INTRODUCTION
Ctenophora is a diverse phylum of gelata consisting of about 190 described species, with estimates for the number of additional undescribed species being roughly equivalent (Appeltans et al. 2012). Molecular and phylogenomic analyses of ctenophores have indicated that they may be the sister group to all other animals (Dunn et al. 2008, Whelan et al. 2017, but see Simion et al. 2017). Dunn et al. (2015) highlighted that nonbilaterian phyla do not only possess simple traits but also complex and unique traits, as do bilaterians, making it important to describe traits across all animal phyla in order to properly compare evolutionary relationships and diversification.
Ctenophores are the only organisms that possess colloblasts, specialized adhesive structures for predation. The diversification of feeding behaviors in ctenophores is in part due to the presence or absence of tentacles containing colloblasts, and the manner in which they are arranged (Komai 1922, Mackie et al. 1988, Emson and Whitfield 1991, Matsumoto and Harbison 1993, Haddock 2007). Through the use of electron microscopy, morphometrics of colloblasts have been recorded for some species (Bargmann et al. 1972; Benwitz 1978; Franc 1978; Mackie et al. 1988; Emson and Whitfield 1991; Carré and Carré 1993a, b; Eeckhaut et al. 1997), but only one study has compared variation in colloblasts across a small portion (4 species from 4 families) of the phylum (Franc 1978). Colloblast polymorphisms within a species have only been observed in Minictena luteola (Carré and Carré 1993b). The vocabulary associated with colloblasts has long been confused, but von Byern et al. (2010) have recently clarified a standard terminology, which is used herein (Fig. 1). The goal of the present study is to survey the diversity of colloblast characteristics across the phylum through the use of scanning electron microscopy (SEM) and morphometric analyses.
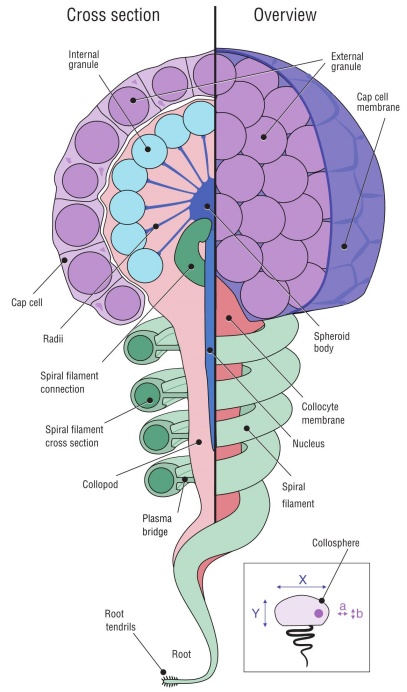
Figure 1 Colloblast morphology and schematic of how morphometrics were recorded (inset). The colloblast is made up of 2 different cell types that merge in the latter stages of development. The collocyte is the primary cell that comprises the colloblast and contains a majority of the organelles that are key to the function of colloblasts during predation. Cap cells produce external secretion granules and form the cap cell membrane that covers the surface of the developing colloblast. Internal secretion granules are produced by the collocyte and are anchored to the spheroid body via the radii. The elongated nucleus is located beneath the spheroid body and extends downwards into the collopod. The spiral filament coils downwards from the spheroid body and is connected to the collopod by a thin plasma bridge that breaks away upon colloblast ejection. The root and root tendrils anchor the colloblast to the denser, inner-layer of the tentacle or tentillum.
