Introduction
Bivalve mollusks, specially mussels, are frequently used in environmental pollution monitoring (Kimbrough et al. 2008). As sessile and filter feeding organisms, they bioaccumulate compounds present in the environment and provide a time-integrated measure of pollution at a given site (Sericano 2000). For a bivalve mollusk to be used as a biological monitor “a correlation [must exist] between the pollutant content of the organism and the average pollutant concentration in the surrounding habitat” (Sericano 2000). In the case of organic contaminants, direct relationships are usually observed between bioaccumulation in mussels and environmental concentrations in both water and sediments (Baumard et al. 1999, Beiras et al. 2003b, Solaun et al. 2015). Such relationships have also been reported for metals in some cases (Yap et al. 2002, Beiras et al. 2003a), but no relationship has been observed in others (Gundacker 1999, Beiras et al. 2003a, Giarratano et al. 2010).
Several factors are implied in the final metal concentrations observed in mussels, including physicochemical (uptake route, chemical speciation of metals in both the dissolved and particulate phases) and biological (filtration rate, ability to regulate internal concentrations, physiological state) ones. These factors contribute to the complexity of the prediction of metal bioaccumulation at a given site and can account for the lack of correlation between metals in organisms and metals in environmental compartments. To account for all or most of these factors and processes, mechanistic biodynamic models have been developed. These models integrate the uptake of dissolved metals via the gills, the assimilation of metals from ingested particles, and the excretion of metals into a single equation that predicts the concentrations of metals in the organism at the steady state (Griscom et al. 2002, Luoma and Rainbow 2005). Biodynamic models have proven successful in the forecast of metal bioaccumulation in bivalve mollusks, but what happens with forecasting in the opposite direction? Are bivalve mollusks useful indicators of the risk metals pose to the ecosystem?
According to the free ion activity model (Campbell 1995) and the biotic ligand model (Di Toro et al. 2001), the free ion is the most reactive fraction of a metal, and this is the fraction that should be controlled given the risk its toxicity poses on the most sensitive organisms, such as invertebrate larvae. It would therefore be very useful to find a biomonitor, sessile and widespread like mussels, that can reflect only the bioavailability of dissolved metals, since metal concentrations in mussels are the result of the integration of both dissolved and particulate metal uptake routes. We hypothesize that mussel gills can be used for this purpose. Given that gills are the main exchange surface for dissolved metals, they are expected to reflect the bioavailability of dissolved metals and to respond rapidly to changes in dissolved metal bioavailability, in comparison to the whole organism, which is more influenced by the digestive pathway, the stage in the gametogenic cycle, metal detoxification mechanisms, and other physiological processes. The present study analyzed metal concentrations in gills and whole soft tissues of Mytilus galloprovincialis mussels sampled from sites showing different degrees of metal pollution, to find relationships with concentrations in the environment, including sediment, water, and particulate matter, and determine the performance of these tissues as metal biomonitors.
Materials and methods
Sampling
Sampling was performed during an investigative monitoring of marine pollution in Ría de Vigo (Galicia, NW Spain), which included 4 sampling campaigns, in March and October 2004, March 2005, and April 2006 (Beiras et al. 2012). Sampling was performed at 7 sites (Fig. 1). Five of these sites ranged from clean to moderately polluted and were located from the outermost (BA) to the innermost (RE) part of the ria, 3 of which were under the influence of the Cangas (CA), Moaña (MO), and Vigo (AG) urban areas. The other 2 sites (ViB and ViN) were located in the industrial Vigo Harbor and can be considered heavily impacted sites. AG, MO, and RE were revisited during the 4 sampling campaigns, CA and ViB were sampled during the last 3 campaigns, and BA and ViN were visited only once, so that the data set comprised 20 data points.
Sampling of mussels, water, and sediment was performed on the same day for each sampling campaign. Mussels (M. galloprovincialis) between 40 and 55 mm in size were collected from the lower intertidal area by hand. Water and sediment samples were collected from the R/V Navaz (Spanish Oceanographic Institution) using Go-Flo bottles and a box corer, respectively. Water was sampled at 2 depths, at 1 m beneath the surface (subsurface sample) and at 1 m above the bottom (bottom sample). When water column depth was ≤6 m, only the subsurface sample was taken. All water samples were filtered onboard using 0.45-µm polyethersulfone (PES) filters by connecting a flow-through filtering system to the Go-Flo bottle and applying N2 pressure. Filters were collected in triplicate after filtration of 1 L of water to analyze metals in total particulate matter (TPM). Three more liters were filtered through 3 pre-weighted filters to gravimetrically determine the amount of TPM. Filtered water was collected in high-density polyethylene (HDPE) bottles.
