Servicios Personalizados
Revista
Articulo
Indicadores
-
Citado por SciELO
-
Accesos
Links relacionados
-
Similares en SciELO
Compartir
Revista mexicana de fitopatología
versión On-line ISSN 2007-8080versión impresa ISSN 0185-3309
Rev. mex. fitopatol vol.37 no.2 Texcoco may. 2019 Epub 30-Sep-2020
https://doi.org/10.18781/r.mex.fit.1812-1
Phytopathological notes
Inhibition of Colletotrichum gloeosporioides and Botrytis cinerea by Guazuma ulmifolia Lam extracts
1estudiante del doctorado BEMARENA, CUCBA, Universidad de Guadalajara, México;
2Departamento de Madera, Celulosa y Papel, Cucei, Universidad de Gudalajara, Zapopan Jalisco México, CP 45010
3 Departamento de Botánica, CUCBA, Universidad de Guadalajara, Zapopan Jalisco México, CP 45010
4 Laboratorio de Fitopatología, CUCBA, Universidad de Guadalajara, Zapopan Jalisco México, CP 45010.
Guazuma ulmifolia (Malvaceae) is a tree used in the popular medicine of many countries to treat various diseases. We evaluated the inhibitory activity of G ulmifolia bark and fruits extracts, obtained with solvents of increasing polarity, against Colletotrichum gloeosporioides and Botrytis cinerea. Thin-layer chromatography assay (TLC) and extract yield were the criteria used to establish the most efficient extraction solvent. Extracts were screened for their phytochemical components and their corresponding antifungal effect by thin-layer chromatography-Bioautography and Mycelial Growth Inhibition methods in a randomized design. Fruit extracts were more inhibitory than bark extracts, while C. gloeosporioides was more resistant than B. cinerea. At a dose of 10 mg·mL-1; fruit extracts inhibited 85.5 ± 2.1% of B. cinerea and 45.8 ± 10.0% of C. gloeosporioides mycelia growth. Bark extracts inhibited 58.0 ± 2.4% and 34.1 ± 2.9% of B. cinerea and C. gloeosporioides mycelial growth, respectively. Images obtained with light microscopy showed that extracts altered the original mycelia and spore morphology.
Key words: Procyanidins; mycelium; DPPH radical; Chromatography TLC
Guazuma ulmifolia (Malvaceae) es un árbol utilizado en la medicina popular de múltiples países para tratar diversas enfermedades. Se evaluó el efecto inhibitorio de los extractos de la corteza y fruto de esta planta, obtenidos con solventes de polaridad creciente, contra Colletotrichum gloeosporioides y Botrytis cinerea. Se utilizó como criterio para elegir el solvente con mejor desempeño en la extracción, ensayos de cromatografía de capa fina (TLC) y el dato del rendimiento de extractos. Posteriormente, se analizaron los extractos por medio de tamizaje fitoquímico y se evaluó la actividad antifúngica con los métodos de TLC-Bioautografia e inhibición de crecimiento micelial, aplicando un diseño experimental aleatorizado. Los extractos del fruto fueron más efectivos que los extractos de corteza, y por su parte, C. gloeosporioides fue más resistente que B. cinerea. A 10 mg mL-1, los extractos de fruto inhibieron 85.5 ± 2.1% el crecimiento micelial de B. cinerea y en 45.8 ± 10.0% el de C. gloeosporioides, mientras que los extractos de corteza inhibieron en 58.0 ± 2.4% y 34.1 ± 2.9% el crecimiento de B. cinerea y C. gloeosporioides, respectivamente. Imágenes obtenidas microscopia óptica muestran que los extractos alteraron la morfología original tanto de los micelios como de las esporas.
