Introduction
The aim of this paper is to explore patterns of distribution and life histories of small pelagic fishes along the west coast of the Baja California Peninsula (Mexico) in the context of regional circulation of the California Current System (CCS).
We focus on the most important species within the region, namely the Pacific sardine (Sardinops sagax), northern anchovy (Engraulis mordax), Pacific mackerel (Scomber japonicus), and jack mackerel (Trachurus symmetricus). Climate variability over seasonal to interdecadal time scales acts to modify the physicochemical and biological characteristics of the habitat occupied by these species. Their mobility allows them to move their spawning grounds both in time and space and to select favorable areas for spawning to ensure reproductive success to sustain their populations. We review the historical trends of the commercial catches and the variability in the distribution of spawning and abundance of eggs as indicators of movement and productivity of the different stocks. Special interest is given to Pacific sardine and Pacific mackerel since they are subject to active management in the Baja California fisheries and show important variation in catches over the scales of months to several decades, and they have suffered historical population collapses in the 20th century.
Small pelagic fishes are key components in the CCS. They are planktivorous species that exhibit schooling behavior and multiple spawning over an annual cycle, and generally occur in large biomasses, have a wide distribution range, and thus share some life history traits. Together with euphausiids, these species represent more than 10% of the biomass of the CCS and affect the abundance, condition, and behavior of upper trophic levels in the food web (Kaplan et al. 2013). One important characteristic is their sensitivity to environmental change, which makes them vulnerable to variations in physical forcing (Parrish et al. 1981) and has resulted in dramatically fluctuating abundances (Lluch-Belda et al. 1989, Schwartzlose et al. 1999, Alheit et al. 2009). However, their commercial exploitation, which represents roughly 25% of the world's fish catch (Checkley et al. 2009), has also been responsible for historical changes in their productivity (Hsieh et al. 2006, Rykaczewski and Checkley 2008). Because they integrate the productivity of lower trophic levels, their often dramatic changes in population size make small pelagic fish important indicators of the response of the pelagic ecosystem to changes in ocean climate.
A population is vulnerable to the combination of fishing and unfavorable environmental conditions that may act to reduce their reproductive capacity (Hsieh et al. 2006, Anderson et al. 2008). Planktivorous pelagic species experience higher susceptibility to fisheries due to the removal of older fish, resulting in truncated age and size distributions as well as their high fecundities (Anderson et al. 2008). According to Peck et al. (2013), the collapse and replacement of a population begins with a change in abundance due to smaller year-class sizes linked to lower reproductive success. Since the correct evaluation of time and spatial scales of distribution and abundance may be biased due to the operational scale of fishing, independent methods are needed to evaluate the natural state of the populations. The information depends on factors such as longevity of the species and their patterns of movement over the annual cycle, as well as interannual and decadal scale changes.
This paper addresses relevant aspects of the life history and distribution of the small pelagic species living in waters of the Baja California Peninsula. We begin with a description of the physical setting of the CCS, followed by more general aspects of how the life cycle of the species is coupled to ocean climate to determine their patterns of abundance and distributions, and we then describe the concept of stocks and demographic characteristics that distinguish each of these subpopulations. Note that we designate the different sardine stocks with names that reflect their association with the water masses in which they reside, that is, "subarctic" stock and "subtropical" stock rather than "cold" and "temperate", respectively, as used by Félix-Uraga et al. (2004). Sometimes we refer to "temperate subtropical" and "cold subarctic" stocks.
Furthermore, we explore the population dynamics of small pelagics in the waters off northern Baja California in relation to their abundance based on catch statistics provided by the National Fisheries Institute (INAPESCA) for sardine, mackerel, and anchovy (Cota-Villavicencio et al. 2010). In order to evaluate the validity and usefulness of existing paradigms in fisheries oceanography, and to stress the importance of the interaction of ocean climate with fisheries resources, we have included our own interpretations and data regarding sardine dynamics and behavior in Baja California waters. As there is much more information available for sardines than for other small pelagic species, thanks to efforts dating back to the 1930s focusing on the Pacific sardine, we use the example of sardines more frequently to illustrate many of the ideas and interpretations presented with respect to small pelagic fish.
Physical setting
The structure and organization of a wide variety of small pelagic fish species along the CCS are closely coupled to the physics and climate dynamics of the upper 200 m of the water column (Mackas 2006, Baumgartner et al. 2008, Checkley and Barth 2009, Durazo 2009). The seasonal biological production of a wide variety of plankton species is stimulated by the injection of nutrient-rich cold and salty water to the upper layer by coastal upwelling throughout the year (Rykaczewski and Checkley 2008). The result of this forcing is the creation of a nearshore transition region that separates cold upwelled waters found along the coast from less dense, warmer, and less saline water offshore. The coastal area is dominated by offshore Ekman transport and the oceanic region is dominated by geostrophic flow (Lynn and Simpson 1987, Durazo and Baumgartner 2002). Newly upwelled water is usually transported to the ocean interior by cold filaments and mesoscale structures (~80-100 km) that extend 200-400 km (Mackas 2006, Checkley and Barth 2009). In the oceanic zone (~1000 km offshore and from the surface to 300 m), the equatorward flow of the California Current (CC) carries cold, low-salinity water to subtropical and tropical latitudes (Lynn and Simpson 1987).
Along the coast, there are regions where coastal upwell-ing produced by the alongshore winds may be enhanced by wind stress divergence and topographic effects. Wind stress curl promotes upwelling outside the influence of the continental border (Landry et al. 2012). Positive curl within the Southern California Bight is associated with the presence of a cyclonic gyre. The southern edge of this large eddy is known as the Ensenada front centered around 31°N. This gyre separates the north-central California region from the Southern California Bight and the waters off Baja California at Point Conception (34°N) (Fig. 1). The front causes more olig-otrophic ocean water to be entrained towards the coast, which suppresses primary production to the south, resulting in relatively low concentrations of chlorophyll in the coastal area off Ensenada (Baumgartner et al. 2008). Elevation of the thermocline observed around capes because of topographic effects occurs at Punta Baja (30°N) and Punta Eugenia (28°N). Here, enhanced upwelling is produced by the interaction of the mean flow of the CC with both capes (Barth et al. 2000). This region exhibits negative wind stress curl, different from the positive values north and south (Nelson 1977), which would imply clockwise circulation. However, the presence of a quasi-permanent cyclonic circulation and relatively cold waters year-round (Lynn and Simpson 1987, Durazo 2015) suggests that localized upwelling is dominated by the current-cape interaction rather than wind stress curl. Cape-enhanced upwelling upstream provides the necessary conditions for high nutrient supply, while the presence of cyclonic circulation provides the physical mechanism for larval growth and survival. Positive wind stress curl may also be important in the Gulf of Ulloa (~26°N), where the combination of upstream nutrient-rich water supply from Punta Eugenia, the cyclonic circulation associated with wind divergence, and the contribution of southern waters by the transport induced by a poleward coastal flow from summer to winter, secures the required conditions for high productivity. Thus, the spatial and temporal variability of wind stress curl along the peninsula (Durazo 2015) may play a key role in the seasonal pumping of nutrients to the euphotic zone, and may also structure the onshore-offshore gradients of primary and secondary producers off Baja California, as has been suggested by Rykaczewsky and Checkley (2008).

Figure 1: Map showing the location of the sites mentioned in the text: Point Conception (PC), Southern California Bight (SCB), Ensenada (ENS), Punta Baja (PB), Punta Eugenia (PE), Bahía Magadalena (BM), and Cabo San Lucas (CSL).