Sample treatment and chemical analyses
Sediments were sieved through a 2-mm mesh prior to chemical analyses. For metal analyses, sediments (triplicate samples) were digested in microwave with a mixture of HNO3-HCl-HF (Merck Suprapur, Germany). Cu and Zn were analyzed by flame atomic absorption spectrometry using a Perkin Elmer AAnalyst 800 (USA), and Cd and Pb were analyzed by electrothermal atomic absorption spectroscopy.
For the analysis of whole mussels, the soft tissues of at least 50 specimens were pooled into a composite sample, homogenized, and freeze-dried. Samples were digested in microwave with HNO3, and metal analysis was performed following the same techniques used for the sediments. The gills of 8 individuals were excised, and Cd, Cu, Pb, and Zn were analyzed separately for each individual using inductively coupled plasma mass spectrometry (ICP-MS) (Thermo Elemental; Cheshire, UK) after microwave-assisted digestion of samples with a mixture of HNO3 and H2O2.
Filters for TPM determination were washed immediately after filtration with 30 mL of 0.5 M ammonium formate and dried at 90 ºC for 48 h. Filters for analysis of metals in TPM were digested with a mixture of HNO3 and H2O2, and metals in the acid extract were analyzed by ICP-MS.
Water samples were acidified to pH 2 with HNO3 and subjected to UV-digestion of organic matter. Dissolved metals (Cd, Cu, Pb, and Zn) were measured by anodic stripping voltammetry using a polarographic stand (Metrohm 663 VA; Herisau, Switzerland) coupled to an Eco-Chemie AutoLab PGSTAT10 pontentiostat. More details about the analytical procedures are given in Beiras et al. (2012).
All labware in contact with samples were previously soaked in 10% HNO3 for 24 h and rinsed with ultrapure water. PES filters were rinsed with 0.2% HNO3 followed by ultrapure water. HDPE bottles for water samples were additionally rinsed with approximately 100 mL of filtered water from the sampling site prior to sample collection.
The quality of chemical analyses was subject to a continuous quality assurance system, including the use of duplicate samples, procedural blanks, and certified reference materials (CRM-278 and CRM-414 from the Boureau Communautaire de Reference, EU, for mussel tissues and particulate matter; BCSS-1 and BEST-1 from the National Research Council of Canada for sediments; and CRM 403 from the Belgium Community Bureau of Reference for seawater). Adequate analytical performance was also assured by regular participation in international intercomparison exercises, such as Quality Assurance of Information in Marine Environmental Monitoring in Europe (QUASIMEME 2005), with satisfactory Z-scores for each metal reported (Besada et al. 2008).
Data treatment
Pearson correlation coefficients were used to evaluate the correlation between variables. Despite the majority of variables not being normally distributed (Kolmogorov-Smirnov normality test), logarithmic transformation was not considered necessary because the relationship between untransformed variables did not depart from linearity (Sokal and Rohlf 1995). Multiple regression analysis was performed using IBM SPSS Statistics v24. Partition coefficients were calculated for particulate metals as the ratio between particulate metal concentration (µg·L-1) and dissolved metal concentration (µg·L-1).
Results
Metal concentrations in environmental compartments
Sediments in harbor sites were clearly enriched with metals with respect to the other sites (Table 1). This is especially remarkable for Cu and Zn, for which no overlapping existed between the concentrations found in non-harbor and harbor sites. The variation ratio (highest to lowest concentration) for all sampled sediments was 40 for Cu, 16 for Pb, 29 for Zn, and 18 for Cd. Metal concentrations in TPM (µg·g-1 dry weight [dw]) were of the same order of magnitude of those observed in sediments; however, at the sites with the most contaminated sediments, metal concentrations in suspended particles were generally lower than those in sediment particles.
Table 1 Range of metal concentrations measured in whole mussel soft tissues (MMussels) and in abiotic environmental compartments (sediment [MSed], suspended particles [MTPM], and water column [dissolved (MDis) and particulate matter (MPart)]) at the sampling sites during the study period. Sites are grouped according to their location, outside (non-harbor sites) or inside (harbor sites) Vigo Harbor. Codes for sampling sites are described in the main text. Dry weight (dw); not applicable (n.a.).
