1. Introduction
The Cd isotopes are of specific interest since they have only two fewer protons than the individual closed-shell Sn nuclei. The large abundance of stable isotopes in combination with the interesting properties of the closed proton shell near Z=50 and the presence of neutrons in the middle of the N=50-82 shell make the Z=50 region favored for nuclear structure studies [1-4]. Schraff-Goldhaber and Wesener observed that The Cd isotopes have low lying states that reflect the quadrupole-vibrational excitations of a spherical-equilibrium surface [5]. lachello and Arima [6] successfully described the collective nuclear properties in intermediate- mass nuclei using the Interacting Boson Model (IBM-1). Moreover, the IBM-1 model generated the U(6) group algebra, that then produces three subgroup symmetries: U(5), SU(3), and O(6). These three dynamics are associated with a vibrational, rotational, and 7-soft nuclei, respectively [2,3]. However, many researchers have suggested that nuclei may have an intermediate structure consisting of the transitions U(5)-SU(3), U(5)-O(6), and SU(3)-O(6) [4,5].
Great deal of research on the structure of electromagnetic transitions and energy levels in even-even Cd isotopes have been carried out [6-12]. The stable isotopes of 110,112Cd have already been explained as collective nuclei with multiphonon excitations. Morrison and Smith [13] presented four distinct constant boson interactions in order to illustrate the framework of 108,116Cd isotopes for states with angular momentum (L=1 to 6). Pignanelli et al. [14] used the interacting boson model to study the octupole excitations in nuclei with masses between 98 and 150. The low-spin state in even-even 106-112,116Cd isotopes was examined utilizing γ-ray (in/off-beam) and conversion-electron spectroscopy [15]. Long et al. [7] used IBM-1 to explain the low-lying levels and high-spin states of 116,118,120 Cd. The same procedure was also carried out by Bollaert et al. [16]. Moreover, Kadi et al. [17] used the (n,n', γ) reaction to investigate the lifetimes of various levels as well as the decay parameters of multiphonon quadrupole vibrational states and invasion structures in 116Cd. They found that the intruder pattern is fully confirmed in the 116Cd nuclei. likewise, Gade et al. [18] evaluated the electric and magnetic dipole excitations of 108Cd nuclei and estimated the lifetimes of eight dipoles. Furthermore, the researchers demonstrated a transition path between the dynamic U(5) and O(6) limits for the 108Cd using the IBM-2 framework. Garrett et al. [19] used the interaction boson model to analyze the systematic variation of 110-114Cd isotopes. Their calculations show that Cd isotopes at the two-photon levels reflect the data quite well, while the calculations for the 0+ states in 116Cd fail completely. Systematic variations occur across the Cd isotopic chain at the three-phonon level, demonstrating the breakdown of vibrational motion in low-spin states. Hossian et al. [20] used IBM-1 to study the energy level of 104-122Cd nuclides. They noted that Cd isotopes have a vibrational symmetry U(5). The resulting Hamiltonian was utilized by Nomura etal. [21] to calculate the low-lying excitation bands, quadrupole and monopole electrical transition rates for the even-even 108-116Cd. Many intruder states were predicted, which corresponded to empirical data. Besides, Leviatan et al. [22] show that vibrational symmetry is retained in a part of the 110Cd spectrum and destroyed in certain nonyrast states. In addition, the vibration in the bulk of the low-lying normal states is reportedly preserved. Besides the IBM-1, Harris [23] and Mariscotti et al. [24] for example, used a framework in which higher-order nuclear angular velocity terms in the cranking model were preserved as well as the moment of inertia was extended to excited bands with two variables (go and C). Raduta et al. [25] de-quantized a second-order quadrupole boson using a time-dependent variation principle. They applied the coherent state model technique on nuclei, and used the least squares method to calculate the implicated parameters. This paper aims to use the interacting boson model (IBM-1) with a new empirical equation (NEE) to calculate the ground and other states for even-even 114-128Cd isotopes. The EPS contour was examined for Cd nuclei. In addition, the reduced transition probabilities B(E2) are determined and compared to experimental data.