We use the hypothesis of Logerwell and Smith (2001) to examine the paradigm that "environmentally based recruitment models, when updated with new data, invariably fail" (Leggett and Frank 2008). Logerwell and Smith (2001) propose that eddies could provide a mechanism for offshore expansion of favorable habitat for growth and survival of sardine larvae with a higher concentration of chlorophyll within the eddies. Cyclonic and anticyclonic eddies propagate to the west at ~2 km per day (Kurian et al. 2011). However, despite providing a mechanism for offshore expansion of favorable habitat for early life stages of sardines, Nieto et al. (2014) provide evidence that the offshore advection reduces recruitment success due to the ultimate loss of the reproductive products from the population. Nonetheless, it is reasonable that eddies located near or inshore of the axis of the CC (see fig. 5 in Nieto et al. 2014) would allow larvae and early juveniles to reach the nearshore nurseries and survive to recruit to the adult population. This study by Nieto et al. (2014) contradicts the hypothesis proposed by Logerwell and Smith (2001) and can be used as the basis of an improved hypothesis since their results indicate increased recruitment during warm El Niño years and poor recruitment during cool La Niña years, suggesting that transport of eddies is increased by Ekman dynamics. El Niño conditions impede offshore transport of larvae and early juveniles and may even improve survival by onshore transport or by simply retaining a relatively high density of food in the coastal zone.
Basin-wide scales also modulate the habitat characteristics of the CCS. The changes are mainly associated with the large-scale atmospheric circulation which results in important changes in the oceanic environment. In the eastern North Pacific, the wind field is controlled by two atmospheric pressure centers: the Aleutian low-pressure system, located at approximately 50°N, 170°W, and the central Pacific high-pressure center. This combination is known as the North Pacific Oscillation (NPO, Mackas 2006) centered north of Hawaii. An enhanced Aleutian Low during winter creates strong poleward winds and currents. The NPO intensifies in the spring and summer and is responsible for the northwesterly winds and intensification of the offshore Ekman transport off central California and Baja California (Checkley and Barth 2009). In autumn and winter, the weakening of the high pressure system causes a reduction in the equatorward flow (U.S. GLOBEC 1994). Interannual to decadal changes in the position and magnitude of the NPO modify upwelling-favorable winds, sea surface temperature (SST), and sea level at those scales. Over the oceanic environment, the changes are seen as variations in the relative contributions of the major water masses in the upper 300 m, namely the equator-ward flow of subarctic water and the poleward transport of tropical and subtropical waters.
The Pacific Decadal Oscillation (PDO, Mantua et al. 1997) and the North Pacific Gyre Oscillation (NPGO, Di Lorenzo et al. 2008) are the main modes of variability in the North Pacific Ocean forced by the pressure centers. Fluctuations in these modes have been associated with variations in salinity, nutrients, and chlorophyll, with consequences at all trophic levels (Durazo 2009, Lavaniegos 2009). Di Lorenzo et al. (2013) have suggested that the Aleutian Low controls the PDO and that it is associated with the first mode of SST anomalies obtained for the entire North Pacific. The warm phase of the PDO is associated with the deepening of the Aleutian Low and a weakening in the equa-torward flow of the CC (King et al. 2011). The response to weakened upwelling-favorable winds is the anomalous increase of nearshore sea level and SST that relaxes the ocean pressure gradient towards the coast. The NPGO is the oceanic response to the NPO forcing, identified as the second mode in the sea level anomaly for the central and eastern North Pacific (180-110°W, 25-62°N; Di Lorenzo et al. 2010). The positive phase of the NPGO is associated with the intensification of the NPO, which in turn intensifies northwesterly winds and strengthens the equatorward flow of the CC.
El Niño Southern Oscillation (ENSO) is another ocean-atmosphere phenomenon that influences ocean habitat. There are two types of ENSO, the canonical El Niño and El Niño occurring in the central Pacific (Kug et al. 2009). Di Lorenzo et al. (2013) proposed a conceptual model in which climate variability in the North Pacific over several decades produces a poleward redistribution of heat from tropical latitudes. The warming in the equatorial regions caused by ENSO modifies the zonal and meridional atmospheric circulation (Hadley and Walker cells), which eventually alters rainfall and evaporation global patterns. A redistribution of tropical heat associated with changes in water masses and wind stress fields (Durazo and Baumgartner 2002) may cause a bottom-up effect in the food web of the ecosystem (U.S. GLOBEC 1994, Checkley and Barth 2009, McFarlane et al. 2010).
Life cycle adjustments to variability in ocean conditions
Small pelagic fish have similar adaptations of their life histories that allow them to adjust to seasonal variability in the conditions of the pelagic habitat including the search of food, reproduction, and growth (Blaxter and Hunter 1982). Their migration patterns and schooling behavior used for foraging and protection against predators are perhaps the most important features that allow them to accomplish this (Radovich 1982, PFMC 1998). Relatively large sizes of individuals are found in both Pacific mackerel and jack mackerel, which are presumably adapted to a greater range of movement in their foraging. Their reproduction is oviparous at night, with multiple spawning over the year so that their peak spawning is apparently linked to peaks in food availability (Blaxter and Hunter 1982).
With the exception of T. symmetricus, all small pelagic species belong to intermediate trophic levels (2-3). However, significant seasonal changes in wind stress modify both the habitat characteristic and the composition of food on which small pelagics depend (Table 1). In particular, anchovies can act as filter or particulate feeders. In the latter case, which is the dominant, they have the ability to select copepods and euphausiids (Van der Lingen et al. 2009). Although it is not mentioned by Van der Lingen et al. (2009), we believe that only juvenile anchovies would select copepods, since the size of the prey determines the size of the predator. Trachurus symmetricus is the species with the greatest longevity (>30 years), highest fecundity (1.85 × 106 eggs year-1), and greatest size (~80 cm) of the small pelagics. It feeds on crustaceans, small fish, and pelagic mollusks (Demer et al. 2012). Sardines have a finer branchial basket with more gill rakers and very fine teeth that are likely to improve harvesting of their predominant supply of food in their habitat (Van der Lingen et al. 2009). Because sardines posses a special organ that allows them to process vegetative matter, they are able to feed on both phytoplankton as well as crustaceans (McFarlane et al. 2005, 2010). Their larvae show a preference for copepods with average sizes of ~3 mm (Van der Lingen et al. 2009). The Pacific mackerel is more prey-selective and its diet includes squid and other pelagic mol-lusks as well as smaller fish such as anchovy (CalCOFI 1953, Lo et al. 2010a, Demer et al. 2012; see Table 1 for comparison of the species).
Table 1: Trophic level associated with the habitats occupied by the different life stages of small pelagic fish.

*Transitional areas refer to regions between coastal upwelling and oceanic waters
The use that different species make of selected environments at different life stages (larvae, juveniles, adults) is considered an adaptation to the seasonal variability. The life cycle of anchovy is associated with upwelling coastal areas, mainly with reproductive peaks in winter (Checkley et al. 2009), and their spawning areas may extend from coastal waters to ~300 km offshore (Blaxter and Hunter 1982). The jack mackerel is usually associated with oligotrophic oceanic environments compared to other small pelagic species (PFMC 1998, Anderson et al. 2008, Demer et al. 2012), and has a reproductive peak in June (Macewicz and Hunter 1993). Its center of abundance occurs between 150 and 400 km off the coast (Ahlstrom and Ball 1954). Sardine and Pacific mackerel occupy a transitional zone between the region of coastal upwelling and oceanic waters during spring, with sardine located principally off central California and mackerel off the central region of the Baja California Peninsula (Parrish and MacCall 1978, Nevárez-Martínez et al. 2006, Lo et al. 2010b). Sardine spawning peaks are in spring and summer while Pacific mackerel spawn in spring (April-May), with relatively large egg size in the north and an equatorward size gradient. In contrast to sardines, Pacific mackerel spawning exhibits extremely patchy and dense distributions (Parrish and MacCall 1978, Lo et al. 2010a; see Table 2 for comparison of the species).
Table 2: Stocks of small pelagic fish and their latitudinal preferences associated with hydrographic features in the California Current System. They are defined in relation to human activity and their affinities to different thermohaline characteristics, different reproductive schedules, and unique population parameters.