Non-harbor sites | Harbor sites | |||||||
BA | CA | MO | AG | RE | ViB | ViN | ||
MMussels (µg·g-1 dw) | ||||||||
Cd | 0.53 | 0.66-0.73 | 0.57-0.77 | 0.50-0.77 | 0.55-0.61 | 0.57-1.2 | 1.0 | |
Cu | 7.5 | 4.5-6.6 | 5.4-7.6 | 5.9-9.3 | 4.1-9.9 | 33-109 | 59 | |
Pb | 2.0 | 2.0-2.5 | 2.1-3.0 | 3.7-4.5 | 4.0-4.7 | 3.9-15 | 7.5 | |
Zn | 217 | 266-393 | 213-271 | 257-270 | 230-290 | 342-369 | 411 | |
MSed (µg·g-1 dw) | ||||||||
Cd | 0.15 | 0.08-0.17 | 0.20-0.44 | 0.46-0.52 | 0.53-0.70 | 0.70-1.4 | 0.64 | |
Cu | 9.3 | 29-37 | 44-49 | 70-83 | 51-55 | 199-363 | 367 | |
Pb | 18.5 | 67-78 | 45-110 | 63-139 | 112-185 | 117-217 | 305 | |
Zn | 53 | 127-180 | 144-177 | 216-264 | 157-190 | 510-1530 | 299 | |
MTPM (µg·g-1 dw) | ||||||||
Cd | n.a. | 0.17-0.62 | 0.12-0.40 | 0.18-1.3 | 0.24-0.42 | 0.17-0.63 | 0.19 | |
Cu | n.a. | 17-93 | 29-83 | 36-145 | 29-85 | 32-518 | 69 | |
Pb | n.a. | 19-78 | 29-70 | 32-148 | 51-180 | 29-102 | 56 | |
Zn | n.a. | 38-555 | 50-580 | 91-543 | 94-192 | 133-556 | 166 | |
MPart (µg·L-1)a | ||||||||
Cd | n.a. | 0.40-1.4 | 0.27-0.61 | 0.45-3.1 | 0.55-0.93 | 0.33-0.69 | 0.49 | |
Cu | n.a. | 0.04-0.15 | 0.06-0.27 | 0.09-1.1 | 0.08-0.13 | 0.07-0.58 | 0.18 | |
Pb | n.a. | 0.05-0.14 | 0.06-0.17 | 0.08-1.1 | 0.16-0.24 | 0.06-0.19 | 0.15 | |
Zn | n.a. | 0.09-0.97 | 0.10-1.1 | 0.16-1.8 | 0.25-0.37 | 0.29-1.4 | 0.43 | |
MDis (µg·L-1) | ||||||||
Cd | <0.01b | <0.01b | <0.01b-0.5 | <0.01b | <0.01b | <0.01b | 0.07 | |
Cu | 0.17-0.46 | 0.27-1.6 | 0.22-1.0 | 0.32-1.3 | 0.37-1.2 | 0.51-17 | 2.9 | |
Pb | 0.10-0.19 | 0.16-0.6 | 0.1-0.4 | 0.09-0.49 | 0.11-1.6 | 0.11-0.93 | 0.14 | |
Zn | 0.77-2.4 | 0.74-4.1 | 0.79-4.5 | 1.2-3.7 | 0.4-20 | 1.6-48 | 6.5 |
aExcept Cd concentrations, which are expressed in ng·L-1.
bDetection limit for dissolved Cd.
Regarding the water column, Cu and Zn concentrations were lower in the particulate phase than in the dissolved phase, with partition coefficients of 0.21 ± 0.17 (n = 31) for Cu and 0.27 ± 0.29 (n = 30) for Zn. Compared to other metals, Pb showed a higher tendency to be on the particulate phase, with higher partition coefficients (0.68 ± 0.61, n = 32).
Cu concentrations in sediments correlated with dissolved Cu concentrations in surface water samples (r = 0.83, P < 0.001), with particulate Cu concentrations in surface water samples (r = 0.77, P < 0.001), and with Cu concentrations in surface TPM (r = 0.68, P = 0.003). For the other metals, the only correlations observed were between metal concentrations in surface and bottom TPM. In general, the results showed a high degree of variation and different patterns regarding metals in sediment, water, and particulate matter, so the 3 compartments may have different relationships with metal bioaccumulation in filter feeding organisms.
