C. The mobile phase was constituted by 0.5% formic acid in water (solvent A) (solution 50% in water, Sigma-Aldrich, St. Louis, USA) and methanol (solvent B) (HPLC grade, JT Baker Inc, Phillipsburg, USA). The elution was performed at different conditions: 0 min, flow 0.4 ml/min, 80% solvent A; 0.25 min, flow 0.150 ml/min, 80% solvent A; 5 min, flow 0.200 ml/min, 80% solvent A; 12 min, flow 0.180 ml/min, 55% solvent A; 25 min, flow 0.100 ml/min, 0% solvent A; 26 min, flow 0.200 ml/min, 60% solvent A; 27 min, flow 0.400 ml/min, 80% solvent A; 30 min, flow 0.400 ml/min, 80% solvent A. The detection of phenolic compounds was performed by the retention time of standards and their absorption spectrum (280 nm, 320 nm, 360 nm and 380 nm). Analyzed compounds were caffeic acid, cinnamic acid, chlorogenic acid, ellagic acid, ferulic acid, gallic acid, 2-hydroxycinnamic acid, p-coumaric acid, 3-hydroxybiphenyl, protocatechuic acid, rosmarinic acid, sinapic acid, syringic acid, vanillic acid, apigenin, catechol, catechin hydrate, epicatechin, epigallocatechin gallate, gallocatechin gallate, kaempferol, kaempferol 3-β-glucopyranose, luteolin, maclurin, mangiferin, myricetin, naringenin, naringin, neohesperidin, phloretin, phloridzin dyhydrate, pyrogallol, polydatin, quercetin-3-β-d-glucoside, quercetin dihydrate, resveratrol and rutin. Quantification of phenolic compounds was performed with standard calibration curves, and results were reported as µg/g d.w. (Velderrain-Rodríguez et al., 2018). Identified compounds in the extracts were quercetin (retention time: 17.57-17.68 min; quantification range: 0.584 to 149.5 µg/ml; regression type: lineal; r2 value: 0.9997) (Sigma-Aldrich, St. Louis, USA) and p-coumaric acid (retention time: 10.13-10.42 min; quantification range: 0.756 to 96.75 µg/ml; regression type: lineal; r2 value: 0.9998) (Sigma-Aldrich, St. Louis, USA).
DPPH assay
Parkinsonia praecox extracts (100 µl) were mixed with DPPH (Sigma-Aldrich, St. Louis, USA) solution at 300 µM (100 µl). After 30 min stored (in darkness) at 25 °C the absorbance was read on a microplate reader (517 nm) (ThermoScientific Multiskan GO, Osterode, Germany). Results were reported as IC50 (concentration to inhibit the 50% of DPPH radical, µg/ml) and µM Trolox Equivalent (TE)/g d.w. (Usia et al., 2002)
ABTS assay
ABTS + assay was performed by mixing 5 ml of ABTS (Sigma-Aldrich, St. Louis, USA) (7 mM) with 88 µl of K2S2O8 (>99%, Sigma-Aldrich, St. Louis, USA) (0.139 mM) for 16 h (in darkness). The absorbance of the radical was adjusted to 0.700±0.02 at 754 nm. Parkinsonia praecox extracts (5 µl) were combined with adjusted radical (245 µl) and then the samples were incubated (5 min) in darkness. Finally, the absorbance was read at 754 nm (Fluostar Omega microplate reader, BMG Labtech, Ortenberg, Germany). Results were reported as μM Trolox Equivalent (TE)/g d.w (Modified method of Re et al., 1999).
ORAC assay
One hundred and fifty μl of fluorescein (Free acid, Sigma-Aldrich, St. Louis, USA) (10 nM), 25 μl of phosphate buffer (Sodium phosphate dibasic heptahydrate and hydrated monobasic sodium phosphate; Fagalab, Mocorito, Sinaloa, Mexico) (75 mM, pH 7.4) as blank, and 25 μl (50 μg/ml) of P. praecox extracts were mixed. AAPH (97%, Sigma-Aldrich, St. Louis, USA) (240 mM) was also incorporated to the mixture and incubated at 37 °C (15 min). Fluorescence was read every 90 s for 1.5 h at an excitation wavelength of 485 nm and an emission wavelength of 530 nm on a microplate reader (Fluostar Omega microplate reader, BMG Labtech, Ortenberg, Germany). Results were reported as μM Trolox Equivalent (TE)/g dried weight (d.w.) (modified method of Ou et al., 2001).