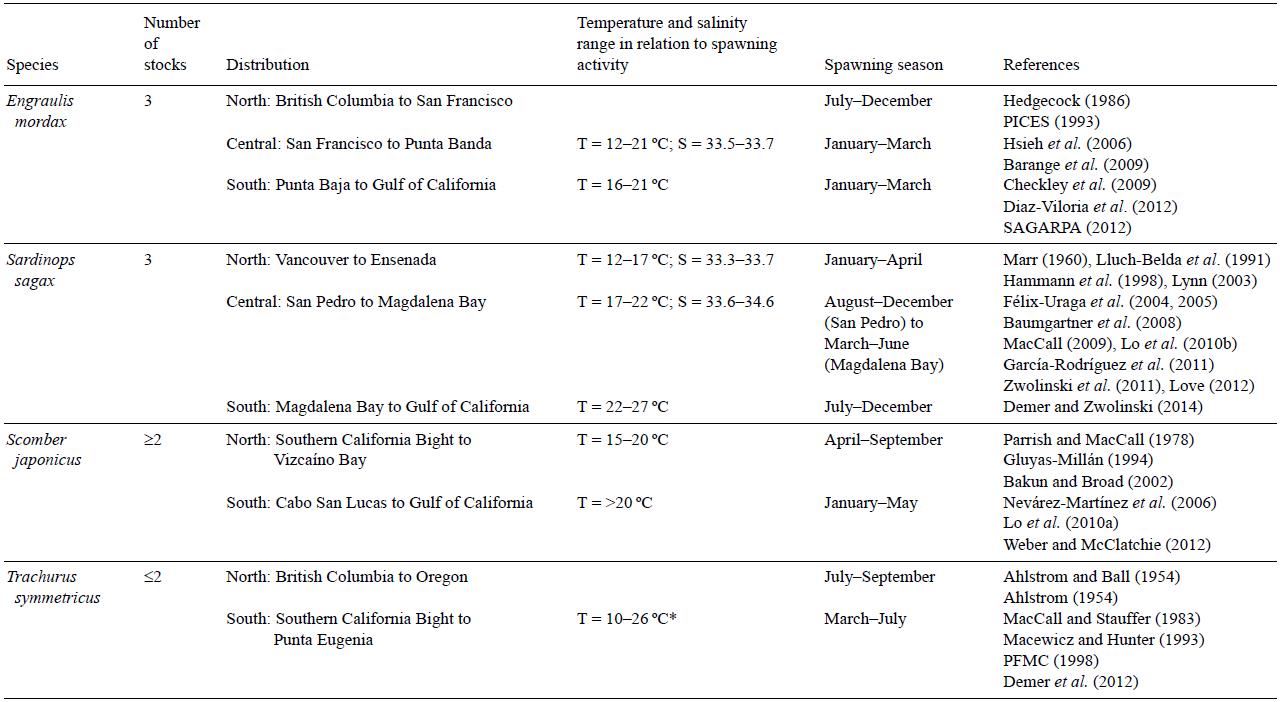
*Preferred spawing temperature is reported for the species, but the range may indicate the presence of stocks.
A reproductive strategy that allows adaptation to the habitat that a particular species occupies is the buoyancy of their eggs, which allows them to remain in the upper 90 m of the water column during their 1.5-4 days hatching time (Ahlstrom 1954). Additionally, the size and shape of eggs are useful characteristics for species identification. The oval eggs of anchovy are thought to be the reason for reducing cannibalism on their eggs (Blaxter and Hunter 1982). According to Moser (1996), sardines have larger and relatively buoyant spherical eggs (1.3-2.1 mm) compared to jack mackerel (0.9-1.1 mm) and Pacific mackerel (0.8-1.3 mm). Seasonal differences in the volume of the egg yolk sac, consisting of proteins, are also important. Vitelline dry weight of winter-spring spawned eggs represents ~38% of the weight, compared to 12-25% of summer eggs (fig. 8 in Blaxter and Hunter 1982). This allows for an extended period of larval survival under starving conditions after occlusion.
Advection of larvae away from regions of low concentrations of food and/or other unfavorable factors improve their odds for survival. Dispersion due to winds and currents may also reduce the chances for survival although their specific attributes such as shape and composition, plus their short incubation period (<5 days) minimize their dispersion from their area of spawning. The selection of spawning area may be an adaptive mechanism to counter or use the variability in environmental conditions, particularly at the longer decadal scale (Ahlstrom 1960), as a species expands its range of distribution. The colonization of new areas allows a population to establish itself under more favorable environmental conditions, which is clearly evident in the expansion and contraction of populations of small pelagic species. This is consistent with the association of potential spawning habitat with favorable environmental condition indicated by Checkley et al. (2000), Lynn (2003), and Van der Lingen et al. (2005). There is also a tendency for phenotypic differences in small pelagics in response to environmental variability, while there is little to no genetic differentiation (Smith 2005).
The physicochemical characteristics of the habitat occupied by small pelagics are mainly controlled by the structure and intensity of the wind field, which modifies the width of the coastal zone and the position of the upwelling front. These conditions may be illustrated by contrasting the conditions observed during 2002 and 2003 in the CCS (Fig. 2). We used climate scenarios obtained with the data-assimilation SODA-POP numerical model developed by Carton et al. (2005), which was accessed on the Texas A&M University website (http://sodaserver.tamu.edu). Nearshore positive (negative) salinity (temperature) anomalies were observed in 2002, consistent with an increased alongshore wind stress during La Niña. This in turn produced increased offshore Ekman transport with lifting of shelf-break subsurface water that caused salinities to rise and temperatures to fall at the surface near the coast. In contrast, winds during 2003 weakened and produced positive temperature anomalies, the relaxation of the coastal sea surface height gradient, and weaker upwelling leading to lower salinities. Conditions observed in 2003 were related to a reduced transport of CC water coincident with a decreased wind stress curl and weak vertical Ekman pumping (Durazo 2009, Durazo et al. 2010), and remained until 2006.

Figure 2: Sea surface wind stress (arrows) circulation patterns during April 2002 and April 2003 as derived by the SODA-POP numerical model. Color contours indicate the corresponding wind stress magnitude anomalies referred to the 1958-2008 wind stress climatology. In 2003, the figure shows a weakening of wind stress (0.04 N m-2) off central California, the main region where sardine spawning occurred. Contour interval is 0.02 N m-2.
Since the early 1990s, the main spawning center for sardines has been located off central California, with latitudinal and onshore-offshore shifts associated with the El Niño and La Niña phenomena (Lynn 2003, Song et al. 2012). During La Niña conditions and a more intense CC in spring 2002, Baumgartner et al. (2008) showed a significant offshore movement of the subarctic stock that lives in the low salinity and cooler subarctic waters. Its spawning ground extended as far south as Punta Eugenia (28°N), a behavior not previously reflected in the conceptual model of Félix-Uraga et al. (2004). In contrast, a weaker CC and a reduced offshore Ekman transport during El Niño 2003 displaced the species towards northern Baja California and central California, and compressed the stock towards the coast resulting in strong recruitment in 2004 in California and the Pacific Northwest (Lo et al. 2010b, Zwolinski and Demer 2013). In 2003, the stability of the water column would likely have been more favorable for the reproductive success of the southern subtropical stock.
Biogeographical changes in relation to the habitat are linked to the concentration and density of plankton during larval and adult stages, producing an increase in sardine egg production during periods of high food availability (Bakun and Broad 2002). When energy gain exceeds movement costs, northern stock sardines begin their migration after they reach 20 cm length and one year of age (Zwolinski and Demer 2012). MacCall (2009) suggests that unlike other species, an important advantage of sardines and anchovies in a fluctuating environment is that they are able to transfer their reproductive energy into the following season, with anchovies moving into estuaries and coastal upwelling areas in the Southern California Bight when their population sizes are reduced, and sardines retreating into the coastal waters off Baja California (Marr 1960, Checkley et al. 2009). When the sardine population shrinks, shoals become mixed with other small pelagic fish like anchovies and mackerel (Bakun and Broad 2002), reducing the effects of predation and thus compensating for the lower population levels (Blaxter and Hunter 1982).