Metal concentrations in whole soft tissues and gills
Regarding whole mussel soft tissues, Cd concentrations were quite homogeneous throughout the sites, being only slightly higher at ViB and ViN (Table 1). Cu concentrations ranged from 4 to 10 µg·g-1 dw at all stations located outside harbors, while at the 2 sites in Vigo Harbor Cu concentrations (33-109 µg·g-1 dw) were about one order of magnitude higher than at the other sites. Pb concentrations were higher at the harbor sites (4-15 µg·g-1 dw) and at the inner stations (RE and AG, ~4 µg·g-1 dw) than at the other locations, where Pb concentrations were between 2 and 3 µg·g-1 dw. The highest Zn concentrations were also observed in whole soft tissues of mussels from the harbor sites, followed by CA, although the difference with the least contaminated sites was not very high (~1.5 times) (Table 1).
In general, metal concentrations in gills were within the same order of magnitude as those found in whole soft tissues, although deviations from the 1:1 line were observed for all metals except Pb (Fig. 2). Pb concentrations were very similar for gills and whole soft tissues along the pollution gradient. In the case of Cu, the deviation from the 1:1 line was not very high in low to moderately polluted places, while at harbor sites, Cu concentrations were up to 5 times higher in gills than in whole soft tissues. It is remarkable that Zn in mussel gills was virtually constant around 60 µg·g-1 dw along a Zn gradient in whole mussel soft tissue (from 200 to 400 µg·g-1 dw), except for a single sample point (ViB-1004), for which Zn concentrations in gills reached the same concentration observed in whole mussel soft tissues. A similar pattern was observed for Cd, showing lower values in gills (around 0.2 µg·g-1 dw) than in whole soft tissues (0.5-1.2 µg·g-1 dw), with only 1 site (ViB-1004) showing higher Cd concentrations in gills. Significant correlations were observed between metal concentrations in gills and those in whole mussel soft tissues for Cu (r = 0.936, P < 0.001) and Pb (r = 0.889, P < 0.001), but not for Zn and Cd.
Comparison of metal bioaccumulation with environmental compartments
No significant correlations were found between bioaccumulated Cd and Cd concentrations in any of the abiotic environmental compartments analyzed. Cu concentrations in whole mussel soft tissues correlated with Cu concentrations in sediments, with Cu concentrations in TPM collected from both surface and bottom waters, and with dissolved Cu in bottom waters (Table 2). Interestingly, ViB in the October 2004 survey (ViB-1004) showed the highest Cu concentration in mussels, especially in mussel gills, but it was not the sampling point with the highest Cu concentration in sediments (Fig. 3). However, it was the sampling point with the highest dissolved Cu concentration in bottom waters (Fig. 3) and in TPM in bottom waters (518 µg·g-1, followed by 163 µg·g-1 in ViB-0305). It seems that some process occurred at that point during this survey that provoked an increase in Cu concentrations in the water column that was in turn reflected in mussel gills and, to a lesser extent, in whole mussel soft tissues. If this point is excluded from the correlation analysis, Cu concentrations in whole mussel soft tissue no longer correlate with dissolved Cu concentrations in bottom waters and Cu concentrations in TPM, although the correlation with Cu concentrations in sediments is maintained (Table 2). Apparently, at up to 100 µg·g-1 of Cu in sediments, mussels were able to maintain Cu concentrations at stable levels, below 10 µg·g-1 dw (Fig. 3). However, at harbor sites, where Cu concentrations in sediments were markedly higher, Cu bioaccumulation increased, probably due to a loss in the regulation ability of mussels to maintain constant body Cu concentrations at these high levels, as will be discussed later. If the data points corresponding to harbor sites (Cu concentrations in sediments greater than 100 µg·g-1) are excluded from the analysis, Cu concentrations in whole mussel soft tissues no longer correlate with Cu concentrations in sediments, although concentrations in mussel gills still do (Table 2).
Table 2 Pearson correlation coefficients between metal concentrations in tissues and metal concentrations in environmental compartments*. The significance of the correlation is given by the P value in italics, and sample number is given in brackets. Significant correlations are highlighted in bold.
