Servicios Personalizados
Revista
Articulo
Indicadores
-
Citado por SciELO
-
Accesos
Links relacionados
-
Similares en SciELO
Compartir
Revista mexicana de fitopatología
versión On-line ISSN 2007-8080versión impresa ISSN 0185-3309
Rev. mex. fitopatol vol.38 no.3 Texcoco sep. 2020 Epub 27-Nov-2020
https://doi.org/10.18781/r.mex.fit.2005-3
Phytopathological Notes
Scanning electron microscopy and enzymatic analysis in Agave americana during Fusarium oxysporum infection
1 Laboratorio de Biotecnología Vegetal, Instituto Tecnológico de Tuxtla Gutiérrez, Tuxtla Gutiérrez, Chiapas, México; Unidad de Bioquímica y Biología Molecular de Plantas, Centro de Investigación Científica de Yucatán, Mérida, Yucatán, México;
2 Unidad de Biotecnología, Centro de Investigación Científica de Yucatán, Mérida, Yucatán, México;
3 CONACYT-Tecnológico Nacional de México/Instituto Tecnológico de Tuxtla Gutiérrez, Tuxtla Gutiérrez, Chiapas, México;
4Laboratorios de Fitopatología, DEPI del Instituto Tecnológico de Tlajomulco, Tlajomulco de Zuñiga, Jalisco, México.
Chitinase and β-1,3-glucanase play an important role in the defense reaction against fungal pathogens. In Agave americana there is limited information on the behavior of these proteins in response to the Fusarium oxysporum attack. In this study, a scanning electron microscopy (SEM) analysis was performed using roots of A. americana infected with F. oxysporum, and the activity of chitinase and β-1,3-glucanase in roots of A. americana was evaluated to 0, 7, 15 and 30 days after inoculation with F. oxysporum. SEM revealed that 15 days after inoculation (DAI) is enough for the fungus to infect the roots of A. americana. It was observed that the activity of chitinase in infected plants increased significantly at 15 DAI with respect to non-infected plants, while the activity of β-1,3 glucanase did not show a significant statistical difference with control plants. These results suggest that, in response to F. oxysporum infection, the activation of the chitinase-type PRs proteins is induced in A. americana.
Key words: enzyme activity; β-1,3-glucanase; chitinase; agave wilting
La quitinasa y β-1,3-glucanasa desempeñan una función importante en la reacción de defensa contra patógenos fúngicos. En Agave americana existe poca información sobre el comportamiento de estas proteínas en respuesta a la infección de Fusarium oxysporum. En este estudio, se realizó un análisis de microscopía electrónica de barrido (MEB) en raíces de A. americana infectadas con F. oxysporum y se evaluó la actividad de quitinasa y β-1,3-glucanasa en raíces de A. americana a 0, 7, 15 y 30 días después de inoculación con F. oxysporum. MEB reveló que 15 días después de la inoculación (DDI) son suficientes para que el hongo infecte las raíces de A. americana. La actividad de quitinasa en plantas infectadas incrementó significativamente a los 15 DDI con respecto a las plantas no infectadas, mientras que la actividad de β-1,3 glucanasa no presentó diferencia estadística significativa con plantas testigo. Los resultados sugieren que, en respuesta a la infección por F. oxysporum, A. americana activa proteínas PRs de tipo quitinasas.
Palabras clave: actividad enzimática; β-1,3-glucanasa; quitinasa; marchitez del agave
Plants are continually defending themselves from phytopathogens by developing a wide range of responses that let them recognize, stop, and counteract infections in time (Prusky et al., 2013). The family of PRs proteins consists of a group of vegetal proteins that are thought to play an important role in resistance to diseases caused by diverse pathogens in plants, including fungi, viruses, bacteria, and oomycetes (Mahendranathan et al., 2016). This group of proteins is widely distributed in the plant kingdom; in healthy plants, PRs are found in minimum amounts, but their expression is significantly increased during and/or after a pathogen attack (Durrant and Dong, 2004). Among the PRs proteins, chitinase (PR3) and β-1,3-glucanase (PR2) are two groups of hydrolytic enzymes that increase their concentration in different plant species after phytopathogenic fungal infection. These enzymes play an important role in the defense reaction against pathogens by degrading the cell wall of the pathogen, because chitin and β-1,3-glucan are important structural components of pathogens cell wall. The β-1,3-glucanase seem to be coordinately expressed along with the chitinases after fungal infections in plants (Durrant and Dong, 2004).
