In 2021, chili pepper (Capsicum annuum) production in Mexico reached 3,086,742 t, valued at 30,642.66 million pesos (SIAP, 2022). Despite this, various root diseases have been affecting chili pepper yields in recent years, resulting in wilting and premature plant death (Pérez-Acevedo, 2017). Depending on chili genotypes, environmental conditions, pathogenicity of the pathogens, and inoculum quantity in the soil, wilting can cause losses of 40 to 100% in chili pepper cultivation (Tembhurne et al., 2017). This disease complex is caused by Rhizoctonia solani, Fusarium oxysporum, F. solani, and Phytophthora capsici (Montero-Tavera et al., 2013), which lead to wilting, premature leaf drop, chlorosis, necrosis, strangulation at the stem base, and soft, watery rot due to vascular bundle obstruction (Pérez-Acevedo, 2017). P. capsici can cause loss of turgidity, yellowing leaves, necrosis at the stem base, and plant death in under 25 days (Gómez-Hernández et al., 2018; Pérez-Acevedo et al., 2017). Fusarium spp. causes leaf discoloration and curling, followed by total wilting due to damage to the vascular system and interruption of physiological processes (Morid et al., 2012). During the first days of seedling growth, the association of Rhizoctonia sp. and Phytium sp. leads to wilting, chlorosis, necrosis at the stem base, epidermis fall, and plant death (Pérez-Acevedo et al., 2017).
Crop management practices for these pathogens, including agrochemical application, control of soil moisture, and cultural practices (González-Chavira et al., 2009). However, in recent years, studies have focused on biological control to reduce agrochemical use, protect consumer health, and minimize environmental impact (Zhao et al., 2021). Bacteria, actinomycetes, and mycoparasitic fungi are the most commonly used microorganisms for controlling P. capsici, R. solani, and F. oxysporum (Yadav et al., 2021). These biological control agents occur naturally in the soil microbiota, where they can persist in a microscopic mix of thousands and millions of microorganisms per gram of soil (Ezziyyani et al., 2004). The importance of biological control relies significantly on native microorganism species, which can function as antagonists through antibiosis, competition for space or nutrients, direct interactions with the pathogen (mycoparasitism and enzymatic lysis), and induction of resistance.
Due to the economic significance of chili cultivation in Guanajuato, Mexico, the objective of this study was to isolate antagonistic fungi from soil samples collected in production areas located in the southern region of the state, with the purpose of determining their effectiveness against fungi associated with chili wilt. By conducting this investigation, valuable local information is provided for the biological control of wilt-causing fungi.
In July 2017, fungi and oomycetes associated with chili wilt were isolated from three plots of San Luis cv. poblano chili plants at the Bajio Experimental Field of the National Institute of Forestry, Agriculture and Livestock Research (INIFAP). Five plants displaying symptoms of wilt, stem rot, stunting, thick stem, and shoot death selected from each plot, placed in polyethylene bags inside a cooler, and transported to the laboratory for processing. Soil collections were carried out during the OI-2018 and PV-2019 cycles in 25 locations across eight chili-producing municipalities in Guanajuato, where plants in vegetative growth with characteristic symptoms of wilt, stem rot, chlorosis, and stunting were recorded. It was observed in both cycles that no agronomic management was implemented, including soil disinfection before planting; poor weed control, and waterlogging caused by uneven terrain or leaks from the irrigation tape. Five soil subsamples were taken from around the chili plants at a depth of 15-20 cm and homogenized to obtain a composite sample of 500 g (Table 1).
Table 1 Soil and chili bell pepper plant collection sites for isolation of antagonistic organisms associated with chili wilt in southern Guanajuato. Sampling cycles, Fall-Winter 2018 and Spring-Summer 2019.