2. Calculation procedure
For nuclei containing N nucleons, the Interacting Boson Model (IBM) assigns the occupancy to a truncated model space. It provides a quantitative interpretation of indistinguishable particles with angular momentum equal to either 0 or 2 forming pairs. In IBM-1, the Hamiltonian is written as [6,26]
The IBM-1 Hamiltonian can be described in nine terms, two of which appear in one-body terms (s and d). The εs and εd denote the energy of bosons, while the rest are two-body terms (C0, C 1, C 4, v 0,v 2 ,u 0,u 2). The number of bosons Nb, on the other hand, is conserved. In general, the IBM-1 Hamiltonian in Eq. (1) can also be expressed as [28]
where ε = εd - εs is the boson energy, and the operators are defined as follows:
Total number of d-bosons, pairing, angular momentum, and quadrupole are represented by the operators
The IBM-1 performs three types of dynamic symmetry for nuclei: vibrational U(5), rotational SU(3) and γ-soft O(6), with their eigenvalues given by [28].
hence, the energy ε, pairing a0, and quadrupole a2 parameters are influence in the U(5), O(6), and SU(3) limits, respectively. Several nuclei have a property that allows them to transition between two or three of the above-mentioned limits. The new empirical equation (NEE) [29] was used in this study to calculate the energy levels of Cd isotopes. The energy levels of the GSB are determined depending only on the angular momentum (I) as follows:
This formula has three parameters, A1, A2 and A3. These parameters were obtained after fitting all of the positive experimental (GSB) data. Further to that, the following formula can be used to compute the other bands [29]
In addition, the E0 (I=0) and B can be estimated from the γ-and β-bands.
3. Results and discussion
The results for the energy levels of the ground and other states for 114-128Cd isotopes, the energy ratios
The simplest way to determine the IBM-1 parameters is to use the energy ratio (R) as a starting point for calculations. The energy ratio,
Table I The energy ratios of Cd isotopes.
Isotopes | 114 Cd | 116Cd | 118 Cd | 120 Cd | 122Cd | 124Cd | 126Cd | 128Cd |
R4/2 | 2.29 | 2.38 | 2.39 | 2.38 | 2.33 | 2.26 | 2.25 | 2.21 |
Table II Shows the IBM-1 and NEE parameters in MeV for 114-128Cd, except for Nb A2 and A3. The pairing, angular momentum, and octupole operators are denoted by the letters PAIR, ELL, and OCT, respectively.
Isotope | Nb | IBM | NEE | |||||
---|---|---|---|---|---|---|---|---|
EPS | PAIR | ELL | A1 | A1 | A3 | |||
114Cd | 9 | 0.510 | 0.057 | 0.014 | 0.056 | 0.0018 | 0.0055 | -8.6383 |
116Cd | 8 | 0 | 0.071 | 0.013 | 0.051 | 0.5147 | 1.6424 | -2.0039 |
118Cd | 7 | 0 | 0.080 | 0.009 | 0.053 | 0.0335 | 0.1086 | -3.4837 |
120Cd | 6 | 0 | 0.099 | 0.010 | 0.055 | 0.0760 | 0.2287 | -2.1158 |
122Cd | 5 | 0.530 | 0.142 | 0.013 | 0.058 | 0.1274 | 0.3536 | -1.64348 |
124Cd | 4 | 0.580 | 0.153 | 0.014 | 0.063 | 0.0001 | 0.0004 | -12.7729 |
126Cd | 3 | 0.610 | 0.183 | 0.005 | 0.063 | 1.3200 | 3.8510 | -2.6660 |
128Cd | 2 | 0.620 | 0.197 | 0.003 | 0.065 | 1.3179 | 3.8221 | -2.5590 |
Table III NEE parameters of the other band in MeV for the Cd isotopes.