One of the most serious phytosanitary problems that the Agave genus faces is the wilt and dry bud rot disease, which is mainly associated with the fungus F. oxysporum (López-Bautista et al., 2020). This pathogen attacks Agave plants regardless of their development stage. The infection starts as whitening of the basal leaves, followed by wilt, necrosis, and rot inside the bud, which eventually causes the plant to die (Flores et al., 2009; López-Bautista et al., 2020). However, the enzymatic defense activity that is promoted during the infection caused by F. oxysporum in Agave spp. is unknown, the objective of this study was to evaluate the activity of the proteins associated with the pathogenicity, such as β-1,3-glucanase and chitinase on A. americana plants infected with F. oxysporum, as well as to observe the pathogen intracellular growth in agave roots using the scanning electron microscopy technique.
The F. oxysporum isolate that was used in this study was obtained from tissue of the stem base of an A. americana plant approximately three years after being transplanted; the plant had wilt symptoms, as well as chlorosis, and leaf rolling. The plant was found in a commercial agave plantation in the Comitán municipality, Chiapas, Mexico (16° 17’ 30” N, 92° 10’ 1” W). For isolation, cuts approximately 0.25 cm in size per side of stem segments from the crown area were made and then disinfected by submerging them for 2 min in a mixture of sodium hypochlorite (6%), ethyl alcohol (100%) and sterile distilled water at 1:1:8 ratio. The fragments were rinsed twice with sterile distilled water, and then sown in potato-dextrose-agar (PDA) culture medium supplemented with 0.12 g L-1 of streptomycin and 0.25 g L-1 of chloramphenicol. Once morphologically identified, according to the characteristics reported for F. oxysporum (Leslie and Summerel, 2006), a monosporic culture of the fungus was obtained, sown again in PDA culture medium, and covered with a sterilized sweet cellophane film so mycelium can grow and be easily removed. The mycelium was used to obtain DNA and amplify the ITS1-5.8S-ITS4 fragment using end-point PCR and the primers ITS1 5´-TCCGTAGGTGAACCTGCGG-3´ and ITS4 5´-TCCTCCGCTTATTGATATGC-3´ (White et al., 1990). The strain was reactivated in PDA by incubation at 28±2 °C for 15 days, and after that period, a mycelial square 1 cm2 in size was inoculated in 250 mL of dextrose-potato broth (24 g L-1). The flask was kept in constant agitation at 120 rpm for 10 days to obtain a spore suspension. The concentration of conidia was determined using a Neubauer chamber.
On the other hand, inoculation was made in six-month old A. americana plants obtained in vitro that were previously acclimated under greenhouse conditions for three months. The plants were transplanted to 10 cm plastic pots filled with a mixture of peat moss and agrolite (1:1), previously sterilized at 121 °C temperature and 15 lb. pressure for one hour, and then kept for 60 days in a growth chamber at 25 °C temperature. The inoculum consisted of a F. oxysporum conidia suspension (2 x 108 conidia mL-1) that was applied to the root of the transplanted plants, where a wound of approximately 0.5 cm in size was previously made. Once this procedure was concluded, the plants were incubated in a growth chamber at 28 °C controlled temperature and 60-90% relative humidity. Sixty plants were inoculated (three replications with 20 plants in each replicate) and 20 plants without inoculation (control).
To observe the F. oxysporum infection process in A. americana plants, root samples of five infected plants were collected at 7, 15 and 30 days after inoculation (DAI), and roots of five non-inoculated plants were also taken. The samples were processed according to the protocol described by Ruíz-May et al. (2011) with slight modifications. The samples were fixed with glutaraldehyde (2.5% v/v) in a phosphate buffer (0.2 M; pH 7.2) for 72 h. Then, the samples were rinsed with a phosphate buffer (0.2 M; pH 7.2), gradually dehydrated with ethanol (30%, 50%, 70%, 85%, 96% and 100%) and dried with liquid CO2 (Balzers CPD 020 Critical Point Dryer; Bal-Tec, Schalksmuhle, Germany). Samples approximately 0.5 mm in size were mounted on metal pieces with carbon conductive adhesive tape coated by sputtering with colloidal gold and observed at 10-20 kV using a Zeiss DSM 940A scanning electron microscope (Zeiss, Oberkochen, Germany).