Palabras clave: Procianidinas; micelio; radical DPPH; cromatografía TLC
Fungal diseases are responsible for considerable losses in agricultural production. Botrytis cinerea, which causes gray rot (gray mold), is a phytopathogen that can infect about 1,000 known plant species via leaves, petioles, roots, fruits, flowers, seeds, as well as postharvest fruits (Rupp et al., 2017). Colletotrichum gloeosporioides, of the family Ascomycetes, is a fungal disease that causes anthracnose in various parts of the plant and fruit. These fungi are one of the most relevant postharvest pathogens since its disease results in long quiescent or latent asymptomatic fruit infections with symptoms appearing during fruit ripening (Rodríguez-López et al., 2009) when the conidia respond to chemical signals, cuticle waxes and ethylene, produced by the host. Many methods have been tried to prevent and control phytopathogens, from the traditional use of chemicals, the development of renewable natural products with unique properties, including biocompatibility, biodegradability, antimicrobial activity, and safety, to the application of essential oils and plant extracts with microbicidal activity (Zhong et al., 2014). Guazuma ulmifolia (Malvaceae), commonly known as guacimo, mutamba, caulote or equiche, is a tropical tree of medium size distributed from northern Mexico to South America (Maurino dos Santos et al., 2018). For their antibacterial, antifungal, and anti-inflammatory properties, the bark, fruits, and leaves are traditionally used to treat various diseases (Maldini et al., 2013). In addition, the foliage and fruit are used as livestock and horses forage. Although bark, leaves and fruit extracts have antimicrobial properties, most scientific reports have focused on the bark and its medicinal properties, which are attributed to its high procyanidins content (condensed tannins) (Lopes et al., 2009); however, there are few reports on the use of G. ulmifolia extracts to control phytopathogens. Restrictions on the use of agrochemicals for the control of pests has led to the development of biological control methods, including biodegradable plant extracts with fungicidal properties. Therefore, the objective of this study was to evaluate the antimicrobial activity of G. ulmifolia fruit and bark extracts against B. cinerea and C. gloeosporioides.
Plant Collection. On April 2015, we collected bark and fruits from five randomly selected wild trees growing in the area known as “Bosque de la Primavera” in the town of Tala Jalisco (20° 29’ 00’’ N latitude and 103° 29’ 30’’ W longitude) at an altitude of 1320 meters above average sea level. From each tree, approximately 3 kg of mature fruits and 1 kg of bark from different parts of the stem, without evidence of diseases, were collected. A representative composite aliquot drawn from the original sample was dried at ~25 °C (in the laboratory), pulverized in a knife mill (Retsch-GMBH), and kept refrigerated until used. “Bosque de la Primavera” is a 30500 ha protected natural area located west of the metropolitan area of Guadalajara, Mexico comprised of oak, oak-pine, and tropical deciduous forest. The average annual rainfall fluctuates between 800 and 1000 mm, while the yearly average temperature is 20.6 ± 6.5 °C (Huerta-Martínez and Ibarra-Montoya, 2014).
Crude Extracts Preparation. The dry milled materials (100 g dry weight) were extracted at 4 °C with 500 mL of hexane, to remove the lipid material (lipids can interfere with the metabolites assays), for 2 hours with constant stirring in a flat bottom flask. Preliminary tests were carried out with solvents of different polarities (hexane, dichloromethane, and methanol) to find the most efficient extracting solvent as determined by a thin layer chromatography (TLC) test. The results of this screening indicated that dichloromethane was the best extracting solvent. Fruit and bark samples were extracted with dichloromethane for 48 h at 28 °C with continuous stirring (150 rpm) and a solution to dry sample ratio of 5:1 (v/w) in an incubator (LabTech model LSI-3016R). The slurry was filtered through Whatman paper No 4 and the solvent, containing the extracted material, concentrated until dryness in a rotary evaporator Buchi under reduced pressure and 40 °C. We used the total solids test to determined the extract yield and expressed as milligrams of dry solids in the solvent extract per gram of original dry plant material.
Pathogens Culture and Inoculum Preparation. CUCBA Phytopathology Laboratory of the University of Guadalajara kindly provided the C. gloeosporioides and B. cinerea strains isolated from diseased papaya and strawberry, respectively, and identified by morphology and physiological characteristics Pure cultures of these strains were transferred into separate glass tubes and dispersed in 5 mL of sterile saline solution (NaCl, 0.85% w/v) or distilled water, and the microorganisms concentration was adjusted with saline solution or water to 1 - 2.5 x 106 cells mL-1, using for this a Neubauer cell-counting chamber (Moro et al., 2017).
Phytochemical Screening. Before the bioassay tests, the biological activity of the phytochemical compounds (e.g., flavonoids, tannins, terpenoids, alkaloids, saponins, glycosides, and so on) found in the extracts were qualitatively identified using established procedures (Harbone, 1998).