Population changes potentially modify the reproductive potential and are positively associated with age (Hsieh et al. 2006, Anderson et al. 2008). Although changes in ecosystem structure are forced by interannual variability (El Niño/La Niña), the persistence in the atmospheric dynamics of the North Pacific at decadal scales leads to ocean climate regimes with periods of roughly 30 years. Surprisingly, this does not yet appear to have been affected by the global warming associated with the increase in greenhouse gases. An example of this regime is the regional cooling between 1947 and 1976 that changed both sardine and mackerel age structure in southern California fisheries. Between 1916 and 1959, the average age of sardine at all ports was ~3.5 years (Marr 1960, Hsieh et al. 2006). Furthermore, during the collapse of the sardine fishery beginning in the 1940s, the fisheries off California and the Pacific Northwest showed average ages with modes of 2 and 4 years (San Pedro and British Columbia), with a maximum of 8-13 years (Radovich 1982). During 1953, individuals older than 4 years disappeared from the fishery and were not found in the coastal samples of California and Baja California (CalCOFI 1953). Additionally, coastal cruises during the summer and autumn of 1950 to 1952 indicated reproductive failures since 1948 from northern California to Magdalena Bay. The catches off Ensenada in 1952 showed a decrease of ~2.2 times the 1951 captured biomass (21,330 metric tons [t]) and the disappearance of individuals of 2-4 years belonging to the 1946, 1947, and 1948 age classes (CalCOFI 1953). A similar trend was observed in mackerel in which there was a significant reduction of individuals of age 5 to 1 from 1929 to 1970 (Hsieh et al. 2006).
Sedimentary records of sardine scales have also shown interdecadal fluctuations in the abundance of sardines in the CCS since roughly 400 AD. This is likely to reflect changes in recruitment associated with the expansion/contraction of the sardine population (Field et al. 2009). This explains the variations in the sedimentary record of different anoxic basins in the CC, with an expanded population at high densities centered in central California and British Columbia, and contraction in regions around Baja California and the Gulf of California (Parrish and MacCall 1978, Rodríguez-Sánchez et al. 2002). Similar results are observed in mackerel. Fluctuations in abundance (including population collapses) of small pelagic fish appear to be quasi-periodic, with periods centered around ~60 years (Baumgartner et al. 1992).
Spawning regions
Whereas the biological definition of an exploited stock requires sufficient information to characterize the different spawning areas and the timing of reproduction of the different stocks (table 2; MacCall 1986), the operational definition depends mainly on the area of capture by the fishery. It is important to recognize that the distributions are not stable since decadal fluctuations can alter the location of spawning centers over the latitudinal range of the different stocks (Ahlstrom 1960, 1966). Their ranges can shift over periods from interannual to decadal scales as oceanographic conditions change, such that spawning habitats can be differentiated by their thermohaline properties as shown in Figure 3 and Table 2. We define a stock here as a group of individuals from a population under human exploitation, with unique biological parameters such as size at age and differences in fecundity that depend mainly on food availability. This is illustrated by the low fecundity of Pacific sardine in waters off northern Baja California where food availability is limited compared to fish in waters off southern California during 1994 (see fig. 11 in Lo et al. 2005).

Figure 3: Hypothesis of the latitudinal movements of sardine stocks associated with hydrographic features of the spawning habitat during 2000. In the lower plots, the geographical shifts of spawning habitat of the northern (blue dashed line) and southern (red dashed line) stocks are shown. The spawning habitats, defined as subarctic and subtropical habitats, of the two stocks are differentiated by their thermohaline water properties shown at the top of the figure. Colors on the plot indicate temperature-salinity seasonality during egg collections made by the IMECOCAL program in 2000: January (black), April (blue), July (green), and October (red).
The existence of three sardine stocks has been identified in the CCS, from northern California, through the waters off the Baja California Peninsula, and into the Gulf of California (Félix-Uraga et al. 2004, Smith 2005). This is consistent with the patterns of movements of sardines established by Clark (1945) through the use of tagging over the period 1935 -1944. Morphometric data from otoliths associated with capture temperatures (García-Rodríguez et al. 2011) and cohort analysis (Félix-Uraga et al. 2005) provide further evidence of these three sardine stocks. Morphotypes are almost surely the result of environmental factors that modify the phenotypic characteristics of the organism since there is no evidence of genetic separation of the stocks. While the anchovy population is also considered to consist of three stocks, its genetic structure provided by the analysis of allozymes was found to be highly complex and differentiated (Hedgecock 1986). Marine Plio-Pleistocene deposits and fish remains in coastal Indian middens in southern California indicate that the northern anchovy has been present in the CCS for at least ten million years, while the Pacific sardine is a recent arrival having appeared roughly around 15,000 years ago (Fitch 1969). The characteristics of the stocks of small pelagic fish in the CC and the Gulf of California are described in Table 3.
Table 3: Demographic parameters associated with small pelagic fish population showing differences in sizes and trophic levels among species, as well as considerable variation in the ages at sexual maturity, spawing frequency, and batch fecundity.
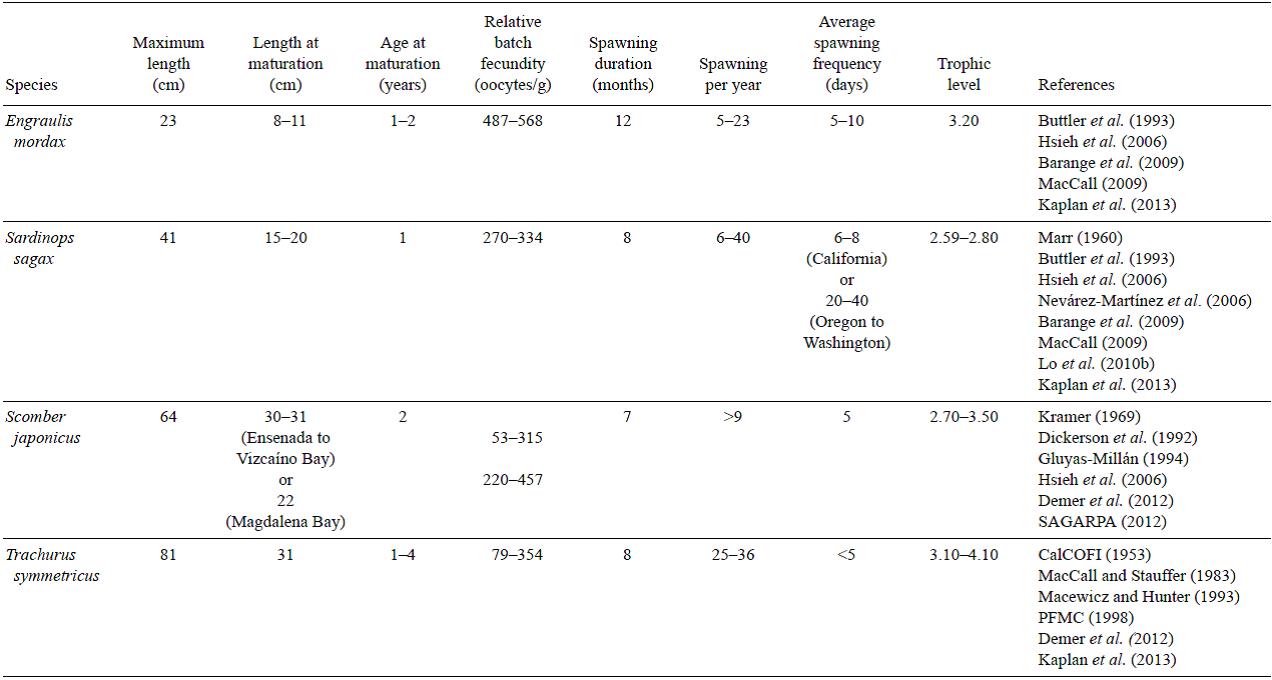
The large-scale expansions and contractions of the habitats of small pelagic populations are associated with large changes in biomass. Changing environmental conditions on interdecadal time scales induce progressive changes in abundance and distribution of anchovy and sardine populations (Rodríguez-Sánchez et al. 2002, Lecomte et al. 2004). These changes not only result in displacement of their centers of biomass but also affect the carrying capacity of their habitat in relation to foraging and reproduction (Barange et al. 2009). In the case where a population is contracting, productivity decreases into what is known as refuges to insure survival. For the anchovy these areas have been the waters in the northern CCS off Oregon. This is contrasted with refuge for the Pacific sardine after the collapse of its population, which began in the late 1940s, that contracted southward into the waters off Baja California where natural mortality and lack of reproductive success reduced the biomass to very low levels (Marr 1960, Rodríguez-Sánchez et al. 2001).