CuSed | CuTPMsur | CuTPMbott | CuParsur | CuParbott | CuDissur | CuDisbott | ||||||||||||
CuWhole mussels | 0.668 | 0.942 | 0.370 | 0.664 | 0.466 | -0.363 | 0.903 | 0.285 | 0.489 | 0.373 | 0.373 | 0.418 | 0.945 | 0.483 | ||||
0.001 | <0.001 | 0.158 | 0.005 | 0.080 | 0.246 | <0.001 | 0.395 | 0.182 | 0.154 | 0.233 | 0.067 | <0.001 | 0.080 | |||||
(20) | (19)a | (16)b | (16) | (15)a | (12)b | (12) | (11)a | (9)b | (16) | (12) | (20) | (15) | (14)a | |||||
CuMussel gills | 0.413 | 0.974 | 0.788 | 0.639 | 0.866 | 0.106 | 0.932 | 0.262 | 0.778 | 0.223 | 0.396 | 0.071 | 0.991 | 0.494 | ||||
0.079 | <0.001 | <0.001 | 0.010 | <0.001 | 0.743 | <0.001 | 0.436 | 0.014 | 0.425 | 0.202 | 0.773 | <0.001 | 0.073 | |||||
(19) | (18)a | (16)b | (15) | (14)a | (12)b | (12) | (11)a | (9)b | (15) | (12) | (19) | (15) | (14)a | |||||
PbSed | PbTPMsur | PbTPMbott | PbParsur | PbParbott | PbDissur | PbDisbott | ||||||||||||
PbWhole mussels | 0.372 | 0.839 | 0.310 | 0.270 | 0.091 | 0.014 | 0.218 | 0.903 | -0.307 | |||||||||
0.107 | <0.001 | 0.242 | 0.396 | 0.738 | 0.965 | 0.357 | <0.001 | 0.286 | ||||||||||
(20) | (19)a | (16) | (12) | (16) | (12) | (20) | (15) | (14)a | ||||||||||
PbMussel gills | 0.149 | 0.710 | 0.304 | 0.170 | -0.030 | -0.073 | 0.219 | 0.960 | 0.059 | |||||||||
0.543 | 0.001 | 0.270 | 0.597 | 0.915 | 0.822 | 0.368 | <0.001 | 0.840 | ||||||||||
(19) | (18)a | (15) | (12) | (15) | (12) | (19) | (15) | (14)a | ||||||||||
ZnSed | ZnTPMsur | ZnTPMbott | ZnParsur | ZnParbott | ZnDissur | ZnDisbott | ||||||||||||
ZnWhole mussels | 0.457 | 0.441 | 0.070 | -0.252 | 0.040 | -0.414 | 0.013 | 0.445 | 0.192 | |||||||||
0.043 | 0.059 | 0.797 | 0.429 | 0.883 | 0.181 | 0.959 | 0.097 | 0.510 | ||||||||||
(20) | (19)a | (16) | (12) | (16) | (12) | (18) | (15) | (14)a | ||||||||||
ZnMussel gills | 0.174 | 0.578 | 0.259 | 0.194 | -0.049 | -0.101 | -0.084 | 0.995 | 0.201 | |||||||||
0.477 | 0.012 | 0.352 | 0.546 | 0.862 | 0.754 | 0.749 | <0.001 | 0.490 | ||||||||||
(19) | (18)a | (15) | (12) | (15) | (12) | (17) | (15) | (14)a |
*Metal concentration in whole soft tissue (Whole mussel), mussel gills, sediments (Sed), total particulate matter (TPM), TPM in surface water (TPMsur), TPM in bottom water (TPMbott), particulate concentration in surface water (Parsur), particulate concentration in bottom water (Parbott), dissolved concentration in surface water (Dissur), dissolved concentration in bottom water (Disbott).
aOne data point (ViB in the October 2004 sampling) was excluded from the analysis.
bAll data points corresponding to harbor sites were excluded from the analysis.