TLC-Bioautography (TLC-B). TLC-B methods are those in which biological tests (antimicrobial or enzymatic) are applied on chemical extracts for the qualitative detection of bioactive compounds (Choma and Jesionek 2015). In our work, plant extracts were separated into their phytochemical constituents by TLC. For this, ~10 μL of extract was applied on a TLC plate (10 x10 cm, silica gel, Merk, 60F254) and developed with toluene:ethyl acetate (85:15) mobile phase. After this, the phytochemicals were visualized with UV light (254 and 365 nm, Handfield UV Lamp, UVGL-58) and their Rf value (Retardation factor) recorded. We then used the developed plates to assess the compounds antimicrobial activity by direct bioautography using a green tea extract as a positive control. The dried plates were sprayed with the corresponding fungal suspension (1 x106 cells mL-1) until moist, incubated for 24 h at 37 °C, and then impregnated with p-iodonitrotetrazolium violet (Sigma, 2 mg mL-1, 6 h, 35 °C). Tetrazolium salt (INT) is an electron acceptor which the biologically active microorganisms reduce it to red-colored formazan becoming colorless when the fungi growth is inhibited (Eloff et al., 2008). Red-purple areas reveal active microbes and white areas indicate inhibition of fungal activity. To assess the antioxidant activity, we sprayed the TLC plates with a 0.2 % solution of the free radical compound DPPH (2, 2, diphenyl-1-picrylhydrazyl in methanol, Sigma®). After heating the TLC plates, the spots (compounds) with antioxidant activity turned to a yellowish white color over the DPPH purple background (Belaqziz et al., 2017).
Mycelial Growth Inhibition (MGI). Growth agar medium was prepared by blending (using a DragonLab homogenizer) liquid PDA agar with 1% (v/v), Tween 80 (surfactant to improve emulsification) and enough extract to achieve concentrations in the 1-20 mg mL-1 range (this range is wide enough to detect inhibitory activity). Enough agar was deposited on Petri dishes (90 mmØ) and allowed to solidify; then a 7 mm diameter disc, impregnated with a fungus conidiospores solution containing 1 x106 cells mL-1, was placed at the plates’ center. PDA and benomyl (1 mg mL-1) served as negative and positive controls, respectively. Benomyl [1-[(butylamino)carbonyl]- 1H-benzimidazol-2-yl], is an azole derivative commonly used for the chemical control of these fungi. The dishes were incubated at 28 °C and the colony growth measured every 24 h, with the aid of a ruler, until the mycelium in the negative control reached the dish’s edge. Tests were done in triplicate, and the percent mycelial inhibition calculated with the following formula (Zhong et al., 2014):
The lethal concentrations, (LC50) and (LC95), were calculated from the curve of mycelial inhibition vs. extract concentration.
Microscopy study. Samples of fungi treated with bark and fruit guacimo extracts (10 mg mL-1), benomyl, and PDA were deposited on microscope slides and homogeneously dispersed with distilled water and viewed in an optical microscope (Zeiss model KF2) equipped with a camera (Axio Cam ERC5S).
Statistical Analyses. A randomized experimental design was set up with the factors: Plant part (Fruit and Bark); fungi (C. gloeosporioides and B. cinerea); treatment time (72 h, 144 h, 240 h, 312 h), and extract concentration (1 mg mL-1, 10 mg mL-1, 20 mg mL-1). The response variable radial was growth inhibition (%). Data was analyzed by multifactorial ANOVA (95.0% confidence level, P < 0.05) to determine the statistical significance factors’ effect and Tukey’s test to establish the level of significance among values. We used the statistical software Statgraphics Centurion XVII (©2014 Statpoint Technologies, Inc.) for data analysis.