Historical perspective of changes in small pelagic populations
The management of small pelagics along the western coast of the Baja California Peninsula is regulated by Norma Oficial Mexicana (Official Mexican Norm) 003-PESC-1993 (DOF 1993) and the SAGARPA (2012) fishery management plan. These regulations cover technical and operational aspects of the fishery. The management plan, in which the goal is to achieve sustainability of the resources and the mitigation of environmental and fishing impacts, is based on a legal minimum catch size. Productivity of the fishery is currently measured as the amount of biomass captured. However, due to unique demographic characteristics and the differential response to environmental conditions of these species, a fishery-independent management plan is needed to monitor and evaluate each of the stocks found along the peninsula. Legal minimum catch sizes and periods of closures should take into account the status of the population and reproductive peaks in each region.
The waters off the Baja California Peninsula are divided into two fishing units: a northern unit extending from the US-Mexico international border down to Punta Baja and a southern unit running from Punta Eugenia to the area south of Magdalena Bay (Cota-Villavicencio et al. 2010, Torres-Villegas 2012). Catches are landed at the ports of Ensenada in the north and San Carlos in Magdalena Bay in the south. Although the operation of the fishing fleet is restricted to a distance of 70 km from the coast (DOF 1993), it currently operates at a distance of approximately 20 km offshore due to lack of proper refrigeration (Cota-Villavicencio et al. 2010). The four small pelagic species are recorded in the catches in the northern region, while catches in the Magdalena Bay region consist only of sardine and mackerel (Nevárez-Martínez et al. 2006). Over the last decade, sardines and mackerels, which are currently under active management, are mostly frozen for shipping overseas or for national consumption (SAGARPA 2012), with biomass of the landings used as an indicator of productivity of the fishery. Anchovy and jack mackerel (Trachurus) are under passive management, with no upper limit having been established for annual catches.
The history of the operation of the fleet based in Ensenada shows four periods: exploration, substitution, development, and consolidation (Fig. 4; following the curves presented in fig. 7 in Csirke 1985). The exploration phase lasted from 1929 to 1950. Sardine fishing began in Ensenada in 1929 and extended to Cedros Island in the 1940s and to Magdalena Bay in the 1950s motivated by the local market demand (Nevárez-Martínez et al. 2006). By the end of the 1940s sardine catches began to decline. Tagging studies indicated the movement of the northern sardine stock towards northern Baja California and showed that the resource was unregulated and under intense fishing pressure from southern California to British Columbia (Clark 1945). Catches in California ports were gradually declining and northward movement was limited to waters off central California. The change in the northern stock spawning ground towards central Baja California began in 1949 (CalCOFI 1953). By 1952, sardine spawning disappeared north of Point Conception and was found completely displaced towards the region from Punta Baja to the south of Magdalena Bay (Ahlstrom 1960). Every year afterwards ended in a regional reproductive failure due to the absence of sufficient food supply coupled with unregulated fishing and the unfavorable environmental conditions (Marr 1960) until the recovery that began in the 1980s, which was emphatically confirmed by the appearance of sardines in British Columbia waters in 1992 (McFarlane and Beamish 2001).

Figure 4: Time series of Pacific sardine (Sardinops sagax) catches made by the fleet operating off the northern portion of the Baja California Peninsula from the coast to 20 km offshore. The information includes catches off Cedros Island up to 1994, when the fleet ceased operations.
With the collapse of the Pacific sardine in the California fishery, a substitution stage began. This was characterized on the one hand by the exploration of other resources such as anchovy and on the other by the movement of the Mexican fleet to the Gulf of California (Nevárez-Martínez et al. 2006). The catch of other small pelagics became important during the period of sardine collapse, with an increase and expansion of the northern anchovy biomass in 1950-1952, which eventually became the most important fishery off California (CalCOFI 1953). The larval distribution of T. symmetricus indicated a spawning nucleus near the center of the Baja California Peninsula (between Point Conception and Punta Baja), located between 150 and 600 km offshore from March to July (CalCOFI 1953). The catches of jack mackerel showed an increase in size from 20.3 to 38.1 cm in southern California, with predominant ages of 2 years and a maximum of 6. During this period, Pacific mackerel larvae were also found throughout the year, in the same region where jack mackerel were spawning, with peaks in April and May (CalCOFI 1953). The catch of Pacific mackerel in southern California showed a similar behavior to that of sardine, including the collapse of the fishery (Parrish and MacCall 1978). Mackerel, anchovy, and jack mackerel showed a decrease in their catches in California while becoming more important in Baja California (CalCOFI 1953). Anchovy landings in Ensenada acquired more importance due to the collapse of Peruvian anchovy in the early 1970s, and the demand resulted in intensive exploitation off northern Baja California (Nevárez-Martínez et al. 2006), reaching a peak in 1981 with >2.5 × 105 t (see fig. 1 in Cota-Villavicencio et al. 2010).
Compared to other small pelagics, anchovies show a greater sensitivity to environmental effects, which reduce the survival and recruitment of juveniles. With the gradual increase in temperatures in the CCS after 1976, the spawning habitat of anchovies retreated northward. Catches off Baja California (0-1 year individuals) decreased in importance with the movement of their spawning grounds into southern California (MacCall 2009). Butler et al. (1993) suggested that warmer temperatures may have reduced the growth rates of early larval stages after the exhaustion of their yolk reserves, thus reducing the size of the larval population by 74%. During this period the occasional catches of sardine and mackerel increased, giving way to the development stage of their fisheries in Mexico (Cota-Villavicencio et al. 2010).
In the late 1980s, the anchovy fishery off Baja California collapsed at the same time that the spawning area moved northward into southern California. The presence of anchovy was widely distributed off southern California during 1997 and 2005-2008 (Bjorkstedt et al. 2010). However, there were years in which spawning was either confined to coastal areas or virtually absent. There has been significant spawning of northern anchovy off Baja California at least since 2006. Data from the Mexican Program for California Current Research (IMECOCAL, for its Spanish acronym) indicated important spawning off Baja California by T. symmetricus particularly in the winter of 2001, when there was no spawning off California. Jack mackerel spawning areas are widely distributed in the oceanic waters off Baja California, showing a meridional migration pattern during spring/summer and fall/winter. Their presence in the CalCOFI region has been limited to relatively low densities with distribution overlapping areas of sardine spawning during warm years off both southern California and northern Baja California (Baumgartner et al. 2008, Bjorkstedt et al. 2010).
Félix-Uraga et al. (1996) proposed that the significant recruitment of sardines after 1984 (>1.5 x 108 individuals) in the Magdalena Bay fishery was a result of a large increase in abundance as indicated by the 16-fold increase in landings during the sardine recovery in the period 1984-1994. Older individuals (>5 years) were observed in the Magdalena Bay catches in southern Baja California in 1983, 1988, and 1992, leading to strong recruitment in the following years (Félix-Uraga et al. 1996, 2004). The year of increased catches in Ensenada was 1989 (8566 t), favored by a shift to the north in the distribution of the subtropical stock (17-20 °C during the fall) with a subsequent decrease in landings in Magdalena Bay during the subsequent years, from 1989 to 1993 (Félix-Uraga et al. 1996). The trend in the Magdalena Bay recruitment remained high through 2004, when the recruitment and the catches began a downward trend until 2010 (SAGARPA 2012). Availability of the cold subarctic stock off Ensenada was reduced in the early 1990s due to its displacement northward into southern California (Félix-Uraga et al. 2004). This result reveals two elements needed to understand the population structure and movements of the sardines: (1) the information needed to discriminate between the two stocks from sardine landings in Ensenada prior to 1990 is limited since the fishing was focused on anchovies rather than sardines, and (2) upon recovery of the sardine population, underway by the late 1980s, both the subarctic and subtropical stocks had begun seasonal northward movements along the coast with the subarctic stock reaching as far as British Columbia (MacFarlane and Beamish 2001, Lo et al. 2010b).