MATERIALS AND METHODS
Twenty species of ctenophores were collected from the epi- to bathypelagic zones off the coasts of central California and the Hawaiian Islands. These locations are advantageous because of their relatively near-shore access to very deep environments (Haddock et al. 2017). Shallow species were hand-collected in glass jars by blue-water divers (Haddock and Heine 2005). Meso- and bathypelagic species were collected using suction samplers and detritus samplers on remotely operated vehicles from the Monterey Bay Aquarium Research Institute (Robison 1993). When found extended in the feeding position, tentacles were sucked into a Pasteur pipette and separated from the animal. Tentacles retracted into the tentacular bulb were dissected from intact animals using fine metal forceps and surgical scissors. Tentacles were then prepared by primary fixation at sea using 4% borax-buffered formalin in filtered seawater. In the lab, tentacles were washed with 2.5% NaHCO3 for 30-45 min, and secondary fixation was carried out using 2% OsO4 in 1.25% NaHCO3 for 2-3 h depending on the size of the sample. After secondary fixation, tentacles were rinsed with deionized water until the solution ran clear. Tentacles were dehydrated through an ethanol gra dient using Reichert capsules, critical-point dried in acetone-carbon dioxide using a SAMDRI-PVT-3D, and then sputter coated with gold-palladium using a HUMMER 6.2. Micrographs were taken on a JEOL JSM-6480LV scanning electron microscope and morphometrics of the 3 main ultrastructural components were analyzed using ImageJ (Schneider et al. 2012). If needed, the micrograph’s contrast and brightness were adjusted using linear transformations in Adobe Photoshop.
Collosphere diameter was measured at 2 perpendicular lengths (inset in Fig. 1), one from a superior view (X) and one from a profile view (Y); all collosphere area measurements were two-dimensional and included the external secretion granules. Collosphere classification was determined by the collosphere diameter ratio. The numerator of this ratio was the superior view measurement (Fig. 1 inset a), and the denominator was the profile view measurement (Fig. 1 inset b). Collospheres with a diameter ratio of ~1.0 were considered spheroid; a ratio of ~1.3, ellipsoid; and ratios of ~0.5 or between ~1.1 and 1.2, non-uniform. External secretion granule diameter was measured at 2 perpendicular lengths, and the two-dimensional area was measured only on granules that did not appear “deflated” or “popped”. The spiral filament cross section was measured at multiple points perpendicular to the central axis of the filament. Cross sections of tentacles, tentilla, and colloblasts were observed on specimens that were prepared using the dry-fracturing technique (Toda et al. 1989).
RESULTS