Municipio | Localidad | Coordenadas geográficas | Variedades de chile por unidad de producción |
---|---|---|---|
O-I/2018 | |||
Celaya | Rancho “Estrada” | 20.530793; -100.869292 | Crusadier, Aristóteles |
Irapuato | Cuarta Brigada | 20.623832; -101.275164 | Crusadier, Aristóteles |
Irapuato | Rancho “Los Lobos” | 20.592263; -101.31285 | Crusadier; Aristóteles |
Villagrán | Rancho “El Pintor” | 20.514751 -100.944374 | Imperial |
Silao | Loma Bonita | 20.992519; -101.507566 | Húngaro |
Silao | Rancho “Santa Faustina” | 20.887298; -101.435176 | Secuoya, Estrella |
Romita | Fracción Puerta Grande | 20.88635; -101.495124 | Don Vicente |
Pénjamo | Rancho “Buenos Aires” | 20.381657; -101.834033 | Arista |
Pénjamo | Rancho “San Gabriel” | 20.429055; -101.598688 | 5807 |
Abasolo | Rancho “El Palomar” | 20.413018;-101.556484 | 5807 |
P-V/2019 | |||
Cortazar | Rancho “Las Fuentes” | 20.447392; -100.969701 | Serrano |
Villagrán | Rancho “La Charca” | 20.500479; -101.026391 | Bravo |
Villagrán | 20.490822; -101.027278 | Serrano | |
J. Rosas | Santiago Cuenda | 20.599575; -101.002517 | Serrano |
Abasolo | En nombre de Dios | 20.548681; -101.549556 | Serrano |
La Galera | 20.441680; -101.569290 | Serrano | |
Rancho 1 | 20.450681; -101.595814 | Serrano | |
Rancho “El Pitayo” | 20.470594; -101.575088 | Tajín | |
Pénjamo | Rancho “Buenos Aires” | 20.386537; -101.837438 | Jalapeño |
Guanajuato | San José de Llanos | 20.845623; -101.315313 | Jalapeño |
Silao | Rancho “Santa Faustina” | 20.889908;-101.438975 | Pimiento |
Parcela junto RSF | 20.924923; -101.433522 | Pimiento | |
Rancho de Elisa | 20.935769; -101.435210 | Pimiento | |
Romita | El Ranchito | 20.893356; -101.488338 | Jalapeño |
La Aldea | 20.898463; -101.481889 | Serrano |
To process the wilted plants, we removed their aerial parts, preserved the stem, root collar, and root, and made 1 cm2 tissue cuts. The cuts were disinfected with 5% sodium hypochlorite for 30 s and washed twice with sterile water. We placed the tissue on Potato Dextrose Agar (PDA) medium and incubated it at 25 °C. After five to seven days, we observed mycelial growth and isolated the colonies that developed. We identified all strains of interest according to their morphological characteristics (Sneh et al., 1991; Van der Plaats-Niterink, 1981; Barnett and Hunter, 1972). To purify the strains, we re-isolated them on PDA using hypha tips (Rhizoctonia sp., Phytium sp., and Phytophthora sp.) and single conidial cultures (Fusarium sp. and Alternaria sp.).
The soil samples underwent serial dilutions (101-107), with 1 g of sieved soil sample taken and saline solution (0.8% NaCl) used as the diluent. After homogenization in a Genius® vortex for 1 minute, 500 µL of dilutions 103, 105, and 107 were cultivated in triplicate on Potato Dextrose Agar (PDA) and Luria Agar (LA) by surface spreading, resulting in 18 culture plates per sample. The plates were then incubated at 25°C for 72 h. Fungi that grew on the culture media were re-isolated on PDA and identified at the genus level based on their macroscopic and microscopic characteristics, including size, color, type of mycelium, and colony shape, as well as hyphae, mycelium, conidia, and conidiophores. Colonies with similar morphological characteristics were discarded, and those with slow growth were eliminated. Monosporic cultures were used to obtain pure strains. To pre-select fungi with possible antagonistic capacity, dual confrontations with Phytophthora spp., Fusarium spp., and Rhizoctonia spp. were performed. Four points were marked on a PDA culture plate, separated by 1.7 cm, and the candidate antagonist microorganism was seeded at the first point (approximately 1 cm from the edge of the plate), with the remaining three points seeded with the fungus. Possible antagonists were seeded three days prior to the confrontation to give them an advantage in growth. The incubation was carried out at 25°C for five days. The confrontations were performed in triplicate, using 12 culture plates and control. To determine the percentage of radial growth inhibition (PRGI), the growth radius of Phytophthora spp., Fusarium spp., and Rhizoctonia spp. was measured, and the formula used by Suárez et al. (2008) was employed to calculate the PRGI:
Where R1: radius of the fungus associated with wilt (control) and R2: radius of the fungus associated with wilt against the antagonist.