Root samples of five plants infected by F. oxysporum and five control plants were collected at 0, 7, 15 and 30 DAI; this procedure was done three times. The enzyme extract was obtained using the methodology described by Pan et al. (1991) with slight modifications. Briefly, 0.3 g of A. americana lyophilized roots were homogenized using liquid nitrogen, with 1.5 mL of extraction buffer (sodium acetate 0.05 M, pH 5.0, to which 250 mM of saccharose, 50 mM of NaCl, 1mM of β-mercaptoethanol and 1 mM (PMS) were added) to which 0.15 g of polyvinyl polypyrrolidone (PVPP) were added. The homogenate was centrifuged at 13,000 rpm for 30 min at room temperature, and finally, the supernatant was stored at -20 °C to be used in the enzyme essays. The protein contents of the enzyme extracts were determined by the method of Bradford (1976).
The chitinase or β-1,3 glucanase activity was determined using enzymatic extracts of the roots collected at 0, 7, 15 and 30 DAI with F. oxysporum, as well as from the control plants. The chitinase essay was done according to the methodology of Ferrari et al. (2014) with slight modifications. For the reaction mixture with the enzyme extracts, 50 µL of 0.05% glycol-chitin as substrate and 448 µL of 0.5 mM acetate buffer (pH 5.0) were used; tubes were incubated in a water bath at 40 °C for 30 min, and, once this procedure concluded, the tubes were cooled and a potassium ferrocyanide solution (C6FeK4N6) was added. The tubes were kept at 95 °C for 15 min; after this time, the tubes were cooled, then the absorbance was determined at 420 nm. The pattern curve was obtained with N-acetylglucosamine. The chitinase activity was expressed as units of µmol min-1 mg-1 of protein.
The β-1,3 glucanase activity was determined by the method of Honorato et al. (2015). The reaction started by adding 7 μL aliquots of the enzyme extract in a mixture of 986 μL of 50 mM sodium acetate buffer (pH 5.0) and 7 μL of laminarin substrate (0.15%). The reaction mixture was incubated in a water bath at 40 °C for 10 min. After the incubation period, the amount of the reducing sugars was determined by adding 333 μL of dinitrosalicylic acid (DNS) to the mixture and the resulting mixture was incubated in a water bath at 90 °C for 10 min. The reaction was interrupted by cooling the samples on ice for 5 min. The absorbances of the samples were measured at 540 nm wavelength. The glucanase activity was expressed in units of µmol min-1 mg-1 of protein.
The data obtained was analyzed using an analysis of variance (ANOVA) in order to determine if there were significant differences between the treatments evaluated, followed by a comparison of means using Tukey test (p≤0.05). The statistical analysis of the data was conducted using the STATGRAPHICS® Centurion XVI.II software (Statgraphic, 2010).
The isolate was molecularly confirmed as Fusarium oxysporum, according to the database of the GenBank, and named ITTG_Foxy_C6, which is part of the strain pool of the Technological Institute of Tuxtla Gutiérrez, with accession number in the GenBank (MT791313).
The colonization of roots by F. oxysporum was observed using scanning electron microscopy in the different sampling days (Figure 1). Although the longitudinal cuts made to the roots at 7 DAI did not indicate the presence of the fungus, mycelium and spores were observed on the root surface, which suggested that the fungus had not yet entered the root. Czymmek et al. (2007) and Padilla-Ramos et al. (2018) mentioned that, during the attachment of spores to the root surface, the fungus forms a dense network of hyphae (mycelium) and releases secretions and/or effectors that allow penetration. In addition, electronic microscopy has shown the absence of appressoria or specialized penetration hyphae of F. oxysporum, as well as the formation of hyphae that penetrate the wall of epidermal cells and the formation of a septum at the entry point (Perez-Nadales and Di Pietro, 2011). F. oxysporum hyphae were observed, in longitudinal cuts of roots at 15 DAI, which means that the fungus was able to overcome the defenses of the plant, invading and growing within the root across the inter- and intracellular spaces, and reaching the xylem vases using the holes generated by the fungus. At 30 DAI, the fungus completely colonized the inside of the root, this is, as the mycelium grows, it expands and produces microconidia, which are carried upward within the xylem vessels by way of a stream of raw sap (mineral salts and water) (Figure 1). When the fungus invades and proliferates within the plant roots, the conductive vessels are obstructed causing a water imbalance and the leaves start to develop yellowing symptoms, followed by wilt (Wang et al., 2015). Under optimal conditions, F. oxysporum requires 30 days to complete its infection process in A. americana roots.