Collosphere and external secretion granule measurements were successfully made on all 20 species; however, spiral filament morphometrics were only recorded for 13 species due to no visible extended colloblasts, or unsuccessful dry-fracturing (Table 1). Three variations of collosphere form, spherical (Fig. 2), ellipsoidal (Fig. 3), and non-uniform (Fig. 4), were found. Colloblasts were observed to either have or not have a thin cap cell membrane covering the collospheres, including the external secretion granules. External secretion granules were deposited by the cap cell in either a single cluster covering the surface of the collosphere or in 1 of 3 patterned granule deposition forms.
Table 1 Morphometrics of colloblasts in ctenophores.
Species | Collection location | Collection ID | Spiral filament cross section (μm) | External granule area (μm2) | External granule diametera (μm) | External granule diameterb (μm) | Secretion granule deposition form | Collosphere area (μm2) | Collosphere diameterX (μm) | Collosphere diameterY (μm) | Collosphere classification |
Aulacoctenidae Aulacoctena sp. 1 | California | D1046-D4 | 2.2 ± 0.2 | 1.0 ± 0.1 | 1.0 ± 0.1 | 1.1 ± 0.1 | Patterned | 116.0 ± 15.0 | 11.0 ± 1.7 | 8.6 ± 1.6 | Ellipsoid |
(Fig. 3a, b; 7c) | |||||||||||
Aulacoctenidae | |||||||||||
Aulacoctena sp. 2 | California | D1168-D3 | 1.8 ± 0.1 | 0.8 ± 0.1 | 0.9 ± 0.1 | 0.9 ± 0.1 | Patterned | 80.0 ± 8.1 | 11.0 ± 0.8 | 8.5 ± 0.5 | Ellipsoid |
(Fig. 3c, 7d) | |||||||||||
Bathyctenidae | |||||||||||
Bathyctena chuni | Hawaii | KM18-T08-3 | 0.4 ± 0.03 | 1.0 ± 0.2 | 1.1 ± 0.1 | 1.1 ± 0.1 | Clustered | 44.0 ± 5.5 | 7.1 ± 0.4 | 7.5 ± 0.2 | Spheroid |
(Fig. 2a) | |||||||||||
Mertensiidae | |||||||||||
Callianira sp. | Hawaii | KM-M123-MS3 | Not visible | 0.6 ± 0.1 | 0.8 ± 0.1 | 0.8 ± 0.1 | Clustered | 13.0 ± 2.2 | 4.1 ± 0.5 | 4.0 ± 0.4 | Spheroid |
(Fig. 2b) | |||||||||||
Undescribed Family | |||||||||||
Cydippid sp. C | California | D1162-D2 | 0.9 ± 0.1 | 0.6 ± 0.1 | 0.8 ± 0.1 | 0.8 ± 0.1 | Clustered | 16.0 ± 5.8 | 3.0 ± 0.5 | 5.8 ± 1.4 | Non-uniform |
(Fig. 4e, 5, 6a) | |||||||||||
Pleurobrachiidae | |||||||||||
Hormiphora palmata | Hawaii | KM18-BW2-5 | Not visible | 0.4 ± 0.1 | 0.7 ± 0.1 | 0.7 ± 0.1 | Clustered | 10.0 ± 1.4 | 3.5 ± 0.3 | 3.4 ± 0.3 | Spheroid |
(Fig. 2c, 6b) | |||||||||||
Pleurobrachiidae | |||||||||||
Pleurobrachia bachei | California | 24Mar19-BW2- | 0.5 ± 0.06 | 0.6 ± 0.1 | 0.8 ± 0.1 | 0.8 ± 0.1 | Patterned | 28.0 ± 2.5 | 6.1 ± 0.2 | 5.7 ± 0.3 | Spheroid |
(Fig. 2d, e; 7a) | 36, 37, 38 | ||||||||||
Undescribed Family | |||||||||||
Cydippid sp. RLL | California | D1022-D4 | Not visible | 0.9 ± 0.2 | 1.0 ± 0.1 | 1.0 ± 0.1 | Clustered | 20.0 ± 3.4 | 3.3 ± 0.1 | 6.4 ± 0.7 | Non-uniform |
(Fig. 5f) | |||||||||||
Undescribed Family | |||||||||||
Cydippid sp. RC | California | D1168-D7 | Not visible | 0.9 ± 0.1 | 1.0 ± 0.1 | 1.0 ± 0.1 | Clustered | 87.0 ± 14 | 11.0 ± 0.7 | 10.0 ± 1.6 | Spheroid |
(Fig. 2f) | |||||||||||
Undescribed Family | |||||||||||
Cydippid sp. RH | Hawaii | KM18-M120- | 0.9 ± 0.1 | 0.5 ± 0.1 | 0.8 ± 0.1 | 0.8 ± 0.1 | Clustered | 83.0 ± 10 | 9.8 ± 0.9 | 9.6 ± 1.2 | Spheroid |
(Fig. 2g) | MS4 | ||||||||||