FRAP assay
FRAP reagent was elaborated with the combination of ten volumes of acetate buffer (300 mM, pH 3.6), 1 volume of TPTZ (>98%, Sigma-Aldrich, St. Louis, USA) (40 mM, dissolved in 40 mM HCl), and 1 volume of ferric chloride (97%, Sigma-Aldrich, St. Louis, USA) (20 mM, dissolved in water). Parkinsonia praecox extracts (20 μl) were combined with FRAP reagent (280 μl). After 30 min (in darkness) at 25 °C the samples were read using a microplate reader (630 nm) (ThermoScientific Multiskan GO, Osterode, Germany). Results were reported as µM of Fe(II)/g d.w. (Benzie and Strain, 1996).
Cell culture
Cell lines were cultured in DMEM (Dulbecco´s Modified Eagle Medium) (Sigma-Aldrich, St. Louis, USA) supplemented with 5% heat-inactivated serum feral bovine (ByProducts, Guadalajara, Mexico) and penicillin (Sigma-Aldrich, St. Louis, USA) (100 U/ml) in 25 cm2 culture dishes. The cultures were maintained in an Isotherm incubator (Fisher Scientific, Waltham, USA) under the standard conditions (5% of CO2 at 37 °C and 95% of relative humidity). A549 (non-small-cell lung cancer cells), MDA-MB-231 (triple negative breast cancer), PC-3 (adenocarcinoma prostate cancer grade IV) and HeLa (human cervical cancer) and L929 (non-cancerous subcutaneous connective tissue) were obtained from the American Type Culture Collection (ATCC; Rockville, USA).
MTT assay
Fifty μl (1 × 104 cells) were placed in each well of a flat 96-well plate and incubated (24 h, 37 °C, 5% of CO2 atmosphere). Afterwards, the cells were treated with P. praecox extracts (50 μl) and incubated during 48 h under the standard conditions. Previously, extracts were dissolved in DMSO and re-suspended in DMEM (Sigma-Aldrich, St. Louis, USA) to give a final DMSO (>99.9%, Sigma-Aldrich, St. Louis, USA) concentration of 0.25% (that showed no interference with the cell lines’ growth). Then, DMEM (50 μl) containing different concentrations of the extracts were added and the cell cultures were incubated for 48 h. Subsequently, MTT (Sigma-Aldrich, St. Louis, USA) solution (10 μl, 5 mg/ml) was added to each well. Next, formazan crystals generated by metabolically active cells, were dissolved with acid isopropanol. The absorbance of microplates was read at 570 and 630 nm (iMark microplate absorbance reader, BioRad, Laboratories, Cd. Mx, Mexico). Results were reported as IC50 values (IC50 is defined as the required concentration to inhibit 50% of cell proliferation), and were calculated using nonlinear regression analysis in Microsoft Excel software (Hernandez et al., 2007; Torres-Moreno et al., 2015).
Statistical analysis
One-way analysis of variance was performed for the obtained data using the NCSS Statical software v. 2007 (NCSS Statical software, 2021). Tukey-Kramer tests were used to mean comparisons. Significance level was p≤0.05. Correlation analysis was performed using Pearson correlation.
Results
Phytochemical screenings
Phytochemicals screening of P. praecox extracts is shown in Table 1. The analysis consists of the determination of 11 groups of secondary metabolites, where PPB presented the highest quantity of metabolites, among them terpenes, phenolic compounds, flavonoids, tannins and sugars. PPF and PPS exhibited similar bioactive compounds profile comparing with PPB, except for terpenes. It is important to highlight that these qualitative tests showed a pronounced intensity in the reaction corresponding to phenolic compounds for all cases, probably being the main group of compounds in the extracts.
Table 1: Phytochemical screening of Parkinsonia praecox (Ruiz & Pav.) Hawkins extracts. PPF: Parkinsonia praecox flower extract; PPB: Parkinsonia praecox berry extract; PPS: Parkinsonia praecox stem extract. - (absence); + (presence).