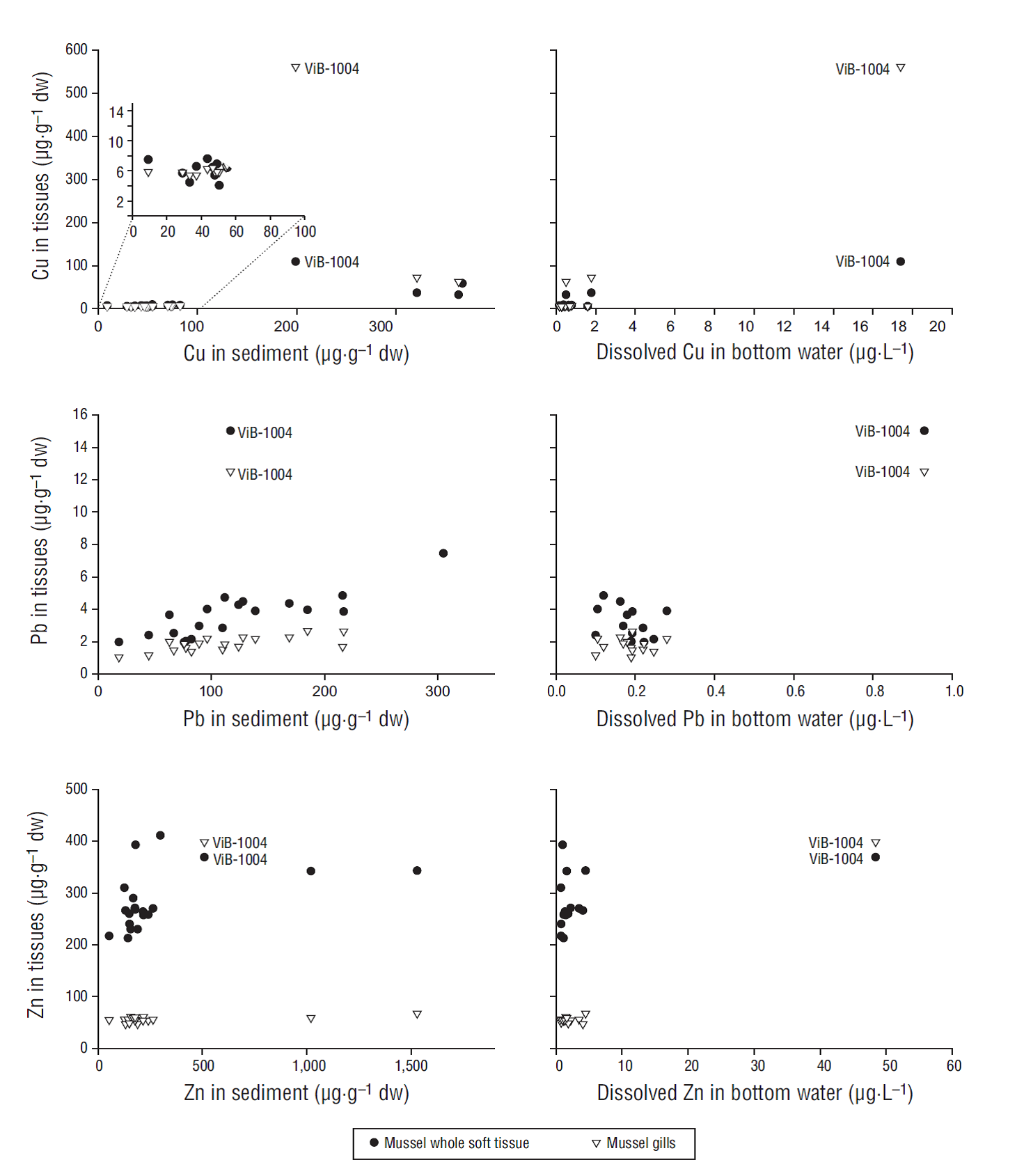
Figure 3 Relationships between metal concentrations in tissues (whole soft mussel tissues and mussel gills) and environmental concentrations in sediment (left panel) and dissolved metal in bottom waters (right panel). The ViB-1004 sampling point is identified
In the case of Pb, there was no correlation between Pb in tissues and Pb in sediments, because of the same outstanding point, ViB-1004, which showed the highest Pb concentration in mussel tissues but an intermediate concentration in sediments (Fig. 3, Table 2). If this point is excluded from the analysis, a significant correlation is observed between bioaccumulated Pb (both in gills and in whole soft tissues) and Pb in sediments. On the contrary, if Pb concentration in tissues is compared to Pb concentration in bottom waters, a significant correlation is observed due to the data associated with ViB-1004, but if this point is excluded, the correlation is lost (Table 2). A high Pb concentration was observed at this station and sampling time both in mussels and in bottom waters (dissolved), but this was not reflected in sediment concentrations, which were more stable and did not show high fluctuations with the time of sampling (Fig. 3).