The fungi with a PRGI greater than 50% and an antagonistic effect against the confronted fungi were selected. To conduct this test, a PDA medium disk with Phytophthora spp., Fusarium spp., and Rhizoctonia spp. mycelium was placed 1 cm from the edge of the Petri dish, and the antagonist fungus was placed at the other end (Correa et al., 2007). Ten repetitions were carried out for this test. The growth diameters of the fungi associated with wilt were measured at 120 hours. The radial growth of Phytophthora spp., Fusarium spp., and Rhizoctonia spp. in the absence of the antagonist was determined as a control. The PRGI was determined using the formula proposed by Suárez et al. (2008). Additionally, the degree of antagonism of the selected isolates was determined using the scale of Bell et al. (1982), which classifies the area that the antagonistic organism colonizes in the presence of the phytopathogen into six categories. The PRGI in the pre-selection and dual cultures were analyzed using a factorial design of two factors: antagonist (11 levels) and pathogen (3 levels). The data were transformed using the arcsine function and subjected to analysis of variance and mean comparison. The R version 1.1.1717 software, PBC, was used for the statistical analysis.
DNA extraction from 15-day-old fungal strains was performed using the protocol described by Lievens et al. (2003). Genomic DNA was quantified at a wavelength of 260 nm using the Nanodrop 8000 spectrophotometer (Thermo Scientific®), and its integrity was verified by electrophoresis on 1% agarose gels. All DNA samples were diluted to concentration of 10 ng µL-1. The internal transcribed spacer (ITS) region of the fungal 18S ribosomal DNA component was amplified using the primers ITS1 (5’TCCGTAGGTGAACCTG-CGG3’) and ITS4 (5’TCCTCCGCTTATTGATATGC3’), as described by White et al. (1990). The expected final PCR product was 650 bp. The PCR product was purified using the ZR DNA Sequencing Cleanup KitTM D4050S following the manufacturer’s protocol. Sequencing was performed at the Genomic Services Department of Langebio, CINVESTAV-Campus Irapuato, and the obtained information was analyzed using the Finch TV program (Version 1.4.0) and compared against the BLAST: Basic Local Alignment Search Tool database.
Microorganisms associated with chili wilt were identified in the collected samples, with Fusarium spp. being the most prevalent, followed by Rhizoctonia spp. and Phytophthora spp. In addition, the presence of Pythium spp. and Alternaria spp. was also observed. Table 2 presents the observed symptoms in the collected plants along with the corresponding fungus or oomycete identified.
Table 2 Isolates of fungi and oomycetes associated with chili wilt in symptomatic plants collected in southern Guanajuato, Mexico.
Síntoma | Tejido muestreado | Hongo aislado |
---|---|---|
Marchitez de planta, achaparramiento | Raíz, tallo | Rhizoctonia spp. (binucleada) |
Marchitez de planta, achaparramiento | Raíz, tallo | Rhizoctonia spp. (mono nuclear) |
Muerte de brote | Raíz, tallo | Rhizoctonia spp. (binucleada) |
Muerte de brote | Raíz | Pythium spp. |
Muerte de brote | Raíz, tallo, cuello de raíz | Fusarium spp. |
Muerte de brote | Raíz | Alternaria spp. |
Muerte de brote | Raíz | Alternaria spp. |
Tallo grueso | Tallo | Alternaria spp. |
Marchitez | Raíz, tallo, cuello de raíz | Phytophthora spp. |
In total, 120 fungi with potential antagonistic activity were isolated and screened for pre-selection as antagonistic agents based on their morphology and growth rate, resulting in the selection of 11 strains. The analysis of variance revealed a significant interaction between the two factors (Table 3) at a significance level of 0.001. H5P1 exhibited the highest inhibitory effect against Phytophthora spp. (80.12%), whereas HMZA (80.1%) and H6P2 (82.41%) showed similar inhibitory effects against Fusarium spp. for Rhizoctonia spp., both HMZA (80.6%) and H6P2 (81.69%) showed the highest inhibitions. The lowest PRGI was observed for the confrontation of Rhizoctonia spp. against H11P (16.44%). Therefore, strains H5P1, H6P2, and HMZA were selected due to their consistent antagonistic potential with PRGI in the range of 50 to 82% and were identified at the molecular level.