Undescribed Family | |||||||||||
Cydippid sp. M | Hawaii | KM18-M121- | 1.3 ± 0.2 | 2.1 ± 0.3 | 1.5 ± 0.2 | 1.5 ± 0.2 | Clustered | 90.0 ± 9.9 | 10.2 ± 0.8 | 10.0 ± 0.7 | Spheroid |
(Fig. 2h) | MS4 | ||||||||||
Tjalfiellidae Platyctene sp. P (Fig. 2i) | California | D1165-D10 | 1.0 ± 0.1 | 1.3 ± 0.2 | 1.2 ± 0.1 | 1.2 ± 0.1 | Clustered | 52.0 ± 5.7 | 9.1 ± 0.4 | 8.4 ± 0.1 | Spheroid |
Tjalfiellidae Platyctene sp. W (Fig. 2j) | California | D1165-BT1 | 0.4 ± 0.1 | 0.8 ± 0.2 | 1.0 ± 0.1 | 0.9 ± 0.1 | Clustered | 16.0 ± 2.7 | 4.4 ± 0.3 | 4.5 ± 0.4 | Spheroid |
Lampoctenidae Lobate sp. V (Fig. 4c, 6c, 7f) | California | D1045-D4 | Not visible | 0.8 ± 0.1 | 0.9 ± 0.1 | 0.9 ± 0.1 | Patterned | 50.0 ± 9.0 | 5.1 ± 0.7 | 11.0 ± 1.5 | Non-uniform |
Undescribed Family Cydippid sp. B (Fig. 2k) | California | D1019-D1, D6 | 0.9 ± 0.1 | 1.1 ± 0.3 | 1.1 ± 0.1 | 1.1 ± 0.2 | Clustered | 63.0 ± 6.5 | 8.6 ± 0.6 | 8.4 ± 0.6 | Spheroid |
Euplokamididae Euplokamis sp. (Fig. 2l, 7b) | California | D1169-D4 | 0.5 ± 0.04 | 1.5 ± 0.2 | 1.3 ± 0.1 | 13.0 ± 0.1 | Patterned | 65.0 ± 4.1 | 8.9 ± 0.5 | 9.2 ± 0.6 | Spheroid |
Cestidae Cestum veneris (Fig. 4a) | Hawaii | KM18-BW8-21 | Not visible | 0.7 ± 0.1 | 0.9 ± 0.1 | 0.9 ± 0.1 | Clustered | 38.0 ± 5.1 | 7.0 ± 1.3 | 6.2 ± 0.9 | Non-uniform |
Leucotheidae Leucothea pulchra (Fig. 4b) | Monterey Bay Aquarium | 28Apr19-TT1, 2 | Not visible | 1.5 ± 0.2 | 1.3 ± 0.1 | 1.3 ± 0.1 | Clustered | 22.0 ± 2.8 | 5.3 ± 0.5 | 4.4 ± 0.6 | Non-uniform |
Mertensiidae Mertensiid sp. Y (Fig. 2m) | California | D1137-D3 | 0.7 ± 0.1 | 0.7 ± 0.1 | 0.9 ± 0.1 | 0.9 ± 0.1 | Clustered | 45.0 ± 5.0 | 7.4 ± 0.3 | 7.2 ± 0.4 | Spheroid |
Undescribed Family Cydippid sp. RG (Fig. 2n, 6e) | California | D1165-D7, D9 | 1.7 ± 0.2 | 0.9 ± 0.2 | 1.1 ± 0.1 | 1.0 ± 0.1 | Patterned | 92.0 ± 9.0 | 10.0 ± 1.0 | 10.0 ± 0.3 | Spheroid |

Figure 2 Species with spherical collospheres. (a) Bathyctena chuni. (b) Callianira sp. (c) Hormiphora palmata. (d) Pleurobrachia bachei. (e) Cross section of P. bachei tentillum showing the double-layered tentacle morphology. (f) Cydippid sp. RC. (g) Cross section of Cydippid sp. RH colloblast with nucleus, spheroid body, and radii visible. (h) Cross section of Cydippid sp. M colloblast with a majority of organelles visible. (i) Platyctene sp. P. (j) Platyctene sp. W. (k) Cydippid sp. B. (l) Euplokamis sp. (m) Mertensiid sp. Y. (n) Cydippid sp. RG.

Figure 3 Species with ellipsoidal collospheres. (a) Aulacoctena sp. 1. (b) Profile view of Aulacoctena sp. 1 colloblasts showing the spiral filament and root, but lacking the collopod in the middle of the spiral. (c) Aulacoctena sp. 2.

Figure 4 Species with non-uniform collospheres. (a) Wispy oral tentilla of Cestum veneris. (b) Clump of 4 trailing tentacles from Leucothea pulchra. (c) Oral tentilla from a mature Lobate sp. V. (d) Oblong colloblasts from Lobate sp. V. (e) Indistinct colloblasts of Cydippid sp. C. (f) Indistinct, loop-like collospheres found in Cydippid sp. RLL.