Secondary metabolite | PPF | PPB | PPS |
---|---|---|---|
Terpenes | - | + | - |
Flavonoids | - | + | + |
Tannins | + | + | + |
Saponins | - | - | - |
Cardiac glycosides | - | - | - |
Sugars | + | + | + |
Free amino acids | - | - | - |
Alkaloids | - | - | - |
Phenolic compounds | + | + | + |
Coumarin | - | - | - |
Quinones | - | - | - |
Quantification of phenolic compounds
The phytochemical screening suggests that phenolic compounds are the most predominant bioactive compounds present in P. praecox extracts; phenolic compounds were quantified by the Folin-Ciocalteu method. Results evidenced that PPS presented higher amount of phenolic content (p<0.05) compared to PPF and PPB, which presented 2.9 and 1.7 lower concentration, respectively (Fig. 2).
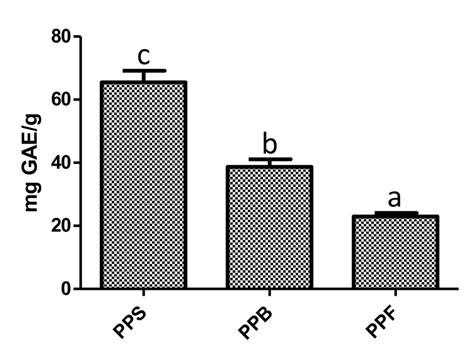
Figure 2: Phenolic quantification of Parkinsonia praecox (Ruiz & Pav.) Hawkins extract. PPS: Parkinsonia praecox stem extract; PPB: Parkinsonia praecox berry extract; PPF: Parkinsonia praecox flower extract. Data are presented as the mean ± standard deviation of three independent experiments. a-cBars with different letter in each graph represent significantly different values (p<0.05).
UPLC-DAD analysis
To identify the phenolic compounds presented in P. praecox extracts, UPLC-DAD analysis was carried out. For chromatogram interpretation, a contrasting analysis using a database of 37 phenolic compounds (phenolic acids and flavonoids) was performed. The analysis of PPS allowed to identify the presence of quercetin with a concentration of 218.8 µg/g (Table 2), while in PPF, p-coumaric acid at concentration of 176.4 µg/g was identified (Table 2). Regarding PPB, it was not possible to identify any flavonoid or phenolic acid. This suggests that different phenolic compounds are present in PPB, which were not identified due to the limitations of the database.
Table 2: Concentration (μg/g) of identified phenolic compounds in Parkinsonia praecox (Ruiz & Pav.) Hawkins extracts. PPS: Parkinsonia praecox stem extract; PPB: Parkinsonia praecox berry extract; PPF: Parkinsonia praecox flower extract. Data are presented as the mean ± standard deviation of two independent experiments. ND: no detected.
Compound | PPS | PPB | PPF |
---|---|---|---|
Quercetin p-coumaric acid | 218.8±1.5 ND | ND ND | ND 176.4±2.6 |
Antioxidant activity
Antioxidant potential of evaluated extracts of P. praecox is shown in Table 3. Results obtained by the DPPH method evidenced that P. praecox extracts exhibited the capability to scavenge this free radical. PPS presented the highest (p<0.05) antioxidant potential comparing with other evaluated extracts. Additionally, PPS required the lowest (p<0.05) concentration (137 µg/ml) to stabilize the 50% of DPPH radical (IC50), while in case of PPB and PPF an increment of the concentration was required (1.4 and 2.2 time, respectively) to show a similar effect.
Table 3: Antioxidant activity of Parkinsonia praecox (Ruiz & Pav.) Hawkins extracts. PPS: Parkinsonia praecox stem extract; PPB: Parkinsonia praecox berry extract; PPF: Parkinsonia praecox flower extract; DPPH: 2,2-diphenyl-1-picrylhydrazyl; TEAC: trolox equivalent antioxidant capacity; FRAP: ferric reducing antioxidant power assay. Data are presented as the mean ± standard deviation of three independent experiments. a-cDifferent letters within the same column indicate significant differences (p<0.05).