Table 3 Percentage of Radial Growth Inhibition (PRGI %) of pre-selected strains of fungal isolates with antagonistic potential for fungi associated with chili wilt in southern Guanajuato, Mexico.
Hongo | Hongos antagonistas | ||||||||||
---|---|---|---|---|---|---|---|---|---|---|---|
HMZA | H4P5 | H4C12 | H5P1 | H6P2 | H11P | H13P | H14P | H14P3 | H20P | H22P | |
Phy | 71.4 ± 2.4 cde | 31.45 ± 1.6 nopq | 55.58 ± 0.5 f | 80.12 ± 1.9 ab | 75.38 ± 1.4 bcd | 25.58 ± 2.3 pqr | 50.04 ± 2.3 fghi | 25.14 ± 1.8 gr | 29.48 ± 4.4 nopq | 27.55 ± 2.1 opqr | 25.46 ± 2.0 pqr |
Fus | 80.1 ± 1.1 ab | 20.72 ± 1.5 rs | 43.94 ± 1.8 ghij | 66.67 ± 1.2 e | 82.41 ± 1.3 a | 35.86 ± 2.2 jklmn | 35.94 ± 1.1 jklmn | 50.44 ± 2.0 fgh | 33.49 ± 2.5 lmnop | 52.41 ± 2.6 fg | 52.81 ± 1.8 f |
Rhi | 80.6 ± 1.2 ab | 42.33 ± 2.2 hijk | 30.76 ± 0.9 nopq | 77.71 ± 0.9 bc | 81.69 ± 2.3 a | 16.47 ± 3.9 s | 41.57 ± 3.6 ijkl | 35.22 ± 3.5 klmno | 57.23 ± 7.4 f | 53.13 ± 5.0 f | 40.08 ± 2.6 jklm |
Phy: Phytophthora spp., Fus: Fusarium spp., Rhi: Rhizoctonia spp. Note: Equal letters do not present significant statistical differences (p<0.001). Values correspond to the mean and ± standard deviation
The ITS1 and ITS4 primers were used for amplification, resulting in amplicons of approximately 650 bp, which are characteristic of fungal isolates (White et al., 1990). Following sequencing and gene comparison using BLAST, the isolates were identified with 100% homology. Aspergillus flavus (H5P1), Penicillium sp. (H6P2), and Trichoderma harzianum (HMZA) were identified as the antagonistic fungi (GenBank accession no. KM386418.1, KP131554.1, KJ598874.1, respectively). The average PRGI of the antagonist strains against Phytophthora spp., Fusarium spp., and Rhizoctonia spp. was observed to be 50-82% (Table 4). An interaction was found between the levels of antagonist and pathogen at a significance level of 0.001. Under the dual confrontation technique, similar behavior was observed, where A. flavus (79.72%) and Penicillium sp. (75.30%) showed greater inhibition of radial growth of Phytophthora spp., while T. harzianum exhibited higher inhibition against Fusarium spp. and Rhizoctonia spp. (82.55% and 80.36%, respectively). The lowest inhibition percentages were observed in the confrontation of Fusarium spp. against Penicillium sp. (50.77%) and Rhizoctonia spp. against Aspergillus flavus.
Table 4 Percentage of radial growth inhibition (PRGI) of antagonistic fungi isolated against fungi associated with chili wilt in southern Guanajuato, Mexico.
Hongo | T. harzianum | A. flavus | Penicillium sp. |
---|---|---|---|
Phytophthora spp. | 70.19 ± 2.03 cd | 79.72 ± 2.06 ab | 75.30 ± 2.55 bc |
Fusarium spp. | 82.55 ± 1.29 a | 66.83 ± 11.69 de | 50.77 ± 2.89 g |
Rhizoctonia spp. | 80.36 ± 1.59 ab | 59.18 ± 2.01 f | 62.59 ± 2.00 ef |
Note: Equal letters do not present significant statistical differences (p<0.001). Values correspond to the mean and ± standard deviation.
Additionally, it was determined that the obtained antagonism belonged to class 2 and 3 according to Bell et al.’s (1982) classification system (Table 5). Class 2 antagonism covered two-thirds of the pathogen’s growth area, while class 3 was characterized by the antagonistic agent and pathogen colonizing half of the surface each. Class 3 antagonism was observed in the confrontations of Penicillium sp. against Phytophthora spp. and Rhizoctonia spp., and T. harzianum against Rhizoctonia spp., as depicted in Figure 1.