In results, the fruit extract yield in mg·g-1 dry weight (dw) for the different solvents were: 20.1 for hexane, 52.5 for dichloromethane, and 76.4 for methanol while those for the bark extract were 56.7 for hexane, 122.4 for dichloromethane, and 66.0 for methanol. These values are higher than the 2 mg g-1, and 47 mg g-1reported for macerated guacimo bark with hexane and methanol, respectively (Camporese et al., 2003). Dichloromethane was the most efficient solvent as indicated by the number of metabolites found in the TLC test. The screening attested the presence of alkaloids, flavonoids, tannins, triterpenes, saponins, and coumarins in the extracts, highlighting the presence of flavonoids, triterpenes, and coumarins, due to their biological activity. Our results agree with those of Singanan et al. (2018) who reported the presence of phenols, tannins, saponins, flavonoids, and terpenoids in G. ulmifolia fruit. Some of these biomolecules are characteristic of medicinal plants and have antioxidant, therapeutic, and medicinal properties that can inhibit or eliminate the growth of pathogens, with little or no toxicity to the host cells, and are good candidates for antimicrobial applications (Mujeeb et al., 2014). The TLC- Bioautography results for B. cinerea are included in Figure 1. Results for C. gloeosporioides and B. cinerea were similar, and for the sake of brevity, we only discuss those of the latter. TLC plate A in Figure 1 shows the compounds present in guacimo bark and fruit extracts, shown as distinct round dots. Plate (B) shows the antioxidant activity of each of the spot found in Plate (A) against DPPH radical (TLC-DPPH); on this plate, a yellowish spot indicates antioxidant activity. Finally, plates C and D depict G. ulmifolia extracts and green tea, used as positive control, antimicrobial activity against B. cinerea (TLC-direct bioautography test), respectively. Clear spots indicate antimicrobial activity. As can be seen, the active zones found in plate C (G. ulmifolia extract) coincide with those of green tea, suggesting that both contain similar flavonoids and that this zone comprises the extracts antioxidant and antifungal activity. The Rf values for these spots are approximately 0.30-0.45. The size and intensity of the spots indicate the bioactivity level. A close examination of the TLC plates would show that the fruit extract bioactivity is higher than that of the bark extract.
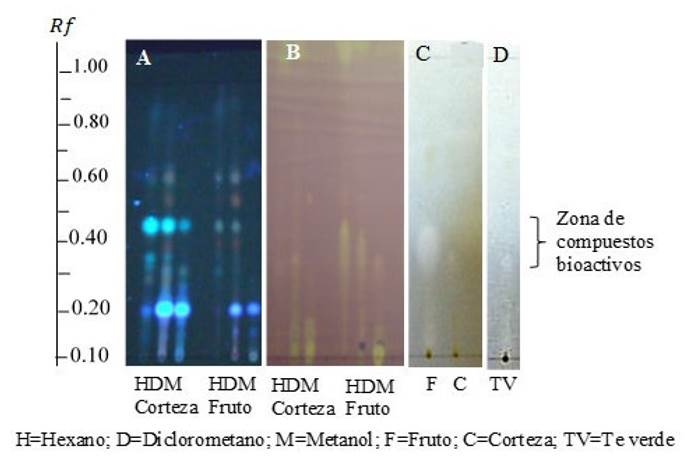
Figure 1 Qualitative biological tests for crude extracts of G. ulmifolia’s bark and fruit against B. Cinerea. Mobile phase Toluene/Ethyl acetate (85/15). A) Silica gel TLC; B) TLC-DPPH. Antioxidant activity of G. ulmifolia extracts. De tection method: Vanillin; C) TLC-Direct Bioautography. Antifungal activity of G. ulmifolia extracts and D) Green tea- Direct Bioautography. Antifungal activity of green tea extracts (Control).
Table 1 contains the results of the mycelial growth inhibition assay (MGI). All experimental variables were statistically significant (ANOVA, P<0.05). G. ulmifolia bark and fruit crude extracts inhibited the growth of B. cinerea and C. gloeosporioides to different extents. The highest inhibition (91.5 ± 1.4%) was observed on C. gloeosporioides with 20 mg mL-1 of fruit extract and 13 days after treatment, similar to Benomyl (1 mg mL-1) effect in this point. This indicates that a considerable amount of guacimo crude extract would be needed to exert the same effect as Benomyl or other chemical fungicides.