Treatment | Antioxidant assay | |||||
---|---|---|---|---|---|---|
µM ET/g | DPPH | IC50 (µg/ml) | TEAC µM ET/g | ORAC µM ET/g | FRAP µM Fe(II)/g | |
PPS | 262.3±30.7c | 137±12.5a | 39.5±0.2c | 1777.7±45.5c | 935.6±52.7c | |
PPB | 179.8±2.8b | 190.9±6.6b | 26.3±0.1b | 920.6±33.9b | 467.7±17.8b | |
PPF | 112.7±3.8a | 302.3±7.3c | 24.7±0.1a | 750.3±41.3a | 306.9±14.8a |
On the other hand, P. praecox extracts were able to stabilize the ABTS radical (Table 3). The PPS extract was the one with the highest ABTS radical stabilizing activity (p<0.05) compared to the PPB and PPF extracts, which exhibited 1.50 and 1.59 lower stabilizing capability, respectively.
Interestingly, P. praecox extracts, in addition to stabilizing synthetic radicals (DPPH and ABTS), were also able to inhibit biological radicals such as hydroxyl radical, which was evaluated through the ORAC method (Table 3). The results showed that PPS presented the highest ORAC values (p<0.05), which indicates greater effectiveness in the inhibition of the hydroxyl radical. The PPB and PPF extracts also showed antioxidant capacity by this method; however, it was 48.2 and 57.8% less effective than PPS.
Reducing antioxidant potential of P. praecox extracts was also demonstrated (Table 3). The evaluated extracts showed capability to reduce the ferric-TPTZ complex to ferrous-TPTZ complex. However, the PPS extract exhibited the highest (p<0.05) reducing power, presenting 200.04% and 304.9% greater antioxidant potential than PPB and PPF, respectively.
Correlation analysis was carried out between the antioxidant activity (DPPH, ABTS, ORAC and FRAP) and the content of phenolic compounds in P. praecox extracts (Table 4). Obtained results demonstrated that there is a strong correlation between the antioxidant activity of the P. praecox extracts and the content of total phenols, since the r values higher than 0.96 were obtained in all cases.
Table 4: Correlation (r) between total phenolic content (TPC) and antioxidant activity of Parkinsonia praecox (Ruiz & Pav.) Hawkins. DPPH: 2,2-diphenyl-1-picrylhydrazyl; ABTS: 2,2′-azino-bis(3- ethylbenzothiazoline-6-sulfonic acid; ORAC: assay measures antioxidant capacity; FRAP: ferric reducing antioxidant power assay.
Antioxidant method | ||||
---|---|---|---|---|
DPPH | ABTS | ORAC | FRAP | |
TPC | 0.96 | 0.96 | 0.97 | 0.99 |
Antiproliferative activity
In this study, we evaluated by MTT assay the antiproliferative effect of P. praecox extracts against a panel of cancer cell lines and against the non-cancerous L929 cell line (Table 5). In general, prostate cancer PC-3 cells were the most sensitive for all the treatments; however, the highest activity was observed for PPF followed by PPS and PPB. L929 cells were most resistant to PPF and PPB, which suggests that these extracts are more selective than PPS. On the other hand, PPS showed moderate activity against MDA-MB-231 and HeLa cells; nevertheless, the proliferation of L929 cells was inhibited most efficiently by this extract.
Table 5: Antiproliferative activity of Parkinsonia praecox (Ruiz & Pav.) Hawkins. PPS: Parkinsonia praecox stem extract; PPB: Parkinsonia praecox berry extract; PPF: Parkinsonia praecox flower extract. Data are presented as the mean ± standard deviation of three independent experiments. a-bDifferent letters within the same column indicate significant differences (p<0.05). IC50 not determined at the máximum tested concentration (400 μg/ml).