Collosphere morphologies and spiral filaments
Of the 20 species examined, 13 had spherical collospheres, 2 had ellipsoidal collospheres, and 5 had collospheres that took other non-uniform shapes (Table 1, Figs. 2-4). Spiral filaments were observed in ctenophores with spherical (Fig. 2) and ellipsoidal (Fig. 3) collospheres. For spherical collospheres, the spiral filament was covered by the collocyte membrane and coiled around the collopod, connected via the plasma bridge (Figs. 1, 2). For ellipsoidal collospheres, the spiral filament was attached to the narrow end of the ellipsoid, and no distinct collopod was observed protruding from the collo sphere as was present in spherical collospheres (Fig. 3). For non-uniform collospheres, spiral filaments were only observed in cydippid species C (henceforth Cydippid sp. C as in Table 1) when the tentacles were draped across the swimming legs of a copepod (Fig. 5). Spiral filaments were seen stretching more than 200 μm from one entangled swimming leg to another (Fig. 5b), compared to the external secretion granule diameter of roughly 0.8 μm (Table 1). Non-uniform collosphere morphometrics were only recorded from a superior view due to the lack of the traditional colloblast form observed from a profile view. Collospheres of lobate species V (henceforth Lobate sp. V as in Table 1) were elongated and anchored to the oral tentilla along the collosphere’s longer axis (Fig. 4c, d). No spiral filaments were observed in Lobate sp. V.
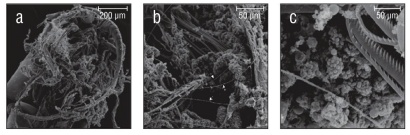
Figure 5 Tentacles of Cydippid sp. C entangled in the swimming legs of a copepod. (a) Underside of copepod with swimming legs visible. (b) Zoomed view of the tentilla. Spiral filaments (arrows) visible stretching roughly 200 μm across the swimming legs of the copepod. (c) Colloblasts near the point of contact between the tentilla and the swimming legs of the copepod.
Cap cell membrane
The cap cell membrane, a thin membrane of cells that contain the external secretion granules awaiting deposition onto the collosphere, was observed in Hormiphora palmata, Cydippid sp. C, and Lobate sp. V (Fig. 6). In Cydippid sp. C, the cap cell membrane was very smooth and tightly enveloped the surface of the collosphere (Fig. 6a). The areas that lacked the cap cell membrane had indistinct collospheres with external granule deposition similar to those seen in H. palmata. In H. palmata, the cap cell membrane had folds throughout its surface (Fig. 6b). On areas of the tentacles of H. palmata where this membrane was absent, granules were deflated and less prominent than those found in other species lacking the cap cell membrane (Fig. 2c), suggesting it may have been torn free and the colloblast had adhered. In Lobate sp. V the cap cell membrane does not completely cover areas of the oral tentilla, and collospheres and external secretion granules can be seen protruding through the cap cell membrane (Fig. 6c).

Figure 6 Cap cell membranes in the senescent stage. (a) Smooth cap cell membrane with external secretion granules visible beneath the surface found in Cydippid sp. C. (b) Hormiphora palmata cap cell membrane visible as folds atop the collospheres. (c) Oblong colloblasts of Lobate sp. V can be seen protruding from the degenerating cap cell membrane.
External secretion granule deposition forms
Of the 20 species examined, 14 species had a continuous cluster of external secretion granules covering the collosphere, and 6 species had 1 of 3 patterned deposition forms (Table 1, Fig. 7). Those with clustered granule deposition forms had little to no space separating the external secretion granules. Those with patterned deposition forms had external secretion granules that were deposited in separated, sometimes geometric, patterns. Aulacoctena sp. 1 (Figs. 3a, b; 7c), Aulacoctena sp. 2 (Figs. 3c, 7d), and Cydippid sp. RG (Fig. 2n) all have external secretion granule deposition patterns resembling a truncated icosahedron, that is, a three-dimensional shape consisting of 12 regular pentagons set edge to edge with 20 regular hexagons to complete the solid. Pleurobrachia bachei and an undescribed Euplokamis sp. have external secretion granules that were deposited in a harlequin pattern, with the external secretion granules located at the vertices of the diamond shapes comprising the pattern (Fig. 7a, b). Lobate sp. V has patterned granule deposition, but the pattern is not as apparent as it is in other species (Fig. 7f). External secretion granules were separated and creases were observed leading from one granule to another across the collocyte membrane (Fig. 7f). Both species of undescribed Tjalfiellidae (benthic platyctenes) have external secretion granules that are clustered and were observed to detach from the collosphere, with the internal secretion granules seen protruding from beneath (Fig. 2i, j). The detachment of external secretion granules was not observed in any of the other species in this study.