For Zn, a significant but weak correlation (r = 0.46, P = 0.043, n = 20) was found between Zn in whole soft tissues and Zn in sediments when all data were included. On the contrary, the correlation for Zn in mussel gills was not significant when all points were included, but when the data associated with ViB-1004 were excluded, a significant correlation was observed between Zn in mussel gills and Zn in sediments (r = 0.58, P = 0.012, n = 18). This was because the Zn concentration in gills was about one order of magnitude higher for this sampling point compared to all others, but ViB-1004 was not the sampling point reflecting the highest concentration in sediments. Zn concentrations in bottom waters were in agreement with the pattern observed for Zn in mussel gills, with Zn concentrations about one order of magnitude higher at ViB-1004 than those at the other points (Fig. 3). A significant correlation was found between Zn in mussel gills and Zn dissolved in bottom waters (r = 0.99, P < 0.001, n = 15), due to the same sampling point (Table 2).
Multiple regressions were done with all data to analyze if metal bioaccumulation could be better modeled using a combination of variables. Cu in whole soft tissues and Cu in mussel gills were well predicted using both dissolved Cu in bottom waters and Cu in sediments (Table 3). The same occurred for Pb in whole soft tissues, which was well modeled using dissolved Pb in bottom waters and Pb in sediments. On the contrary, modeling of Pb in mussel gills did not improve by using 2 variables, dissolved Pb in bottom waters being the best predictor alone. In the case of Zn, Zn in whole soft tissues was best predicted by Zn concentrations in sediments, and Zn in mussel gills was best predicted by dissolved Zn concentrations in bottom waters (Table 2); no significant improvement was achieved with the use of an additional variable.
Table 3 Significant multiple regressions of metal bioaccumulation in mussel tissues with metal concentrations in environmental compartments including all sampling pointsa. Dissolved concentration in bottom water (Disbott), sediments (Sed).
Model | r2 | P value (change in r2)b |
CuWhole mussels = -0.08n.s. (±0.96) + 5.29*** (±0.17) CuDisbott + 0.083*** (±0.007) CuSed | 0.992 | P < 0.001 |
CuMussel gills = -18** (±5) + 31.4*** (±0.83) CuDisbott + 0.15*** (±0.03) CuSed | 0.993 | P = 0.001 |
PbWhole mussels = -0.8n.s. (±0.8) + 14.4*** (±1.7) PbDisbott + 0.013* (±0.006) PbSed | 0.869 | P = 0.047 |
aThe significance of regression coefficients is given by n.s. (not significant), * (0.05 ≥ P > 0.01), ** (0.01 ≥ P > 0.001), and *** (P ≤ 0.001).
bThe P value is given for the observed change in r2 when changing the regression from simple to multiple.
Discussion
Mussels can regulate their internal Cu concentrations within a certain limit (Amiard et al. 1987, Lorenzo et al. 2003, Lorenzo et al. 2005), but at very high Cu concentrations in the environment, this regulation capacity is lost. Because of this, correlations between Cu concentrations in mussels and Cu concentrations in environmental compartments such as sediments are usually difficult to find (Langston and Spence 1995, Hummel et al. 1997, Przytarska et al. 2010) unless very high levels of Cu pollution are reached (Beiras et al. 2003b), as observed in the present study. This has raised questions about the usefulness of mussels in Cu pollution monitoring, and the use of other filter-feeding species such as oysters has been proposed, given that oysters apparently lack any regulatory ability for Cu (Cooper et al. 1982, Lorenzo et al. 2003). Mussel gills seem to be a suitable complement for Cu biomonitoring because they seem to respond more quickly to environmental changes in Cu concentrations than do the rest of the mussel tissues (Sánchez-Marín et al. 2016). This is well exemplified by the data from the ViB-1004 sampling point, where an increase in dissolved Cu of about an order of magnitude compared to the other sampling points was directly reflected by a one-order-of-magnitude increase of Cu in gills, but in the whole body this increase was much smaller (Fig. 3). Also, at low to moderately polluted sites, with less than 100 µg·g-1 of Cu in sediments, gills performed better than whole mussel soft tissue in reflecting environmental variability in Cu concentrations. Cu in mussel gills correlated with Cu in sediments even when harbor locations were excluded from the analysis (Table 2), while whole mussel soft tissues at all sites outside harbors showed similar Cu concentrations.
