1 Introduction
The nuclear fission of actinides begins at the saddle point of the fission barrier when the fissile nucleus is deformed without return and ends at the scission point, from which the fragments separate acquiring their final kinetic energy by Coulomb repulsion. Before and after the scission point neutrons are emitted [1].
One of the quantities proper to study fission dynamics is the average prompt neutron multiplicity as a function of the primary mass of fragments
Recently, a Monte Carlo simulation of the measurement by the 1V1E technique has been performed and applied to the case of 239Pu(nth, f) [17]. In this work, a simulation of the measurement by the 2E technique is performed and applied to that reaction.
The 2E technique consists of calculating the mass of the complementary fission fragments using the measured values of kinetic energy in the momentum conservation relations. Furthermore, conservation of the number of nucleons in the reaction is assumed.
The uncertainty of the value of the kinetic energy is proportional to the number of neutrons emitted before the fragment reaches the detector. The average number of neutrons depends on the mass of the fragment. Added to this is the resolution of the energy measurement, which depends on the detector. As an example, the total resolution of the mass of the Göök et al. experiment is 4 u [18].
2 Input data of the simulation
In the simulation of neutron emission, the state of deformation of the fragments is not considered. Furthermore, the symmetry or asymmetry characteristics of the kinetic energy distribution of the fragments are not distinguished.
The input data are quantities associated with the primary complementary fragments, i.e., the mass numbers (A and A’, respectively), the kinetic energy (E and E’, respectively) and the number of neutrons emitted by each fragment (n and n’, respectively).
As a function of one of the primary complementary fragments mass (A), we use the following quantities: the yield Y (Data taken from Ref. [19]. See Fig. 1); a Gaussian distribution of total kinetic energy of complementary fragments (TKE = E + E’) with its average

Figure 1 Simulation of the measurement by the 2E technique of the mass yield of fragments from the 239Pu(nth,f) reaction. We show the yield of the primary fragment mass (Y(A) diamonds, taken from Ref. [19].) and the yield of simulated as measured (by 2E technique) fragment mass (
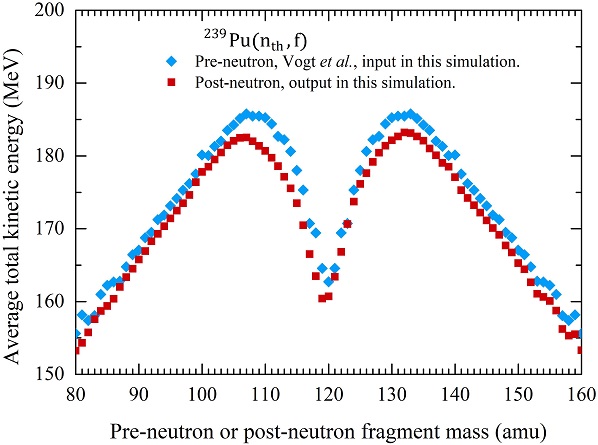
Figure 2 Simulation of the measurement by the 2E technique of the average total kinetic energy as a function of the mass of fragments from the 239Pu(nth,f) reaction. We show the average total kinetic energy as a function of the primary fragment mass (diamonds, values taken from Ref. [19]) and the corresponding to the pseudo mass (squares), which are the input and output data, respectively, in the simulation.
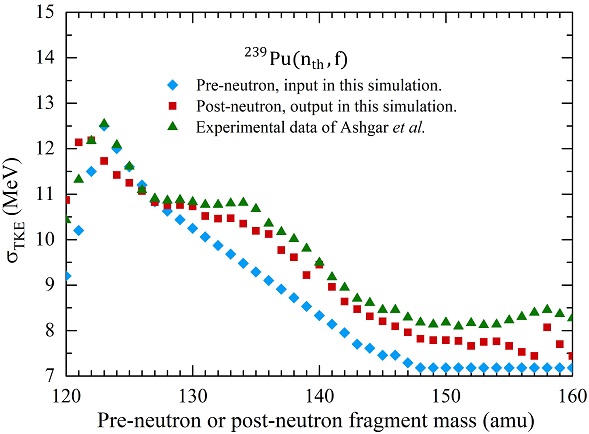
Figure 3 Simulation of the measurement by the 2E technique of standard deviation of the total kinetic energy distribution as a function of the mass of fragments from the 239Pu(nth, f) reaction. We show the standard deviation of the total kinetic energy distribution as a function of the primary fragment mass (diamonds) and the corresponding to the pseudo mass (squares), which are the input and output data, respectively, in the simulation. For comparison, experimental values (triangles, from Ref. [20]) are presented.

Figure 4 The average neutron multiplicity (measured by the 2E technique), as a function of the mass of fragments from the 239Pu(nth,f) reaction. We show the simulated average prompt neutron multiplicity as a function of the primary fragment mass (

Figure 5 Simulation of the measurement by the 2E technique of the average neutron multiplicity as a function of the mass of fragments from 239Pu(nth, f) reaction. The slope of neutron multiplicity against total kinetic energy, -dv/d <TKE> (MeV-1), plotted as a function of primary fragment mass (diamonds) an approach from the experimental data (circles) from Ref. [13].
Based on the anti-correlation of neutron multiplicity associated with the complementary fission fragments observed by Vorobyev et al.
[21] in the case of the reaction 252Cf (sf), a term obeying a Gaussian distribution with an average 0 and a standard deviation
The conservation relations of mass, energy, and linear momentum of the primary fragments with masses A and A’, are the following:
Where A0 = 240, and
3 Output data of the simulation
The output data are quantities assumed to be measured by the 2E technique associated with the complementary fragments, i.e., the mass (
and
With the double energy technique,
and
From (4)-(6), we obtain
where
4 Results
In the fragment mass region around A ≈ 15, referred to the Y(A) curve, the yield curve of the calculated mass
In the fragment mass region around A ≈ 125, referred to the Y(A) curve, the yield of the calculated mass
In general, the average final total kinetic energy
Curve
Figure 4, we represent the simulated average of prompt neutron multiplicity as a function of the pseudo mass

Figure 6 The average neutron multiplicity (measured by the 2E technique), as a function of the mass of fragments from the 239Pu(nth, f) reaction. We show the simulated average prompt neutron multiplicity as a function of the primary fragment mass (
where
5 Discussion
We simulated the measurement, by the 2E technique, of the average prompt neutron multiplicity as a function of fragment mass for the 239Pu(nth, f) reaction. Compared to input values