Figure 1. Scanning electron microscopy photographs of Agave americana roots infected with Fusarium oxysporum A) Root surface at 7 DAI, B) Longitudinal cut of root at 15 DAI, C) Longitudinal cut of root at 30 DAI. C: Conidia, H: Hyphae.
Before this study there were no reports about the use of scanning electron microscopy to confirm that A. americana wilt in Agave species is caused by F. oxysporum. However, similar studies have been conducted for different crops such as serrano type CM334 criollo chili (Capsicum annum), where the advance of necrosis was determined at 3, 23 and 30 days after infection caused by the fungus, which advanced up to the plant stem. The symptoms reported were the presence of mycelium, microconidia, and macroconidia in all the evaluated segments (Sanzón et al., 2012). In chickpea crops (Cicer arietinum), F. oxysporum colonized the inside of the root in only three days (Joshi et al., 2012). Although the symptomatology observed on A. americana plants is attributed to F. oxysporum, more thorough analyses must be conducted since López-Bautista et al. (2020), recently demonstrated that wilt and/or dry rot symptoms in Agave tequilana are associated with five Fusarium species, which through a phylogenetic study were classified in three complexes: F. oxysporum (FOSC), F. solani (FSSC) and F. fujikuroi (FFSC), being F. oxysporum (FOSC) the predominant complex with 56% of representation at the regional level. Therefore, the agave wilt and dry bud rot symptoms are considered as a syndrome caused by different Fusarium species with differential parasitic suitability at the intra- and interspecies level (López-Bautista et al., 2020).
The specific activity of the chitinase during the period analyzed showed a significant increase (63.43 µmol min-1µg protein) at 15 DAI compared to those of the non-infected plants (27.24 µmol min-1µg protein). The increase of the activity could be explained by the fact that some PRs proteins are expressed at basal levels, but their expression increases in response to a pathogen attack and the subsequent activation of the systematic acquired resistance (SAR) (Durrant and Dong, 2004). Furthermore, the induction of PRs proteins is a way to control the pathogen from entering and spreading within the plant (Gupta et al., 2013). In the presence of phytopathogenic fungi, plants produce enzymes such as β-1,3-glucanase and chitinase (Santos et al., 2004) to disintegrate the components of the fungi cell wall up to their basic structural units, glucans, and chitin. A significant statistical difference was observed on the chitinase activity at 30 DAI in the infected and non-infected plants (Figure 2) with lower activity on the infected plants (21.1 µmol min-1µg protein). On the other hand, the activity of β-1,3 glucanase was lower on the infected plants (30.484 µmol min-1µg protein) than on the non-infected plants (32.167 µmol min-1µg protein). However, at 15 DAI, there was a slight increase of activity on the infected plants (Figure 3).
The increase in chitinase and β-1,3-glucanase activity induced by different fungi has been reported in different plant species such as tomato (Solanum lycopersicum), melon (Cucumis melo) and lemon (Citrus x limon) (Ramammoorthy et al., 2002; Baldé et al., 2006; Fanta et al., 2003). Our results suggest that the activity of chitinase and β-1,3 glucanase on A. americana plants acts as a defense mechanism against infection by F. oxysporum at 15 DAI. However, increasing the activity of the PRs proteins activity is probably not enough to keep the fungus from colonizing the roots, as shown in Figure 1.
The scanning electron microscopy analysis confirmed the infection caused by F. oxysporum in A. americana roots at 15 DAI. A molecular analysis confirmed the identity of the fungus. These results suggest that in A. americana plants, the induction of the defense mechanism is mediated by the activation of chitinase, which is involved in the plant defense during F. oxysporum infection. However, the increment of this enzyme activity is not enough to avoid the fungus colonization in roots of these species. This study provides the first insights into the enzyme activity of chitinase and β-1,3-glucanase as possible defense mechanisms in Agave americana.