However, the use of plant extracts would have some benefits such as being an innocuous biological control, among others. In contrast, the bark extracts had a less inhibitory effect than the fruit extracts. Under the same testing conditions (13 days, 20 mg mL-1) its inhibitory activity against C. gloeosporioides was 48.0 ± 1.6% which is almost 50% lower than the 91.5% that was obtained with fruit. B. cinerea was more susceptible to the extracts than C. gloeosporioides (more resilient). For example, after 13 days of treatment and at a dose of 10 mg mL-1, the fruit extracts inhibited the growth of B. cinerea and C. gloeosporioides by 85.5 ± 2.1 % and 45.8 ± 10.0%, respectively, which is indicative of this trend. Results of this study indicate that guacimo extracts repressed fungal mycelial growth with best results at concentrations ³ 10 mg mL-1 and maximum inhibition at 20 mg mL-1; however, at 1 mg·mL-1the inhibition was weak and erratic. Although there are no previous works on the effect of G. ulmifolia crude extracts against these two pathogens other investigations have demonstrated that other plant extracts inhibit their growth. A methanol extract from Piscidia piscipula applied at 2 mg mL-1 resulted in a 52.3% C. gloeosporioides mycelial growth inhibition after five days (Peraza-Sanchez et al., 2005) which is higher than our results. In addition, the hydroalcoholic crude extracts of the soapberry (Sapindus saponaria) leaf 7 days after treatment resulted in a 70% C. gloeosporioides MGI when applied to 50 mg mL-1 and 80% MGI when applied at100 mg·mL-1, while concentrations lower than 50 mg mL-1 had no effect (Passos Marinho et al., 2018). Alcoholic extracts of wild grapevines (Vitis) leaves had a lower inhibitory effect than G. ulmifolia extracts. When applied at 12% wild grapevines (Vitis) leaves resulted in only 70% B. cinerea MGI (Apolonio-Rodríguez et al., 2017). The antimicrobial and medicinal activity of G. ulmifolia bark, aerial part, and fruits extracts are attributed to the presence of flavonoids of the subgroup flavan-3-ols (Lopes et al., 2009) with antioxidant and anti-inflammatory properties including catechin, epicatechin, flavanocoumarins, as the procyanidins and proanthocyanidins derived from them (Maldini et al., 2013). The Lethal Concentration (LC, mg mL-1) parameters calculated from the best inhibitory values shown in Table 1, are as follows: 1. C. gloeosporioides: Fruit (LC50 = 10.57, LC95 = 20.94); Bark (LC50 = 16.54, LC95 = 34.13), 2. B. cinerea: Fruit (LC50 = 5.24, LC9 5= 11.09); Bark (LC50 = 10.6, LC95 = 22.6). Results of the microscopy study are shown in Figure 2. Anatomy of C gloeosporioides and B. cinerea has been previously reported. Figure 2A shows of C gloeosporioides. Its main features (Rodríguez-López et al., 2009) are light gray to dark gray septate hyphae. Conidia are hyaline, cylindrical, and separated from the pedicel by a transverse septum. Also for B. cinerea (Figure 2B), it is established (Miclea Raluca Vasilica et al., 2012) septate and cylindrical hyphae, hyaline at the beginning and later become brown or black, which multiply by cytoplasmic division. Conidia are globose or elliptical, forming exogenous chains (Blastospores). Fungi treatment with guacimo extracts altered its morphology, including distorted, wavy, folded mycelia, with flat or empty portions (Xu et al., 2007); cytoplasmic coagulation exhibited by small vesicles in the mycelium (Figure 2C). Nonpolar phenolic compounds, such as those found in guacimo, could affect the cytoplasmic membrane, causing its disintegration loss of adenosine triphosphate and cell death (Hassani et al., 2012). The conidia showed changes in their original form. (Figure 2E). The conidias’ cell wall can also experience damage with numerous protrusions that eventually leads to their break down and inhibition of their germination (Sharma and Tripathi, 2008). In the same way, C. gloeosporioides treated with Benomyl (benzimidazole) showed empty hyphae with large anomalous structures (Figure 2F). Benomyl is a commercial fungicide that has been used for many years to control anthracnose before fruits harvesting.
Table 1 Inhibition of B. cinerea and C.
gloeosporioides mycelial growth by G.
ulmifolia fruit and bark extracts.