In the case of Pb, both gills and whole organisms showed very similar relationships with abiotic environmental compartments, and their Pb concentrations were very similar, closely following a 1:1 relationship even at the most polluted sites (Fig. 2). Laboratory experiments showed a rapid distribution of Pb within mussel tissues. In a study on dissolved Pb uptake kinetics, Pb concentrations in gills and rest of tissues were similar in all time points (from 1 to 24 h) (Sánchez-Marín et al. 2011). The high Pb concentration at ViB-1004, both in mussels and in the dissolved phase, reveals that mussels are reflecting a real-and probably recent-increase in the concentration of bioavailable Pb, even though this is not reflected in the sediments. Sediments integrate metal pollution at larger time scales than mussels do, and they show much more stable concentrations with time. Therefore, it seems that both gills and whole mussel soft tissues can respond to changes in dissolved Pb concentrations, so the use of gills does not represent an advantage over the use of whole mussel soft tissue for biomonitoring this metal.
As in the case of Cu, bioavailability of Zn seems to be better reflected by gills than by whole mussel soft tissue. This is probably also due to the regulation of this essential metal at the whole organism level (Phillips and Yim 1981, Amiard et al. 1987). Zn concentrations ranged from 200 to 400 µg·g-1 dw in whole mussel soft tissue and did not seem to exceed that range even at highly polluted places such as harbor stations. On the contrary, Zn concentrations in the gills were lower and seemed to respond better to increases in the dissolved fraction (e.g., the ViB-1004 sampling point), whereas the concentration in whole mussel soft tissue did not.
Multiple regression analyses revealed that Cu and Pb bioaccumulation could be well predicted on the basis of metal concentrations in sediments and dissolved concentrations in bottom waters (Table 3). Despite particulate matter being an important source of metal to mussels via the digestive pathway, no significant correlations were found between metal concentrations in organisms and metal concentrations in the particulate form for any metal. The only metal showing significant correlations between mussels and TPM concentrations was Cu (Table 2), although this correlation was mainly due to a single sampling point, ViB-1004, showing much higher Cu in TPM than the other points. A study conducted by Gagnon et al. (2006) using the freshwater mussel Elliptio complanata showed a distinct bioaccumulation pattern for the gills and digestive glands of transplanted mussels. Their hypothesis, which was similar to ours, pointed towards the use of mussel gills as bioindicators of dissolved metal bioavailability, and they also explored the use of digestive glands as bioindicators of particulate metal bioavailability. However, contrary to what was expected, Gagnon et al. (2006) found negative correlations between metal concentrations in gills and metal concentrations in the dissolved fraction, and no correlations between metals in the digestive gland and metals in the particulate form. This manifests the difficulty in finding consistent trends in these kinds of studies, given that many factors usually play a role in metal bioavailability and bioaccumulation, including biological factors such as mussel condition, stage of reproductive development, or ability to regulate body concentrations of some metals, and physicochemical factors such as variations in temperature, food availability, or changes in metal speciation. Bourgeault et al. (2011) showed that the amount of particulate metal assimilated by zebra mussels could be affected not only by the concentration of metal in the particulate form but also by metal speciation in particles and mussel filtration rate, which can vary from site to site; moreover, mussels can selectively ingest some particles and avoid others. These factors cause spatial variations in ingestion rates and assimilation efficiency ( Bourgeault et al. 2011).
Temporal variability of metal concentrations in the water column-in both the dissolved and particulate phases-is expected to be very high and vary with tides, currents, and climatological events. Approaches using passive samplers such as diffusive gradients in thin films for dissolved metal and sediment traps for TPM allow obtaining metal concentrations representative of a longer period and would probably better reflect metal bioaccumulation. The analysis of labile fractions of metal in sediments, as opposed to total metal, allows estimations of bioavailable metal concentrations, and in some cases it yields better correlations with metal concentrations in bivalves (Yap et al. 2002, Amiard et al. 2007). However, issues associated with the regulation of essential metal concentrations in the body still mask pollution levels when using bivalve biomonitors.
In summary, the present results indicate that the use of mussel gills in biomonitoring metal pollution is promising because these tissues can record changes in the concentrations of the dissolved forms of bioavailable metals in the environment, especially at high levels of essential metals such as Cu and Zn, which are regulated at the whole body level. To further confirm this assertion, it would be desirable to extend this study to include more sampling points, including bodies of water with different levels of metal pollution. This can be done by including the analysis of mussel gills and of dissolved and particulate metals in investigative monitoring, preferably employing passive sampling devices, in addition to other most commonly sampled environmental matrices.