Off Ensenada, the consolidation stage of the fishery began in the early 1990s and continued into 2013. Sardine landings have remained above a threshold of 22,500 t (1994), with peaks in 1997 and 2011 of 68,000 and 70,000 t, respectively (Félix-Uraga et al. 1996, Zwolinski and Demer 2013). The catch of Pacific mackerel also increased in this period and eventually reached a maximum of ~51,000 t in 1998 (Nevárez-Martínez et al. 2006, Cota-Villavicencio et al. 2010). Lo et al. (1996) suggest that the increase in the sardine spawning area during spring favored the biomass of the northern subarctic stock, with spawning patterns associated with filaments of cold water (13-14.5 °C) in California (including the area around Monterey Bay and Piedras Blancas) and, to a lesser extent, off Baja California, south to Vizcaíno Bay.
The regional monitoring conducted with underway temperature and salinity sensors, coupled with the collection of near-surface fish eggs using a continuous underway fish egg sampler (CUFES, Checkley et al. 2000), indicate the thermo-haline ranges and the selection of the reproductive habitat by the adults. Given the relatively weak currents of the CC (<30 cm s-1) and the resulting limited horizontal transport of fish eggs (<26 km d-1), the spawning areas clearly indicate the presence of adults. Here, we hypothesize a synchronous latitudinal movement of the spawning habitat of the northern and southern stocks of Pacific sardine off Baja California defined by temperature and salinity (Fig. 3). This idea is consistent with the spatial and temporal pattern of the movement of these stocks proposed in the conceptual model of Félix-Uraga et al. (2004) and corroborated by the predictive model of the potential habitat of sardine (Demer and Zwolinski 2014). The southward movement of the northern stock is associated with the equatorward transport of the cooler subarctic water down to Punta Eugenia during the cold La Niña conditions in winter and spring, while the southern stock dominates the catch in this region in summer and fall (Baumgartner et al. 2008, Checkley et al. 2009). However the availability of the cold subarctic stock is reduced because of the limited offshore operation by the fleet (Cota-Villavicencio et al. 2010).
Based on histological observations of sardine gonads from the commercial catch, the northern stock spawns during winter and spring (SAGARPA 2012). Peak egg production occurs in April, although INAPESCA states that it may occur during March or February in some years. However, because sardine catches are limited to 20 km from the coast, these estimates may be strongly biased since sardines move offshore to spawn during spring to avoid the recently upwelled, colder water nearshore. Baumgartner et al. (2008) showed the distribution and relative concentration of eggs in CUFES samples occurring outside the upwelling fronts in spring, parallel to the coast between 50 and 200 km offshore. The information of the spawning habitat inferred by the presence of eggs indicated a high probability of finding reproductive adults of sardines that were not available to the fishing fleet.
Discussion
We have presented a review of the life cycle, distribution, and relationship to ocean climate conditions of four species of small pelagic fishes along the west coast of the Baja California Peninsula. This region is highly heterogeneous in terms of hydrography, bathymetry, and productivity, and has a wide range of habitats that vary over space (latitudinal and onshore/offshore) and time (from seasonal and interannual to interdecadal scales) for pelagic species along the coast. Habitats are modified by alongshore winds, by wind stress curl, and by the interactions between currents and capes, particularly the prominent cape formed by Punta Eugenia that forms the southern boundary of Vizcaíno Bay in central Baja California. Forcing by alongshore equatorward winds produces upwelling along the coast that provides the nearshore habitat preferred by anchovies. Offshore of the upwelling area, a frontal zone offers the necessary food supply for sardine and Pacific mackerel that prefer similar habitat conditions. The oligotrophic region away from the coast, driven mainly by wind stress curl, is occupied by the larger size and highly mobile jack mackerel. Habitat selection by these different species provides a natural system of organization for their separation into units that are managed as fishery stocks. Although the current management plan calls for monitoring of biomass for the actively managed stocks, information is not yet available in regard to efforts to determine the biomass of sardine or Pacific mackerel off the Baja California Peninsula. However, this information should be forthcoming in the near future with the new 62-m fisheries research vessel recently put into service that is now equipped with hydroacoustic instruments, fishing gear, and CUFES. A fundamental criterion is still the minimum size requirement for individual fish. The overall objective of the management plan is to insure continuity of the fisheries resources by establishing levels of acceptable exploitation to permit sustainable yields of species under active management.
One of the paradigms in fisheries oceanography is "Spawning stock biomass (SSB) is a suitable proxy for reproductive potential of a stock" (Leggett and Frank 2008). The reproductive potential of a fishery stock is often judged by the number of recruits to the adult population. Total egg production based on estimated rates of egg mortality and temperature-based time to hatching can be used as a proxy for reproductive potential that is generally correlated to spawning biomass, although the daily egg production method (Lasker 1985) used to estimate the spawning biomass requires knowledge of adult parameters that include batch fecundity, mean weight, and proportion of females and their frequency of spawning. Even so, the relationship between spawning biomass and egg production does not provide a direct estimate of productivity leading to recruitment. Recruitment is also subject to unfavorable (or more favorable) environmental conditions such as offshore (or onshore) transport of eggs and larvae, which may result in loss (or improved survival) of eggs and larvae, as well as availability (or lack) of food for newly hatched larvae and favorable (or unfavorable) conditions for the survival of juveniles (pre-recruits). Leggett and Frank (2008) cited Yaragina and Marshall (2000) to note that indeed "a search for a comprehensive understanding of the factors regulating variability in reproductive potential, independent of SSB, may be as complex and as inextricably linked to environmental and ecological factors, as are the causes in egg and larval survival."
The Ensenada sardine landings (port of El Sauzal) from March to June 2002 included juveniles (pre-recruits) less than 15 cm long (which is smaller than the legal size) less than 1 year old, and <50 g weight (Fig. 5), and suggested a pattern of recruitment that depends on the previous year's spawning (February-July 2001). There is a significant contrast in size and weight between the first and second semesters of the year. There is a significant contrast in size and weight between the first and second semesters of the year. The dominance of the subtropical sardine stock in the catch resulted in an abrupt shift to a larger size in July (shown in Fig. 5) and their condition improved in July and August relative to the first semester catch dominated by the subarctic stock (shown in Fig. 6). The reduction in size of the sardines in the August through November samples seen in Figure 5 is likely to have been produced by the fishery, as argued by Hsieh et al. (2006).

Figure 5: Length-weight relationship for monthly samples of Pacific sardine (Sardinops sagax) landings at the port of El Sauzal, just north of Ensenada, from January through November 2002. The heavy dashed red line separates the age groups of 0 and 1 year-old fish in the April sample. The length scales run from 100 to 200 mm for each sample.
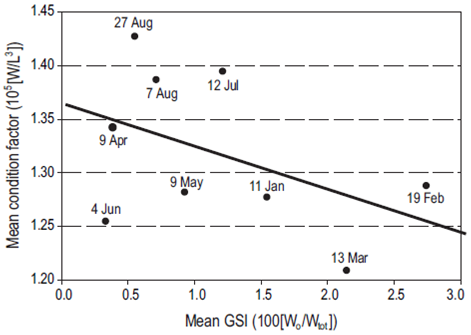
Figure 6: Mean condition factor (W = total weight, L3 = [fork length]3) vs mean gonosomatic index (GSI; Wo = weight of ovary; Wtot = total weight).