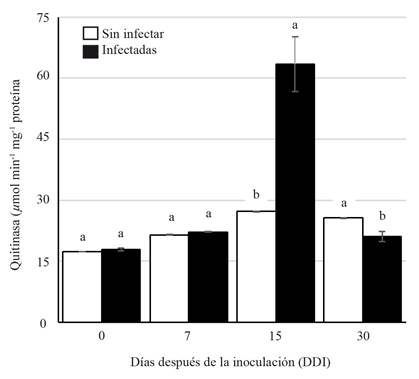
Figure 2. Chitinase activity (PR3) on Agave americana roots during Fusarium oxysporum infection. Different letters indicate statistically significant differences (ANOVA) p≤0.05.
Literatura Citada
Baldé, JA., Francisco, R., Queiroz, A., Regalado, AP., Ricardo, CP. and Veloso, MM. 2006. Immunolocalization of a class III chitinase in two muskmelon cultivars reacting differently to Fusarium oxysporum f. sp. melonis. Journal of Plant Physiology 163(1): 19-25. https://doi.org/10.1016/j.jplph.2005.02.004 [ Links ]
Bradford, MN. 1976. A rapid and sensitive method for the quantitation of microgram quantity of protein utilizing the principle of protein-dye binding. Analytical Biochemistry 72: 248-254. https://doi.org/10.1016/0003-2697(76)90527-3 [ Links ]
Czymmek, KJ., Fogg, M., Powell, DH., Sweigard, J., Park, SY. and Kang, S. 2007. In vivo time-lapse documentation using confocal and multi-photon microscopy reveals the mechanisms of invasion into the Arabidopsis root vascular system by Fusarium oxysporum. Fungal Genetics and Biology 44: 1011-1023. https://doi.org/10.1016/j.fgb.2007.01.012 [ Links ]
Durrant, WE. and Dong, X. 2004. Systemic acquired resistance. Annual Review of Phytopathology 42:(1) 185-209. https://doi.org/10.1146/annurev.phyto.42.040803.140421 [ Links ]
Fanta, N., Ortega, X. and Pérez, LM. 2003. The development of Alternaria alternata is prevented by chitinases and b-1,3-glucanases from Citrus limon seedlings. Biological Research 36: 411-420. http://dx.doi.org/10.4067/S0716-97602003000300012 [ Links ]
Ferrari, AR., Gaber, Y. and Fraaije, MW. 2014. A fast, sensitive and easy colorimetric assay for chitinase and cellulase activity detection. Biotechnology for Biofuels 7(1): 37. https://doi.org/10.1186/1754-6834-7-37 [ Links ]
Flores, LHE., Ireta, JM., Pérez, DJF., Ruíz, CJA., Álvarez, MC. and Byerly, KFM. 2009. Identificación de zonas de riesgo fitopatológico y opciones de prevención y/o control en el Agave tequilana weber variedad azul en Jalisco. Informe de investigación. INIFAP. CIRPAC. CECEAJAL. Tepatitlán de Morelos, Jalisco. 34 p. [ Links ]
Gupta, P., Ravi, I. and Sharma, V. 2013. Induction of β-1,3-glucanase and chitinase activity in the defense response of Eruca sativa plants against the fungal pathogen Alternaria brassicicola. Journal of Plant Interactions 8(2): 155-161. https://doi.org/10.1080/17429145.2012.679705 [ Links ]
Honorato, JJ., Zambolim, L., Aucique-Pérez, CE., Resende, RS. and Rodrigues, FA. 2015. Photosynthetic and antioxidative alterations in coffee leaves caused by epoxiconazole and pyraclostrobin sprays and Hemileia vastatrix infection. Pesticide Biochemistry and Physiology 123: 31-39. https://doi.org/10.1016/j.pestbp.2015.01.016 [ Links ]
Joshi, NS., Rao, KS. and Subramanian, RB. 2012. Anatomical and biochemical aspects of interaction between roots of chickpea and Fusarium oxysporum f. sp. ciceris race 2. Archives of Phytopathology and Plant Protection 45(15): 1773-1789. https://doi.org/10.1080/03235408.2012.674709 [ Links ]
Leslie, JF., Summerell, BA. 2006. The Fusarium Laboratory Manual. Hoboken, Blackwell Publishing. https://doi.org/10.1002/9780470278376.fmatter [ Links ]