Table 5 Antagonism class according to Bell et al. (1982) of T. harzianum, A. flavus and Penicillium sp. vs fungi associated with chili wilt.
Hongo antagonista | Hongo | Clase de antagonismo |
---|---|---|
Trichoderma harzianum | Phytophthora spp. | 2 |
Fusarium spp. | 2 | |
Rhizoctonia spp. | 3 | |
Aspergillus flavus | Phytophthora spp. | 2 |
Fusarium spp. | 2 | |
Rhizoctonia spp. | 2 | |
Penicillium sp. | Phytophthora spp. | 3 |
Fusarium spp. | 2 | |
Rhizoctonia spp. | 3 |
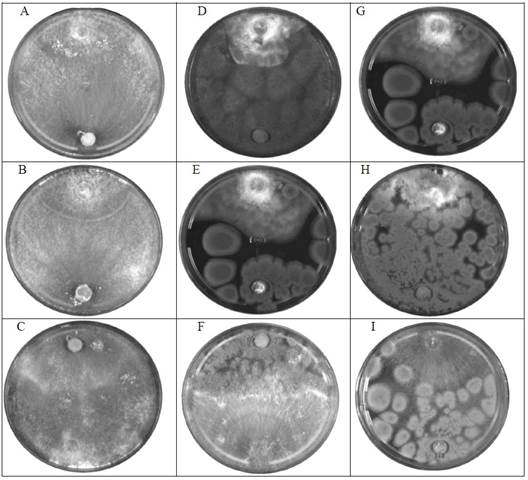
Figure 1 Dual confrontation (phytopathogen vs. antagonist) Phytophthora spp., Fusarium spp. and Rhizoctonia spp. A) T. harzianum vs Phytophthora spp., B) T. harzianum vs Fusarium spp., C) T. harzianum vs Rhizoctonia spp., D) A. flavus vs Phytophthora spp. F) A. flavus vs Fusarium spp., G) A. flavus vs Rhizoctonia spp., H) Penicillium sp. vs Phytophthora spp., J) Penicillium sp. vs Fusarium spp., K) Penicillium sp. vs Rhizoctonia spp. Above: pathogen strain, below: antagonist strain. Cultures of 5 days of growth.
The fungi recovered from plants exhibiting wilt symptoms are consistent with those reported by researchers such as Gómez-Hernández et al. (2018), Albañíl-Juárez et al. (2015), and Velásquez-Valle et al. (2013), who linked the disease to P. capsici, Rhizoctonia sp., Fusarium sp., Verticillium sp., and Pythium sp. Alternaria sp was found in the roots of plants that displayed shoot death, although this pathogen is not always detected in chili wilt. Vásquez-López et al. (2009) identified Alternaria in symptomatic plants, but they could not accurately determine its pathogenicity. Meanwhile, Garibaldi et al. (2019) and Begum et al. (2010) demonstrated that Alternaria alternata and Alternaria tenuis caused symptoms and even severe damage to the production. Albañil-Juárez et al. (2015) reported that F. oxysporum had the highest incidence, followed by Rhizoctonia sp. and Phytophthora sp., in the Bajio and southwest of Guanajuato.