Isotopes | Eo | B |
---|---|---|
114Cd | 1.021 | -0.0005 |
116Cd | 1.1308 | -0.1487 |
118Cd | 1.2231 | -0.0033 |
120Cd | 1.2503 | 0.0005 |
122Cd | 1.4798 | 0.0003 |
124Cd | 1.5895 | -0.2466 |
126Cd | 1.1373 | 0.0109 |
128Cd | 1.1700 | -0.0115 |
The results of the IBM-1 and NEE calculations of the energy levels for ground and other states of the Cd isotope are presented in Figs. 1 and 2, respectively. The low-lying spectrum in Cd nuclei values of the IBM-1 and NEE are really equivalent to available experimental results for 0+, 2 +, 4+, and 6+ states. For the high states, the NEE and IBM-1 calculations are slightly overpredicted with the exception of the 114Cd and 118Cd nuclei, as illustrated in Fig. 1. The levels marked with an asterisk (*) represent cases that the spin or parity of the respective states has not been established experimentally. The IBM-1, NEE, and experimental data on the energy level structures of Cd nuclei appear at first glance to be similar for the other states, as shown in Fig. 2. However, we can render a few predictions based on a comparison of theoretical predictions and experimental results. For the IBM-1 description, the spacing between both 2+ states is slightly overestimated in 114Cd and underestimated in 116-118Cd. There is no gap between both 2+ states in 114-122Cd in the NEE description. Conversely, the comparison between the energies of the states, i.e. for the 6+ states, is significantly improved.
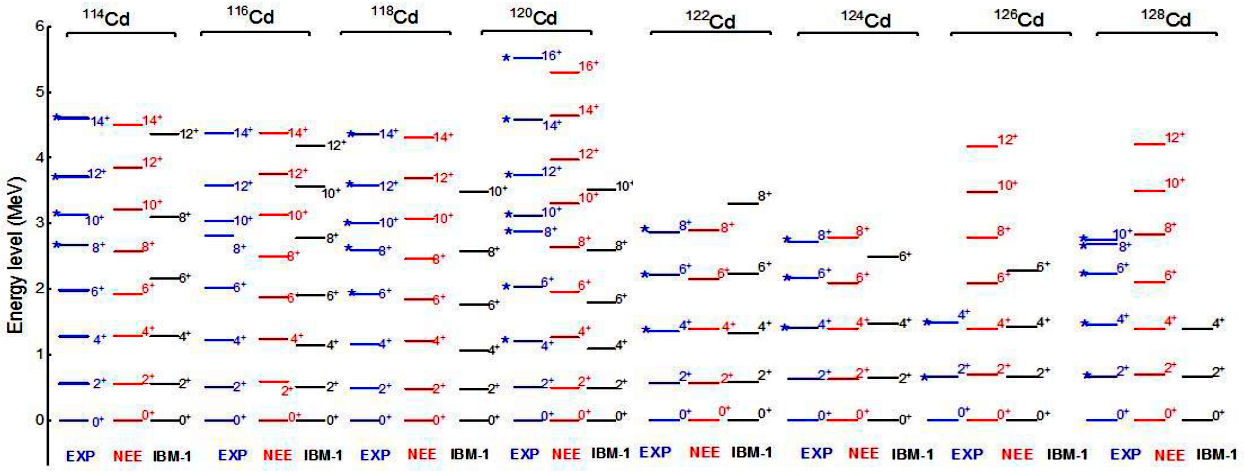
Figure 1 Experimental low-lying energies obtained from [33] in 114-128Cd isotopes compared to IBM-1 and NEE calculations.
As already mentioned, the symmetry shape of a nucleus can be predicted from the energy ratio

Figure 3 Comparison of the
The electromagnetic transitions operator in IBM-1 has a general form [7,28]:
where γ0, α2, and β (L=0, 1, 2, 3, 4) are parameters identifying the different terms in the corresponding operators. The reduced electrical transition probabilities provide information about nuclei structure. The E2 transition operator is recognized to be a Hermitian tensor. As a result, the Nb is always conserved. Then, the E2 transition operator is represented as [34]:
here, the (s†, d†) and (s, d) symbols refer to creation and annihilation operators, respectively. The α2 represents to the effective charge for boson and β2 is a dimensionless coefficient, β2 = χ α2. The B(E2) values for electrical transition probabilities are described in terms of reduced matrix elementsas [28,34,35]:
The effective charges eB for Cd isotopes are shown in Table IV. The experimental values of
Table IV B(E2) values for 114-128Cd were reproduced using an effective charge, eB.