López-Bautista, V., Mora-Aguilera, G., Gutiérrez-Espino sa, MA., Mendoza-Ramos, C., Martínez-Bustamante, VI., Coria-Contreras, JJ., Acevedo-Sánchez, G. and Santana-Peñaloza, B. 2020. Morphological and molecular cha racterization of Fusarium spp. associated to the regional occurrence of wilt and dry bud rot in Agave tequilana. Mexican Journal of Phytopathology 38(1): 79-106. https://doi.org/10.18781/R.MEX.FIT.1911-4 [ Links ]
Mahendranathan, C., Adikaram, NKB. and Jayasingam, T. 2016. Enhancement of natural disease resistance of Capsicum annuum L. against anthracnose disease through selected postharvest treatments. Proceedings of the 9th Australasian Soil-borne Symposium, Lincoln University, New Zealand. 14-17 [ Links ]
Padilla-Ramos, R., Salas-Muñoz, S., Velásquez-Valle, R. and Reveles-Torres, LR. 2018. A novel molecular approach in the study of parasite-host interaction. Revista Mexicana de Fitopatología 37(1): 95-114. https://doi.org/10.18781/R.MEX.FIT.1808-6 [ Links ]
Pan, SQ., Ye, XS. and Kue, J. 1991. A technique for detection of chitinase, β-1,3-glucanase, and protein patterns after a single separation using polyacrylamide gel electrophoresis or isoelectrofocusing. Phytopathology 81(9): 970-974. https://doi.org/10.1094/phyto-81-970 [ Links ]
Perez-Nadales, E. and Di Pietro, A. 2011. The membrane mucin Msb2 regulates invasive growth and plant infection in Fusarium oxysporum. The Plant Cell 23(3):1171-1185. https://doi.org/10.1105/tpc.110.07509 [ Links ]
Prusky, D., Alkan, N., Mengiste, T. and Fluhr, R. 2013. Quiescent and necrotrophic lifestyle choice during postharvest disease development. Annual Review of Phytopathology 51:155-176. https://doi.org/10.1146/annurev-phyto-082712-102349 [ Links ]
Ramammoorthy, V., Raguchander, T. and Samiyappan, R. 2002. Induction of defense-related proteins in tomato roots treated with Pseudomonas fluorescens Pf1 and Fusarium oxysporum f. sp. lycopersici. Plant Soil 239(1):55-68. https://www.jstor.org/stable/24120999 [ Links ]
Ruíz-May, E., De la Peña, C., Galaz-Avalos, RM., Lei, Z., Watson, BS., Sumner, LW. and Loyola-Vargas, VM. 2011. ATP biosynthesis deficiency revealed by proteomics approach is correlated with oxidative burst in Catharanthus roseus (L.) G. hairy roots treated with methyl jasmonate. Plant and Cell Physiology 52:1401-1421. https://doi.org/10.1093/pcp/pcr086 [ Links ]
Santos, IS., Machado, OLT., Da Cunha, M. and Gomes, VM. 2004. A chitinase from Adenanthera pavonina L. seeds: purification, characterization and immunolocalization. Plant Science 167(6):1203-1210. https://doi.org/10.1016/j.plantsci.2004.04.021 [ Links ]
Sanzón, GD., Valdovinos, PG., Rojas, MRI., Zavaleta, ME., Mora, AMA. and Guevara, OL. 2012. Cambios morfológicos en células de chile CM334 inoculado con Phytophthora capsici y con Fusarium oxysporum. Revista Mexicana de Fitopatología 30: 66-71 [ Links ]
Statgraphics Centurion XVI.II. 2010. StatPoint Technologies, Inc. [ Links ]
Wang, M., Sun, Y., Sun, G., Liu, X., Zhai, L., Shen, Q. and Guo, S. 2015. Water balance altered in cucumber plants infected with Fusarium oxysporum f. sp. Cucumerinum. Scientific Reports 5:7722. https://doi.org/10.1038/srep07722 [ Links ]
White, TJ., Bruns, T., Lee, S. and Taylor, J. 1990. Amplification and direct sequencing of fungal ribosomal RNA genes for phylogenetics. In: PCR protocols: A guide to methods and applications Gelfand, DH., Sninsky, JJ., White, TJ. and Innis, MA. (Eds). Academic Press. http://dx.doi.org/10.1016/b978-0-12-372180-8.50042-1 [ Links ]
Received: May 17, 2020; Accepted: July 26, 2020