Landings of the Ensenada fishing fleet suggest that the availability of sardines depends on the latitudinal movements of the stocks in the CCS. Figure 7 shows that the long-term monthly means of catches lag SST by one to two months, indicating that the seasonal modification of habitat conditions induces synchronous migration of the subarctic and subtropical stocks in agreement with the conceptual model of movement of stocks presented by Félix-Uraga et al. (2004). This could be a response of the biomass of both stocks following the latitudinal movement of their characteristic habitats. In summer and fall, the southern stock moves as far north as San Pedro, California, and is compressed into the coast by the seasonal warming. In winter, a portion of the cold northern stock moves southward into northern and central Baja California and then moves offshore to spawn during spring to avoid the cold upwelled water along the coast, resulting in a much lower catch during April, May, and June, as shown in Figure 5 for 2002. It is also noteworthy that 0-aged fish composed roughly one half of the landings in this period (Fig. 5). This suggests that the inshore area likely acts as a refuge from predation and as a source of higher food concentration for the young fish.

Figure 7: Seasonal pattern of Pacific sardine (Sardinops sagax) catches in northern Baja California waters (1990-2005, black line), obtained from the monthly catch data provided by the Fishing Subdelegation in Ensenada (Baja California, Mexico). Monthly averages of sea surface temperature (SST, blue line) ~80 km off the coast near 31ºN. The SST seasonal cycle was computed using 1997-2004 data obtained from NOAA's Pacific Fisheries Environmental Laboratory Live Access Server.
Figure 7 shows that the reduced landings of the subarctic stock are associated with minimum temperatures during spring and that the elevated landings of the subtropical stock are associated with higher temperatures in late summer and fall. The ratio of the catches of subarctic stock in the first semester (January-June) to the catches of subtropical stock in the second semester (July-December) indicates that the availability of the subtropical stock to the Ensenada fleet is reduced in cold years, whereas the availability of the subarctic stock is reduced during warm years, as demonstrated by Figure 8 for the years between 1980 and 2005. Demer and Zwolinski (2014) reported that the southern subtropical stock represented 60-70% (from 36,000 to 42,000 t) of the catch landed at Ensenada in the months from May to November of the years 2006 to 2011. In our opinion there is a small bias in their estimates since this period overlaps the months in which the northern stock is present off Ensenada (May and June, according to our Fig. 7).

Figure 8: Interannual change in sardine catch rates in temperatecold waters off Ensenada from 1980 to 2005. The red regression line shows the progressive increase in the contribution of the temperate southern stock (July-December). Colder years are shown below the regression line and in relation to the ideal rate (heavy dashed line). The thin dashed line indicates the evolution time of catches.
The lack of genetic differentiation between the northern subarctic and southern subtropical stocks has been difficult to explain. We propose that the periods of contraction of the northern stock into the waters off Baja California leads to the mixing of spawners from the two stocks, as occurred during the prolonged period of cooling lasting roughly 30 years from the late 1940s through the mid-1970s. The prolonged period of cooling is clearly evident in the annual means of the PDO index beginning in 1900 (available from http:// www.atmos.washington.edu/~mantua/abst.PDO.html).
Ahlstrom (1960) indicated that sardines had disappeared from the region north of Point Conception by 1952 and moved southward into waters off the Baja California Peninsula, mainly between Punta Eugenia in central Baja California and Cabo San Lucas at the tip of the peninsula. According to Rodríguez-Sánchez et al. (2001), juvenile sardines were found inside the Gulf of California in the 1960s. This mixing of the spawners off Baja California would clearly be expected to result in similar genetic composition between the two stocks, and the lack of differentiation would be maintained as the subarctic stock expanded northwards into British Columbia in the early 1990s during the recovery of the population. This is supported by Zaitsev et al. (2014), who reported the presence of subarctic water mixing with subtropical surface water in front of Magdalena Bay from January 2011 to April 2012. The latter in turn agrees with the observation made by Félix-Uraga et al. (2013) at the 2013 meeting of the Trinational Sardine Forum in Ensenada, that fish from the northern stock were present in Magdalena Bay in 2011 and 2012. Their abstract reports "landings of Pacific sardine decreased progressively from 2007 to 2010. Subsequently catches increased in 2011 and 2012, probably because sardines from the northern stock migrated to the south where they joined with sardines of the southern stock. There is evidence that currently the Pacific sardine population in the California Current System is collapsing. When this happens, this resource seeks refuge in the south of its distribution range, and a mixture of northern and southern stocks is likely to occur. Larger and older sardines than usually recorded in Magdalena Bay could be evidence of this migration of northern stock to the southern coast of the Baja California Peninsula".
The sedimentary record of sardine scale deposition in the CCS beginning at approximately 400 AD indicates that the period of expansions and contractions of the sardine population is centered around 60 years (Baumgartner et al. 1992). This suggests that variability in ocean climate provides a mechanism for almost continuous mixing of the two stocks that has apparently prevented any significant genetic differentiation between the stocks since their appearance in the CCS thousands of years ago.
Smith (2005) reviewed the different methods that have been used to distinguish between the sardine stocks in the CCS: tagging (to discern migration histories), vertebral counts, differences in blood types, growth rates (size at age) and natural mortality rates, and the timing and location of recruitment. This author suggested using the change in number of vertebrae to indicate larval growth and temperature at the spawning site. Smith also suggested exploring the use of microchemistry and stable isotopes in otoliths of post-larval sardines to determine the composition and temperature of water at spawning locations in the Gulf of California and in the CCS (Valle-Rubio 2007). Smith (2005) identified three stocks by names that reflect their spawning areas: the Gulf of California, the inshore region off the southern Baja California Peninsula (our southern, subtropical stock), and the central California offshore region (our northern, subarctic stock). As we know, the offshore California stock and the inshore stock of Baja California overlap but do not mix and thus do not spawn together in "normal" years in the region between San Pedro (the fishing port in southern California) and Ensenada (the fishing port in northern Baja California).
Parrish et al. (1989) discuss the level of taxonomic separation between sardines from different ecosystems and emphasize that the level of genetic difference among subpop-ulations (stocks) in the same region is expected to be negligible relative to the time needed to acquire more significant differences over evolutionary time scales for geographically-and, therefore, reproductively-isolated populations, resulting in distinctive phenotypes with adaptation and genetic drift. It is possible for an isolated population to maintain its genetic identity but, under changing environmental conditions, it would develop a completely different life history. Thus, it is reasonable to expect that subpopulations may be distinguished by their morphometric and meristic differences even though, according to Stepien and Rosenblatt (1996) in their study of Pacific and jack mackerel, there is no detectable genetic difference.
Since sardine fishing preferentially removes adults, the population will eventually be comprised of more juveniles (Hsieh et al. 2006). Juvenile sardines are more susceptible than adults to changes in their environment brought on by variability in ocean climate. Anderson et al. (2008) did not find robust evidence of the fishing impact relative to juveniles in the population. However, year classes associated with the recruitment may reflect their greater presence in the fisheries (Zwolinski and Demer 2013). Similarly, observations by Hsieh et al. (2006) report a significant reduction in the average age of the sardine fishery in California in the period 1983-2004 that may be associated with the expansion of the subtropical stock. The greater presence of juveniles in the first semester catch off Ensenada may be the cause of the higher correlation with the PDO during the period 1997-2005 (Fig. 9). Fishing should actually produce homoge-nization of sizes since there will be fewer adults in the population. Thus, the subtropical stock is probably responsible for the strong correlation between the PDO and the ratio of second semester landings to first semester landings (R2 = 0.8 during 1997-2005), compared to the lack of correlation in the earlier phase of the fishery development (1990-1996), when the northern stock (during its recovery) dominated the Ensenada fishery except for the year prior to the 1992 El Niño (Fig. 8). During 2006-2010, the southern stock continuously dominated the catch off Ensenada. Landings of the subarctic stock during this period ranged from 22% (12,540 t in 2006) to 51% (18,771 t in 2007) of the total annual catch (Demer and Zwolinski 2014).