The three antagonistic fungi that exhibited the highest potential for induced systemic resistance (PRGI) have been previously documented as biocontrol agents against various pathogens. For instance, Polanco-Florian et al. (2020) identified Aspergillus and Penicillium as biocontrollers of Lasiodiplodia theobromae and Fomitopsis meliae, which are the causative agents of citrus stem-end rot. These fungi were previously considered as saprophytes and toxin producers; however, atoxigenic strains that act via competitive exclusion have been identified, making them suitable for use in commercial formulations of biocontrol products. Trichoderma, a widely used biocontrol agent, is commercially available as a resistance stimulator, biofertilizer, bioplaguicide, and growth enhancer (Asad, 2022). The biocontrol mechanism of Trichoderma against soil-borne pathogens has been extensively studied. Abd-El-Khair et al. (2019) reported that Trichoderma could stimulate plant defense via the production of enzymes that inhibit the pathogen, thereby reducing root wilt and rot. Jamil (2021) indicated that Trichoderma acts as an endophytic symbiont by colonizing plant roots and secreting beneficial chemical stimulants for plants. Michael-Aceves et al. (2009) demonstrated that Trichoderma species maintain a scale of 1 to 3 values of mycoparasitism against phytopathogens. The selection of Trichoderma strains for biocontrol depends on their aggressiveness to mycoparasitism and their growth rate advantage over the pathogen (Guédez et al., 2012). In this work, T. harzianum was observed to cover or grow over the phytopathogen Phytophthora spp. in less than a week at room temperature, similar to the observations made by Ezziyyani et al. (2004), where T. harzianum was capable of surpassing P. capsici and reducing the pathogen’s colony in just four days during in vitro confrontation. In the case of root pathogens, Andrade-Hoyos et al. (2019) demonstrated the effectiveness of T. asperellum, T. viride, and T. harzianum isolated from soil against Rhizoctonia sp., Fusarium sp., and Phytophthora capsici, with over 85% inhibition of the strains. The authors attributed this response to the synthesis of secondary metabolites and mycoparasitism mechanisms of action. In another study by Mousumi-Das et al. (2018), T. harzianum exhibited inhibition against F. oxysporum of 78.3%, against P. capsici of 65.3%, and against R. solani of 45.3%. Lastly, Miguel-Ferrer et al. (2021) reported a PGRI of 53% and a class 2 antagonism on the Bell scale of T. harzianum against F. solani.
Penicillium shown to control pathogens through nutrient competition, mycelium disintegration, and lytic action antibiosis (Jiménez-Camargo et al., 2018). In addition, the production of antimicrobial compounds, such as Glandicolina B, has been identified (Sánchez-Fernández et al., 2013). As for Aspergillus, it is generally capable of inhibiting pathogen growth via antibiosis and nutrient competition (Quiroz-Sarmiento et al., 2008). This fungus has also found to produce antifungal compounds, such as Fumitremorgin B (Sánchez-Fernández et al., 2013).
Atalla et al. (2020) demonstrated that Aspergillus oryzae reduced the linear growth of root rot-causing pathogens, including A. alternata, F. oxysporum, Phoma destructor, R. solani, and Sclerotium rolfsii, through the production of xylanase in fava bean. However, Quiroz-Sarmiento et al. (2008) noted that although some strains of Aspergillus exhibit antagonism against root pathogens, pathogenicity tests are necessary to evaluate the possible production of enzymes and toxicological risks to the plant. There are studies demonstrating effective biological control of root-associated pathogens using Penicillium. Boughalleb-M’Hamdi et al. (2018) found that applying P. italicum and P. digitatum reduced root damage and disease severity in melon seedlings. The degree of antagonism of Aspergillus and Penicillium has not been extensively documented, and there are few studies regarding the type of antagonism they exhibit. Rather than defining the type of antagonism, the percentage of inhibition of pathogen growth is referred to Silva-Acosta and Paez-Redondo (2021) evaluated the effect of strains of Aspergillus, Penicillium, and Trichoderma on the control of Phytophthora sp. associated with oil palm. Their results showed that A. niger and Trichoderma sp. exhibited 74% and 79% inhibition in the growth of the oomycete, respectively. Moreover, Almeyda-León et al. (2020) identified Aspergillus and Penicillium as biocontrollers in the growth of Macrophomina phaseolina in percentages above 60%. Similarly, Jiménez-Camargo et al. (2018) demonstrated that strains of Penicillium caused inhibitions ranging from 54% to 81% in the development of P. capsici isolated from seedlings with wilt symptoms.
Rhizoctonia spp., Phythium spp., Fusarium spp., Alternaria spp. and Phytophthora spp. were isolated from chili bell pepper plants grown in production fields located in southern Guanajuato, Mexico. Three strains, namely T. harzianum, A. flavus, and Penicillium sp., exhibit constant antagonism against these pathogens, resulting in inhibition levels ranging from 41% to 83%. Other studies are required to determine if A. flavus and Penicillium sp. can be used as effective biocontrol agents. These findings suggest that these three organisms are an alternative to control phytopathogens associated with soil wilt in production areas. In light of the in vitro inhibitory effect observed, it is crucial to continue and scale up the potential control of the disease in situ to ensure its efficacy and practical application.