Treatments | Cell lines IC50 (μg/ml) | ||||
---|---|---|---|---|---|
A549 | MDAMB- 231 | PC-3 | HeLa | 1929 | |
PPS | 341.3 ± 13.6 | 147.3 ± 8.9 | 78.87 ± 0.5a | 121.65 ± 7.7a | 93.29 ± 5.9 |
PPB | >400 | >400 | 94.21 ± 0.3b | 220.20 ± 13.2b | >400 |
PPF | >400 | >400 | 68.38± 9.1a | 222.45 ± 26.6b | >400 |
Discussion
Natural products have been used for the treatment of different illnesses worldwide. This trend is still moving on and currently, 80% of the human population uses them as primary treatment for different health conditions (Sharifi-Rad et al., 2020). Effectiveness of some of these products has been demonstrated for the development of drugs, due to the presence of highly effective chemical compounds (Newman and Cragg, 2020; Zhang et al., 2020).
A previous study demonstrated the presence of several chemical compounds in P. praecox, such as alkaloids, triterpenes, sterols, saponins, flavonoids and polyphenolics (Torrealba, 2008). Additionally, other Parkinsonia species such as P. aculeata presented a wide variety of bioactive compounds, such as terpenes, saponins, steroids, phenolic compounds, flavonoids, tannins, alkaloids, proteins, amino acids, glucosides, and carbohydrates (Leite et al., 2010; Saha et al., 2010b; Sharma and Vig, 2014; Qureshi, 2017). Our study demonstrated that P. praecox presented minor groups of secondary metabolites, which could be associated with the analyzed plant genus, metabolism, biotic and abiotic plant conditions, type of extraction method and solvents used. These factors determine the bioactive compounds profile that will be present in natural extracts (Azmir et al., 2013; Ochoa-Velasco et al., 2017).
Phytochemical analysis showed that phenolic compounds are the most predominant bioactive compounds present in P. praecox. The obtained results in this study are consistent with previously reported for other analyzed species of the genus, such as P. aculeata, which presented phenolic content values between 10 to 288 mg GAE/g (Aranda-Souza et al., 2012; Sharma and Vig, 2013, 2014; Muddathir et al., 2017; Qasim et al., 2017). In addition, this study shows for the first time the presence of specific compounds in P. praecox, which could support the biological potential of this plant.
The present study provides novel information about the antioxidant potential of P. praecox, being the first report about its biological activity. In addition, it is important to highlight that PPS could be classified as an extract with medium antioxidant potential because it presented an IC50 value lower than 150 µg/ml, while the other extracts exhibited low antioxidant potential (Fidrianny et al., 2015). Additionally, PPS is a natural extract with high reducing potential because it presented FRAP value >500 µM Fe(II)/g, which is the limit value to consider it a natural source with this characteristic (Wong et al., 2006).
On the other hand, the obtained antioxidant results in this study agree with the previously reported ones for P. aculeata. Aranda-Souza et al. (2012), Sharma and Vig (2013; 2014), and Sharma et al. (2017) reported that P. aculeata extracts obtained with different solvents (methanol, butanol, acetone, chloroform and hexane) exhibited capability to stabilize the DPPH radical (IC50: 10.8 to <1000 µg/ml), hydroxyl radical (200 µg/ml of extracts inhibited the hydroxyl radical formation between 20.3 to 80%) and reduce metals (461 to <498 µM Fe(II)/g; IC50: 162.6 to <200.7 µg/ml).
Elevated rates of free radicals and transition metals in biological systems cause indiscriminate damage of biomolecules such as DNA, leading to the development of several pathologies like neurodegenerative diseases, atherosclerosis, cancer and others (Collin, 2019). In this regard, P. praecox (especially PPS) showed the capability to stabilize synthetic and biological radicals, as well as to reduce metals. These extracts can represent a natural alternative that could be used to inhibit the formation of free radicals and interrupt their propagation.