Values represent the
Planta parte | Hongo | CE, | Tiempo de Tratamiento, días | |||
---|---|---|---|---|---|---|
mg mL-1 | 3 | 6 | 10 | 13 | ||
Inhibición de Crecimiento Micelial, % | ||||||
Fruto | Cg | 1 | 10.0±0.0a | 9.5±2.1a | 16.5±3.1a | 13.7±2.6a |
10 | 13.3±4.7a | 17.8±1.6ab | 37.2±3.3b | 45.8±10.0bc | ||
20 | 60.0±0.0c | 83.5±4.4cd | 90.7±0.2d | 91.5±1.4d | ||
Benomilx | 80.0±0.0cd | 85.7±3.8cd | 90.6±2.2d | 91.5±1.7d | ||
Bc | 1 | 36.5±12.3bc | 6.6±20.3a | 31.0±15.6bc | 28.0±18.7ab | |
10 | 44.8±5.4bc | 39.4±17.6b | 75.9±5.0cd | 85.5±2.1cd | ||
Benomil | 80.0±0.0cd | 87.6±3.8d | 88.4±2.1d | 91.5±1.6d | ||
Corteza | Cg | 1 | 20.0±4.1ab | 12.4±3.4a | 18.1±2.4ab | 16.6±1.6ab |
10 | 30.0±8.2b | 34.2±1.5b | 44.2±1.3bc | 34.1±2.9b | ||
20 | 60.0±0.0c | 50.7±1.1bc | 42.6±3.0bc | 48.0±1.6bc | ||
Benomil | 83.33±0.0cd | 87.6±3.8d | 89.4±2.2d | 91.5±1.7d | ||
Bc | 1 | 19.2±1.5ab | 18.6±1.2ab | 20.4±1.6ab | 15.5±2.3ab | |
10 | 58.5±1.8c | 57.3±1.7c | 59.4±2.3c | 58.0±2.4c | ||
20 | 79.3±0.9cd | 76.2±1.9cd | 75.4±2.5cd | 78.4±3.2cd | ||
Benomil | 94.8±0.0d | 94.7±0.5d | 94.8±1.4d | 94.6±2.5d |
CE=Concentración de Extractos; Cg= C. gloeosporioides; Bc= B. cinerea; x concentración Benomilo =1 mg mL-1 / CE = Concentration Extracts; Cg = C. gloeosporioides; Bc= B. cinerea; xBenomyl concentration = 1 mg mL-1
Los valores en la misma columna con letras diferentes indica que son significativamente diferentes, de acuerdo con la prueba de Tukey (P<0.05) / Values in the same column with different letters are significantly different according to Tukey´s test (P < 0.05).
In this study, the fruit extract was more effective on mycelial inhibition than the bark extract and could be a good source of biologically active compounds with therapeutic and fungicidal activity. Although the fruit is the most accessible part of the plant, which is edible, sweet, palatable, and consumed by the general population has not been extensively investigated. C. gloeosporioides was more resistant than B. cinerea; however, more specific tests and evaluation of pathogenicity and virulence are needed to confirm these results.
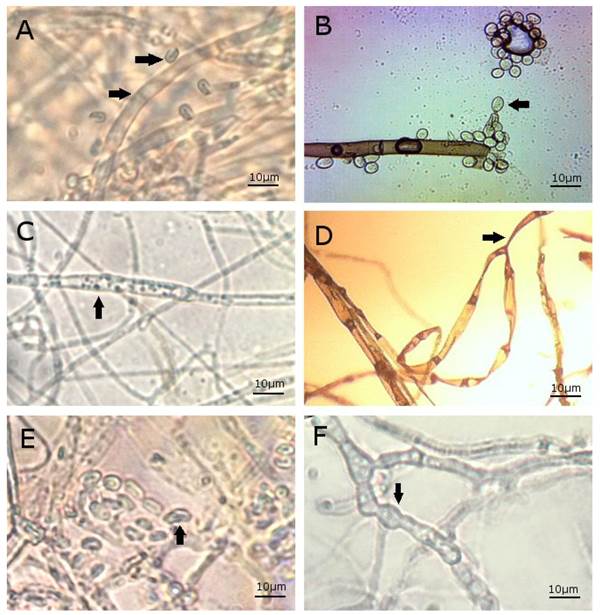
Figure 2 Microscopic changes observed in B. cinerea and C. gloeosporioides after exposure to G. ulmifolia fruit and bark extracts. Light microscope, 100x. A) C. gloeosporioides morphology; B) B. cinereal morphology; C) C. gloeospori oides treated with G. ulmifolia fruit extract: Observe hyphae with deformed structure and coagulated cytoplasm; D) B. cinerea treated with G. ulmifolia fruit extract; E) C. gloeosporioides treated with G. ulmifolia bark extract andF) C. gloeosporioides treated with Benomyl.