Isotope | Nb | eB (eb) |
---|---|---|
114 Cd | 9 | 0.071 |
116 Cd | 8 | 0.076 |
118Cd | 7 | 0.085 |
120Cd | 6 | 0.087 |
122Cd | 5 | 0.093 |
124Cd | 4 | 0.098 |
126Cd | 3 | 0.104 |
128Hf | 2 | 0.109 |
Table V Theoretical versus experimental B(E2) for 114-128Cd isotopes in unit ofe2b2 are compared.
114Cd | 116Cd | 118Cd | ||||
Ii → If | EXP. | IBM-1 | EXP. | IBM-1 | EXP. | IBM-1 |
|
0.102 | 0.101 | 0.113 | 0.112 | 0.114 | 0.114 |
|
0.213 | 0.267 | - | 0.070 | - | 0.065 |
|
0.072 | 0.141 | 0.084 | 0.152 | - | 0.148 |
|
0.089 | 0.043 | - | 0.000 | - | 0.000 |
|
0.148 | 0.090 | - | 0.000 | - | 0.000 |
|
0.203 | 0.152 | 0.188 | 0.152 | 0.209 | 0.184 |
|
0.105 | 0.083 | - | 0.000 | - | 0.082 |
|
0.390 | 0.152 | - | 0.092 | - | 0.000 |
|
0.058 | 0.075 | 0.504 | 0.342 | - | 0.074 |
|
0.390 | 0.258 | 0.361 | 0.2961 | - | 0.156 |
|
0.426 | 0.261 | - | 0.094 | - | 0.000 |
|
0.283 | 0.161 | - | 0.159 | - | 0.147 |
Ii → If | 120Cd | 122Cd | 124Cd | |||
EXP. | IBM-1 | EXP. | IBM-1 | EXP. | IBM-1 | |
|
- | 0.091 | 0.093 | 0.089 | - | 0.059 |
|
- | 0.118 | - | 0.115 | - | - |
|
- | 0.118 | - | 0.115 | - | 0.072 |
|
- | 0.063 | - | 0.058 | - | - |
|
- | 0.058 | - | 0.112 | - | - |
|
- | 0.121 | - | 0.089 | - | 0.063 |
|
- | 0.107 | - | 0.089 | - | 0.038 |
Ii → If | 126Cd | 128Cd | ||||
EXP. | IBM-1 | EXP. | IBM-1 | |||
|
- | 0.069 | - | 0.072 | ||
|
- | 0.083 | - | 0.072 | ||
|
- | 0.083 | - | 0.072 | ||
|
- | 0.031 | - | - | ||
|
- | 0.028 | - | - | ||
|
- | 0.059 | - | - |
The potential energy surface (EPS) application provides information to determine the microscopic and geometric shapes of nuclei. IBM Hamiltonian produced the EPS plots using the Skyrme mean field procedure [30]. The IBM-1 energy surface is constructed by combining the IBM-1 Hamiltonian's expectation value with the coherent state
where
then, the EPS can be written in terms of β and γ as [32]:
Where α's parameters are associated with the coefficient of CL, v2, v0, and u0, as seen in Eq. (1). The term β refers to a nucleus total deformation. Then, the shape of a nucleus could be spherical or distorted depending on whether β = 0 or not. Moreover, the variation in nucleus symmetry is represented by γ term, when γ = 0, the nucleus has a prolate shape; when γ = 60, it has an oblate shape. The deformation energy surfaces of the even-even isotopes 114-128Cd were estimated as plotted in Fig. 5. The energy surfaces formed a prolate and a slightly oblate threshold that should correlate with proton normal and intruder excitations [21]. The 116-120Cd nuclei, on the other hand, have a deformation shape and a γ-unstable character (γ ≈ 30). While the isotopes 114,122,124,126,128Cd have a potential energy surface that is approximately independent of the triaxial γ-parameter. The U(5)-O(6) transitions were identified in the EPS map for Cd nuclei in the (β, γ) deformation space.
4. Conclusions
Using the IBM-1 and NEE methods, the ground and other state energies, the electromagnetic transition, and the potential energy surface of 114-128Cd isotopes were all calculated theoretically. The results of the ground and other energy levels of Cd isotopes are consistent in some places with previous experimental data, i.e., the description of the energies of the 2+ state is not well represented in the IBM-1 model. Furthermore, the results of the IBM-1 on reduced transition probabilities B(E2) roughly agree with the available experimental data. Similarly, the