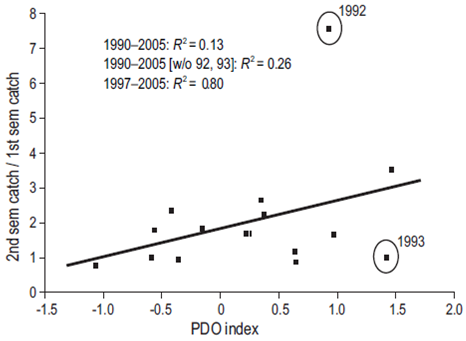
Figure 9: Ratio between second and first semester catches off Ensenada in relation to the Pacific Decadal Oscillation (PDO) index. The least-squares regression line (R 2 = 0.80) is given for the period 1990-2005.
The northern stocks of sardine and mackerel that live in low-salinity and cooler subarctic waters appeared to be declining during the ongoing cold regime that began in 1998 (Lo et al. 2010a, Weber and McClatchie 2012, Zwolinski and Demer 2012). This decline is associated with the intensification of offshore Ekman transport (Bjorkstedt et al. 2010). The regional cooling in surface temperature and the increase in offshore transport has resulted in a southward shift in bio-mass and more offshore transport of eggs and larvae, while the effects of the increased dispersion have actually decreased the concentrations of food available, limiting the size of annual year classes that are recruited to the adult population. This response was also observed during the cooling period that began in the late 1940s, pushing the remnants of both the sardine and Pacific mackerel biomass southward into the waters off northern Baja California, a region that is essentially a desert relative to the availability of food in the region off southern and central California (fig. 11 in Lo et al. 2005, Baumgartner et al. 2008). This scarcity of food apparently was the cause of the reproductive failures (Marr 1960) during each year until their combined biomasses had dwindled to a very small fraction, around 6000 t by 1963 (MacCall 1979). We believe that a similar situation may develop during the current period for both species as occurred during the 1950s and 1960s. This tendency is also found in the reduction in sardine egg density (<50 eggs m-3) observed from 2010 to 2013 in the region of central and southern California where temperatures range from 13 to 15 °C (http://swfsc.noaa.gov/).
Climatic indicators suggest that the northern subarctic stock should be the dominant sardine stock during this ongoing cold period that began in 1998. However, landings along southern California and Baja California indicate the dominance of the subtropical southern stock while the northern subarctic stock is apparently located south of Punta Eugenia. The reason is not yet clear for this unexpected dominance of the subtropical stock in northern Baja California during this cooling period. One effect is that it has lowered fecundity because of the smaller sizes of individuals. What is clear, however, is the need for collaborative monitoring between Mexico and the United States that would improve our understanding of the response to environmental forcing and would allow us to achieve the sustainable fisheries of our common small pelagic resources that we desire and are needed to insure the health of the CC ecosystem.
The study of the dynamics of the anchovy and sardine populations is based on different techniques that recognize at least a degree of structure using genetic (for anchovy) plus morphometric and meristic characteristics, as well as the location and area of their spawning grounds. Commercial catches in Baja California are generally taken near the coast, within a strip approximately 20 km offshore, and stock discrimination is based on SST that could be improved by also including sea surface salinity as shown in Figure 3. While discrimination allows for the generation of relevant time series between ocean climate and distribution and, therefore, the availability of the resource in space and time, it does not provide any information on stock abundance. There is a need for an objective, independent assessment of fish population abundances in near real-time which is now one of the features of the management plan that will cover the actively managed stocks. This will allow the fishing fleet to function more efficiently with the timing and location of the target resource that should result in savings of both time and operational costs.
Our experience with the use of the CUFES system on the IMECOCAL research cruises has shown that it provides high resolution of the distribution and abundance of near-surface eggs along the ship tracks and an indirect means for estimating the biomass of the small pelagic species found within the samples. Although the method is restricted to sampling in the upper 3-5 m of the water column, there is ample coverage over the survey region with ship tracks extending ~220 km offshore. We assume that the abundance of eggs collected represents the mean abundance of a 5-km strip on either side of the ship track. On our small research vessel (the Ulloa) we were not able to implement "adaptive sampling", meaning that we could not stop the ship once we identified an area with abundant eggs in order to make a vertical cast down to 70 m of a small net (CalVET) used to collect eggs within the mixed layer. In our new 49-m vessel it will be possible to scan each sample in real time to identify samples with a threshold of >10 eggs for 30 min of pumping.
Another important drawback of the current survey design is that the IMECOCAL tracklines are separated by a distance of 74 km and, according to the Nyquist theorem (Kelly 1976), we can only identify areas of spawning between track-lines (in the alongshore direction) that extend for distances greater than 148 km. Therefore, the distance between the tracklines would need to be reduced to less than 37 km. The survey design calls for four cruises a year to provide a seasonal picture of the annual state of the ecosystem (January, April, July, and October) that will allow us to determine the distribution and abundance as well as the seasonal change in character of the spawning habitat. A further disadvantage is that we only obtained information over a two- to three-week period on each of the quarterly cruises and thus can only infer what goes on between the cruises. The cost of ship time makes more frequent sampling prohibitive. The only alternative would be to run monthly lines in selected areas in small boats and this would mean trading time for distance. Moreover, our vessel is not currently equipped with hydroacous-tics to locate and estimate fish biomass, nor do we have proper trawling gear to obtain samples for identification and to obtain the adult parameters necessary for the biomass estimation. We thus have to use fish from commercial catches, but since the fleet operates so close to shore we cannot obtain samples of the larger adults offshore, at least in April, which is the peak spawning period of the subarctic stock. We intend to remedy this situation by obtaining the hydroacoustics and fishing gear, but will likely require up to four or five years to obtain financing to purchase and instal the equipment on our vessel.
Morphometric characteristics and distribution of spawning areas of sardine and anchovy stocks are evidence of adaption to specific environmental conditions. This depends mainly on food availability to cover the energetic demands of a population and partitions the CCS into regional habitats, in which each stock prefers a particular size and type of prey. Individuals of a stock use their excess energy for growth and reproduction. Reproductive peaks reflect a seasonal variability in environment conditions in which reproductive strategies are suited to their habitat. Anchovy with oval eggs that lack an oil globule spawn in areas of greater turbulence, whereas sardine with spherical, oil-rich eggs spawn in more stratified conditions outside the upwelling front. These environmental conditions appear to be responsible for the development of the phenotypic characteristics of the two sardine stocks. The genetic differentiation in anchovies is likely the result of their limited migration, in contrast with the lack of genetic differentiation in sardines that can be explained by their more highly migratory behavior that maintains this genetic homogenization. In the case of sardines, unfavorable environmental conditions lead to decreased recruitment and mixing of both stocks in the refuge centers off the Baja California Peninsula.
In summary, the objective of this review has been to provide a framework to describe the mechanisms and processes that alter ocean habitat and life history traits, and that structure the population of small pelagic fishes. This includes a description of the physical setting of the environment occupied by these species, their life cycles, and distribution patterns in the southern reaches of the CC ecosystem. We used a historical perspective to examine the relationship between landings and availability of fish to explain how the movements of these resources are sensitive to climate variability and thus determine when and where they are captured. This is done to illustrate the idea that climate determines the success or failure of the fishery through the availability of the resource. We have presented more complete descriptions of the behavior and character of only three of the four species of small pelagic fishes and their interaction with the ocean climate of the CCS. The relatively small amount of information on T. symmetricus has limited our description to include only distribution of habitat and that presented in Tables 1, 2, 3.
Environmental forcing associated with the regional wind field is largely responsible for the structure and maintenance of the condition of the spawning habitats and food availability. Habitat conditions depend on the variability of the different time and spatial scales that influence productivity and recruitment of a population. Monitoring the state of the ecosystem requires the development of tools that permit us to evaluate its productivity in relation to the environmental conditions, in combination with the effects of fishing. The goal is to construct time series of environmental variables that control the size and reproductive potential of the annual year classes of the fishery stocks. In order to explain the lack of genetic differentiation between the subarctic and subtropical stocks, we have proposed that the mixing and incorporation of individuals from the subtropical stock into the subarctic stock will result in the recruitment of juveniles from the subtropical stock into the subarctic stock and vice versa so that genetic mixing is complete by the time their respective migrations are underway. It is important to emphasize that determining the patterns of movement of stocks and the areas where their overlap is critical to achieving sustainable fisheries.