Acknowledgments
We like to thank the National Council for Science and Technology (CONACYT), by awarding scholarship no. 388048/256531, the BEMARENA-CUCBA doctorate program and the Department of Wood Cellulose and Paper (DMCyP)-CUCEI of the University of Guadalajara for the support provided for this work.
REFERENCES
Apolonio-Rodríguez I, Franco-Mora O and Salgado-Siclán ML. 2017. Inhibición in vitro de Botrytis cinerea con extractos de hojas de vid silvestre (Vitis spp). Revista Mexicana de Fitopatología 35: 170-185. https://doi.org/10.18781/R.MEX.FIT.1611-1 [ Links ]
Belaqziz M, Tan SP, El-Abbassi A, Kiai H, Hafidi A, O’Donovan O and McLoughlin P. 2017. Assessment of the antioxidant and antibacterial activities of different olive processing wastewaters. PLoS ONE 12(9): e0182622. https://doi.org/10.1371/journal.pone.0182622 [ Links ]
Camporese A, Balick M, Arvigo R, Esposito RG, Morsellino N, Simone FD and Tubaro A. 2003. Screening of anti-bacterial activity of medicinal plants from Belize (Central America). Journal of Ethnopharmacology 87:103-107. https://doi.org/10.1016/S0378-8741(03)00115-6 [ Links ]
Choma I and Jesionek W. 2015. TLC-Direct bioautography as a high throughput method for detection of antimicrobials in plants. Chromatography 2:225-238. https://doi.org/ 10.3390/chromatography2020225 [ Links ]
Eloff JN, Katherine DR and Mcgaw LJ. 2008. The Biological activity and chemistry of the South African Combretaceae. Journal of Ethnopharmacology 1119:686-699.https://doi.org/10.1016/j.jep.2008.07.051 [ Links ]
Harborne JB. 1998. Phytochemical Methods; A guide to modern techniques of plant analysis. Third edition. Chapman and Hall, London, 302 p. https://www.google.com.mx/url?sa=t&rct=j&q=&esrc=s&source=web&cd=6&ved=2ahUKEwjXoNCJn5jfAhUJPK0KHbZVBF8QFjAFegQIAxAC&url=https%3A%2F%2Ffaculty.psau.edu.sa%2Ffiledownload%2Fdoc-3-pdf-d71d9e49824fddceb67a26b34e5bc770-original.pdf&usg=AOvVaw0ZVzhs9rixocQh6KWFFFqk [ Links ]
Hassani A, Fathi Z, Ghosta Y, Abdollahi A, Meshkatalsadat MH and Marandi RJ. 2012. Evaluation of plant essential oils for control of postharvest brown and gray mold rots on Apricot. Journal of Food Safety 32:94-101. https://doi.org/10.1111/j.1745-4565.2011.00353.x [ Links ]
Huerta-Martínez FM and Ibarra-Montoya JL. 2014. Wildfires at la primavera forest, Jalisco, Mexico: an approach to possible causes and consequences. CienciaUAT. 9(1): 23-32. http://www.scielo.org.mx/scielo.php?script=sci_arttext&pid=S2007-78582014000100023 [ Links ]
Lopes GC, Rocha JCB, de Almeida GC and deMello JCP. 2009. Condensed tannins from the bark of Guazuma ulmifolia Lam. (Sterculiaceae). Journal of the Brazilian Chemical Society 20(6):1103-1109. http://dx.doi.org/10.1590/S0103-50532009000600016 [ Links ]
Maldini M, Di Micco S, Montoro P, Darra E, Mariotto S, Bifulco G, Pizza C and Piacente S. 2013. Flavanocoumarins from Guazuma ulmifolia bark and evaluation of their affinity for STAT1. Phytochemistry 86:64-71. https://doi.org/10.1016/j.phytochem.2012.10.011 [ Links ]
Maurino dos Santos J, Monteiro Alfredo T, Ávila Antunes K, da Silva Melo da Cunha J, Almeida Costa EM and Silva Lima E. 2018. Guazuma ulmifolia Lam. Decreases Oxidative Stress in Blood Volume 2018: 16 pages. https://doi.org/10.1155/2018/2935051 [ Links ]