Antioxidant activity of natural products is usually related to the presence of chemical compounds in plants, specifically with phenolic compounds (Vidal Gutiérrez et al., 2020). This suggests that the antioxidant activity of P. praecox is associated with the presence of these types of compounds. The highest antioxidant activity of PPS can be associated with the presence of phenolic compounds, mainly quercetin. Different studies have demonstrated that this flavonoid is one of the most potent antioxidant agents in nature (Sharma et al., 2018). The antioxidant mechanism of quercetin is related with the presence of hydroxyl groups in the catechol ring (substitution in position 3’ and 4) and chromane ring (substitution in position 3). In addition, the C ring plays an important role, especially the double bond between C2-C3. This characteristic promotes the electron or proton donation to exert antioxidant effect, and then confers stability by resonance effect (Ulusoy and Sanlier, 2020). Likewise, the antioxidant effect of PPF could be associated with the presence of p-coumaric acid, whose mechanism of action is due to the presence of the hydroxyl group in C3 of the phenolic ring and to the length of its hydrocarbon chain (Jing et al., 2012). These characteristics allow to donate protons or electrons to stabilize free radicals and reduce metals, as well as to stabilize the produced phenolic radicals (Jing et al., 2012). In the case of PPB, its antioxidant effect can be related with the presence of phenolic compounds which were not identified in this study. In addition to phenolic compounds, terpenes are also known for their antioxidant activity. Therefore, the antioxidant activity of PPB can be related with the presence of these types of compounds, whose mechanism of action is associated with the presence of hydroxyl or methoxyl groups in the phenolic ring (Gonzalez-Burgos and Gómez-Serranillos, 2012).
On the other hand, nowadays the search of new selective and effective treatments against cancer continues to be a priority for public health worldwide (Zhong et al., 2021). In this context, medicinal plants provide a good option for the search of new anticancer drugs (Block et al., 2015). According to the National Cancer Institute (NCI, USA) guidelines (Grever et al., 1992), a crude extract could be considered cytotoxic when it inhibits the proliferation of a cell line with a IC50 value <30 µg/ml (Grever et al., 1992). However, even though P. praecox extracts inhibited cell proliferation with IC50 values >30 µg/ml, the study of the antiproliferative effect of its fractions or isolated compounds cannot be ruled out. Previous research about plants has shown the possibility of compound isolation with great antiproliferative effect from methanolic extracts that inhibit a particular cancer cell line proliferation with IC50 values around 130 µg/ml (Torres-Moreno et al., 2015). Hence, P. praecox extracts could be considered to obtain antiproliferative compounds through a bioguided separation.
In several studies, it has been shown that there is a direct correlation between the phenolic composition and the antiproliferative activity of plants (Maya-Cano et al., 2021). The phytochemical screening showed that P. praecox extracts are constituted by phenolic compounds such as quercetin and p-coumaric acid that have been identified in PPS and PPF, respectively. Antiproliferative, apoptotic and cell cycle arrest capability of quercetin and p-coumaric acid on cancer cells have been demonstrated (Wang et al., 2012; Abotaleb et al., 2020). Wang et al. (2012) reported that quercetin reduces the proliferation of the prostate cancer cell lines PC-3 (≈10%) and LNCaP (in ≈20 and 30%) at 5 and 10 µM and induces apoptosis and cell cycle arrest in S and G2 M phases. P-coumaric acid reduces the proliferation of the ovarian cancer cells A-2780 with IC50 of 3.64 × 10-2 mg/ml (Shahwar et al., 2015). The proliferation of the colon cancer cells HT-29 was reduced by p-coumaric acid with IC50 values of 1600 μmol/l and 1000 μmol/l at 48 and 72 h, respectively, whereas the proliferation of SW480 colon cancer cells was inhibited with IC50 of 1400 μmol/l and 1000 μmol/l at 48 and 72 h. The inhibition of HT-29 and SW480 cell proliferation was associated with the capability of p-coumaric acid to induce apoptosis through the inhibition of Gr78 via activation of PERK-eIF2α-ATF-4-CHOP pathway (Abotaleb et al., 2020)
Hence, these compounds, synergistically or additively with other unidentified metabolites, could be contributing to the antiproliferative effect of PPF and PPS. On the other hand, terpenes are a large class of natural compounds with anticancer effect (Huang et al., 2012). Phytochemical analysis showed that terpenes are only present in PPB (Table 1); this suggests that the antiproliferative effect of PPB is due to the combined action of terpenes and phenolic compounds (Wright, 2001; Gul et al., 2016).
Conclusions
The present study demonstrated that P. praecox could be a source of several chemical compounds with biological potential. Additionally, a possible relation between antioxidant and antiproliferative activity is suggested as a consequence of the presence of phenolic compounds. Therefore, despite showing moderate potential, this plant could be used to obtain fractions or to purify compounds with this capability, representing an alternative for different pharmacological applications views.