Figure 7 Geometric external secretion granule deposition patterns found in 3 families and an undescribed cydippid. Patterns are overlaid in yellow. (a) Harlequin deposition pattern found in Pleurobrachia bachei. The same deposition pattern is found in (b) Euplokamis sp. (c) Truncated icosahedron-like deposition pattern found in Aulacoctena sp. 1. Popped and deflated external secretion granules can be seen throughout the surface of the collosphere. The same deposition pattern and popping or deflation of granules is present in (d) Aulacoctena sp. 2 and (e) Cydippid sp. RG. (f) The granule deposition pattern of Lobate sp. V is separated, but not as geometrically uniform. Creases are visible on the surface of the collocyte membrane.
DISCUSSION
The term colloblast is somewhat of a misnomer, because it implies that the structure is composed of only one cell. However, the colloblast is actually formed from multiple cells: the collocyte, which forms the main body of the colloblast, along with numerous cap cells that produce external secretion granules (reviewed by von Byern et al. 2010). Cap cells form a membrane that covers the developing colloblasts on the tentacles. This unique structure separates ctenophores from all other known organisms (see Dunn et al. 2015). Interestingly, at least 2 genera of tentaculate ctenophores have apparently lost the ability to produce colloblasts. These are Haeckelia (Carré and Carré 1980, Mills and Miller 1984) and the curious monotypic genus Ctenella (Carré and Carré 1993a).
General collosphere morphology
Prior to this study, collospheres had been described as hemispherical across the phylum. Observed herein, a majority of collosphere shapes are closer to resembling a sphere rather than a hemisphere. Two other collosphere forms, ellipsoid and non-uniform, were also observed to exist in a few families. In families with these 2 forms of collospheres, colloblasts lack a traditional collopod. Since the collopod is the location of the elongated nucleus and serves as an anchor point for the resting spiral filament, this finding piques interest surrounding the formation and function of such variations during collosphere morphogenesis.
Spiral filaments
The majority of spiral filament observations in this study agree with those reported in previous studies, which have shown that spiral filaments in resting colloblasts are arranged in a helical coil and maintain this helical form in ejected colloblasts, albeit as a looser coil. It has also been observed that the diameter of the spiral filament cross section does not vary from its resting state and ejected state. A previous study using transmission electron microscopy (TEM) found spiral filaments to be present and coiled once or twice in Cestum veneris and 6 to 7 times in Leucothea multicornis (Franc 1978). This is not consistent with spiral filament observations of C. veneris and Leucothea pulchra in the current study. The lack of spiral filaments for these 2 species could hint at potential variation in spiral filament form due to other factors, such as feeding behaviors. More research into the presence and function of spiral filaments is necessary to understand colloblast morphology as a whole.
Cap cell membrane
Collosphere morphogenesis has 3 stages (Storch and Lehnert-Moritz 1974, Mackie et al. 1988, von Byern et al. 2010). The electron-dense external secretion granules covering the collospheres are not derived from the collospheres themselves but instead are deposited by separate cells referred to synonymously as cap cells, accessory cells, covering cells, or support cells (Benwitz 1978, Mackie et al. 1988, Eeckhaut et al. 1997, Babonis et al. 2018). These cap cells form a membrane that covers the outside of the tentacles. During stage three of colloblast development, the cap cell membrane senesces and degenerates, revealing the external secretion granules on the active collospheres beneath. The micrographs herein taken of H. palmata, Cydippid sp. C, and Lobate sp. V show this membrane imaged via SEM. In this study, the absence of the cap cell membrane in almost all micrographs is likely due to the mature stage of tentacle development at the time of collection and not a characteristic of that species throughout development.