Miclea Raluca V. Loredana Alexandra S and Carmen Emilia P. 2012. In vitro studies regarding the morphology of Botrytis Cinerea Pers. isolated from geranium Plants. ProEnvironment 5(2012): 60-66. https://www.researchgate.net/publication/268816084 [ Links ]
Moro IJ, Gabrielle Alexandre Gondo DG, Gonçalves Pierri E, Linharis Rodrigues Pietro RC, Pienna Soares C, Pergentino de Sousa D and Gonzaga dos Santos A. 2017. Evaluation of antimicrobial, cytotoxic and chemopreventive activities of carvone and its derivatives 53(4):e00076. http://dx.doi.org/10.1590/s2175-97902017000400076 [ Links ]
Mujeeb F, Bajpai P and Pathak N. 2014. Phytochemical evaluation, antimicrobial activity, and determination of bioactive components from leaves of Aegle marmelos. BioMed Research International Volume 2014, Article ID 497606, 11 pages. http://dx.doi.org/10.1155/2014/497606 [ Links ]
Passos Marinho GJ, Espellet Klein D and Siqueira Junior CL. 2018. Evaluation of soapberry (Sapindus saponaria L.) leaf extract against papaya anthracnose. Summa Phytopathology 44(2): 127-131. http://dx.doi.org/10.1590/0100-5405/175605 [ Links ]
Peraza-Sánchez SR, Chan-Che EO and Ruiz-Sánchez E. 2005. Screening of Yucatecan plant extracts to control Colletotrichum gloeosporioides and isolation of a new pimarene from Acacia pennatula. Journal of Agricultural and Food Chemistry 53:2429-2432. https://doi.org/10.1021/jf040422i [ Links ]
Rodríguez-López ES, González-Prieto JM and Mayek-Pérez N. 2009. Infection of avocado (Persea americana Mill.) by Colletotrichum gloeosporioides (Penz.) Penz. y Sacc.: Biochemical and genetics aspects. Revista Mexicana de Fitopatología 27:53-63. https://www.google.com.mx/url?sa=t&rct=j&q=&esrc=s&source=web&cd=1&cad=rja&uact=8&ved=2ahUKEwimj_nz05jfAhXMmq0KHWZpBVsQFjAAegQIChAB&url=http%3A%2F%2Fwww.scielo.org.mx%2Fscielo.php%3Fscript%3Dsci_arttext%26pid%3DS0185-009000100007&usg=AOvVaw2lvmZVIo7c0scZf7hpzViJ [ Links ]
Rupp S, Plesken C, Rumsey S, Dowling M, Schnabel G, Weber RWS and Hahna M. 2017. Botrytis fragariae, a new species causing gray mold on Strawberries, shows high frequencies of specific and efflux-Based fungicide resistance. Applied and Environmental Microbiology 83(9): 1-16. https://doi.org/10.1128/AEM.00269-17 [ Links ]
Sharma N and Tripathi A. 2008. Effects of Citrus sinensis (L.) Osbeck epicarp essential oil on growth and morphogenesis of Aspergillus niger (L.) Van Tieghem. Microbiological Research 163:337-344. https://doi.org/10.1016/j.micres.2006.06.009 [ Links ]
Singanan M, Duraiswamy B and Varadarajan V. 2018. Physicochemical, phytochemicals and antioxidant evaluation of Guazuma ulmifolia fruit. International Journal of Pharmacy and Pharmaceutical Sciences 10(9):87-91. http://dx.doi.org/10.22159/ijpps.2018v10i9.26778 [ Links ]
Xu WT, Huang KL, Guo F, Qu W, Yang JJ, Liang ZH and Luo YB. 2007. Postharvest grapefruit seed extract and chitosan treatments of table grapes to control Botrytis cinerea. Postharvest Biology and Technology 46:86-94. https://doi.org/10.1016/j.postharvbio.2007.03.019 [ Links ]
Zhong Z, Aotegen B, Xu H and Zhao, S. 2014. The influence of chemical structure on the antimicrobial activities of thiosemicarbazone-chitosan. Cellulose 21:105-114. https://doi.org/10.1007/s10570-013-0154-6 [ Links ]
Received: December 13, 2018; Accepted: April 09, 2019