External secretion granules
Benwitz (1978) was the first scientist to deduce that external secretion granules are produced by cap cells instead of the collocyte. This observation was later confirmed by Mackie et al. (1988). Patterns of external secretion granule deposition are not possible to visualize clearly using TEM. In the present SEM study, several patterns were observed. The external secretion granule deposition form resembling a harlequin pattern found in P. bachei and an undescribed Euplokamis sp. shows that similar granule patterns can exist across families. This stands true even when referring to the truncated icosahedron-like external secretion granule depo sition pattern that is found in the genus Aulacoctena and the undescribed family containing Cydippid sp. RG.
The patterned granule deposition forms of P. bachei, Euplokamis sp., both Aulacoctena species, Cydippid sp. RG, and Lobate sp. V demonstrate that external secretion granules are anchored to the collosphere in the areas between the bulges of the internal secretion granules. Mech anisms of external granule attachment by the cap cells are unknown but are likely a factor of the geometric patterns present during metazoan epithelial morphogenesis (Gibson et al. 2006).
The contents of the external secretion granules are unknown. Most authors contend that an adhesive is contained within these granules; however, in the present study no material was observed when these granules were broken open. The copepod that we observed was clearly entangled with spiral filaments, indicating they play an important role in prey capture. No sign of adhesive material was observed on the copepod itself, the filaments, or on the surface of the collosphere. Research into ctenophore tentacles has suggested that the colloblast adhesive contains a DOPA-like catecholic compound (Townsend and Sweeney 2019), and molecular studies indicate the colloblast adhesive is unique to the phylum Ctenophora (Babonis et al. 2018).
Colloblasts in mature Lobata
Ctenophores in the order Lobata have tentacles in the larval stage, and these tentacles possess colloblasts (Borisenko and Ereskovsky 2013). Little is known about the senescence of colloblasts on the feeding tentacles during the transition from larval stage to maturity, when elongated feeding tentacles are succeeded by a fringe of oral tentacles. Micrographs (Figs. 4c, d; 6c; 7f) of the oral tentacles found on mature Lobate sp. V revealed that colloblasts are present despite the loss of traditional prey-capturing tentacles. The absence of spiral filaments in the colloblasts on the oral tentilla in this species implies that spiral filaments are not needed to secure prey during the short transition time from capture by oral tentilla to ingestion.
CONCLUSIONS
Although a majority of the species in our study possess spheroid colloblasts with a singular cluster of external secretion granules covering the collosphere, differences in colloblast ultrastructure can be used to examine functional diversity in the phylum Ctenophora. Ctenophores have specialized and diversified to consume a wide variety of prey, and there are both generalists and specialists (Haddock 2007, Choy et al. 2017). Our current understanding of adaptations to capture specific prey, however, is based mainly on organism-scale morphology and behavior. The potential to add ultrastructural information could greatly enhance our ability to detect co-evolution of predation strategies and prey characteristics. This is particularly promising in the species that do not adhere to the simple paradigm of casting a sticky net to catch crustaceans. The diets of species without side branches on their tentacles appear to be either specialized (narcomedusae for Haeckelia, larvaceans for Dryodora) or unknown (Aulacoctena and some deep benthic species). Colloblasts possessed by the genus Aulacoctena stand out due to their ellipsoidal collosphere and truncated icosahe dron-like external secretion granule pattern. Despite lacking tentilla, the tentacles of Aulacoctena species are particularly sticky (personal observations). Although we surveyed a broad swath of ctenophore diversity, it would still be of interest to examine Dryodora and other Euplokamis. Future work could involve searching genomes and transcriptomes for more colloblast-associated genes (e.g., Babonis et al. 2018), potentially unlocking a novel class of adhesive proteins. Many species of ctenophores are still undescribed, and it is important to document these differences among species to better understand the “hidden biology” (Dunn et al. 2015) of nonbilaterians as a whole. Observations of ctenophore colloblasts will be useful characteristics when describing new species of ctenophores. These morphometric analyses are being analyzed alongside genetic analyses to provide a more thorough understanding of the functional diversity of ctenophores.