1. Introduction
The Continuum-Discretized Coupled Channels (CDCC) method is a quite important method for explaining the nuclear breakup processes in direct nuclear reactions involving weakly bound nuclei [1-5]. The CDCC method helps to investigate the structure of the halo nucleus as well as explaining the reaction dynamics [1,4,6]. It can be regarded as an expanded form of Coupled Channels (CC). In order to solve the CDCC method, initially, a three-body system was created. In the CDCC method, a three-body system consists of the two-body projectile and the target nucleus (P → C + v). Then, a four-body system was studied by using the projectile as a three-body system. The CDCC method includes quantal bound and continuum states of the projectile. For the bound and continuum states of the projectile, the three-body Schrödinger equation solution is performed by including the three-body wave function. The continuum states were determined through the k linear and angular momentum. The k continuum is divided into thousands of parts, and this discretization is called the Av average method [3,6-8]. The phenomenological and microscopic optical potential is generally used as the interaction potential between the projectile and the target [4].
17O is the mirror nucleus of 17F, and these isobars display similar features in the ground state and the first excited state. However, their neutron- and proton-binding energies are quite different from each other, such that Sp= 0.600 MeV for 17F and Sn= 4.143 MeV for 17O. Because these nuclei show similar properties, 17O and 17F nuclei were generally investigated in the same studies [9,10].
Recently, the elastic scattering of 17O projectile on different targets has been studied using various methods, and many results have been obtained [9,10]. It is aimed to reproduce theoretically the experimental data and contribute to the previous studies on the subject. Thus, this study aimed to investigate the interaction of 17O projectile with different target nuclei such as 90Zr, 124Sn, and 208Pb at 340 MeV on the Coulomb barrier, to analyze a cross-section of elastic scattering for the 17O+ target systems, and to look at the role of the excited channels. Firstly, we analyzed the elastic scattering angular distributions of 17O+ 90Zr, 17O+ 124Sn, and 17O+ 208Pb systems. Then, we examined the effect of multiple channels with the CDCC method. Finally, we compared the calculated results with experimental data.
In Sec. 2, a basic description of the theoretical process is presented. Section 3 shows the results and discussion, and Sec. 4 provides the conclusion.
2. Theoretical Process
The CDCC formalism
The interaction of a projectile consisting of the target (T), core (C) and a valance (v) particle that is weakly bound to it, is the equation for three-body scattering is shown below:
The three-body Hamiltonian for the system is as follows:
Here, H int = T r + v(r), and v(r) is the interaction between the valance (v) and core (C). T r and T R are the kinetic energies of the projectile-target system bound by r and R. U C (r C ) and U v (r v ) are the interaction potentials of the target (T), core, and valance particle. The T + C + v threebody system and Jacobi coordinates are presented in Fig. 1. The Hamiltonian is generally expressed as (r,R) in Jacobi coordinates [2,3,6].
The total wave function Ψ is determined by including the eigenfunctions of the H int Hamiltonian of the projectile system. The total wave function is shown below:
Here, ϕ i represents the bound and continuum states of the H int Hamiltonian. χ i (R) represents the motion between the target and the projectile. If put into the Schrödinger equation, the standard CDCC equation is represented as follows:
Here, U j = U C (r C ) + U v (r v ) and the coupling potential is
In the CDCC method, continuum states for the corevalance system are represented by linear momentum (k) and angular momentum (l). The (k) continuum is divided into bins. Then, for each bin, the average of the continuum state is taken as a single state using the (Av) average method [3,11].
3. Results and discussion
Interaction between the 17O projectile and the 90Zr, 124Sn, and 208Pb target nuclei was analyzed at 340 MeV by the CDCC method. The elastic scattering cross-section results were obtained for 17O + 90Zr, 17O + 124Sn and 17O + 208Pb systems. In the calculations for three interactions: core-valance, core-target, and valance-target, interaction potentials were used for a three-body system. These interaction potentials are determined as n+16O, 16O+target and n+target, respectively. n+target interaction potentials were obtained by using global parameters of Koning-Delaroche and Bechetti Greenless [12,13]. Optical potential parameters for 16O+target and n+target systems are given in Tables I and II, respectively. These potentials were determined as optical potential, whose real and imaginary parts were chosen to be standard volume Woods-Saxon shapes. The calculations were performed by using the FRESCO code [14].
TABLE I Optical potential parameters for 16O+target systems in the CDCC method
System | V 0 | r 0 | a 0 | W V | r V | a V |
---|---|---|---|---|---|---|
(MeV) | (fm) | (fm) | (MeV) | (fm) | (fm) | |
16O + 90Zr | 100.0 | 1.1 | 0.58 | 30.0 | 1.3 | 0.7 |
16O + 124Sn | 55.0 | 1.2 | 0.6 | 32.0 | 1.3 | 0.7 |
16O + 208Pb | 60.5 | 1.07 | 0.75 | 10.0 | 1.0 | 0.8 |
TABLE II Optical potential parameters for n+target systems in the CDCC method.
System | W V | rV | a V | W V | rV | a V |
---|---|---|---|---|---|---|
(MeV) | (fm) | (fm) | (MeV) | (fm) | (fm) | |
n+90Zr | 54.7 | 1,21 | 0.66 | 14,6 | 1.218 | 0.66 |
n+124Sn | 50.18 | 1.22 | 0.66 | 14.5 | 1.218 | 0.66 |
n+208Pb | 32.68 | 1.17 | 0.75 | 5.19 | 1.32 | 0.65 |
In the CDCC calculations, the continuum of the 17O projectile is discretized in the kmax space and then divided into momentum bins with a width of ∆k. The k term is the linear momentum of the n+17O interaction. For calculations, only ≤ 3 continuum states were taken into account.
Figure 2 shows the elastic scattering angular distribution of the 17O + 90Zr system at 340 MeV. The solid curve shows the results of single-channel CDCC calculations; the dashed curve shows the multi-channel calculations, and the red diamonds show the experimental data that was provided by Ref. [15]. It is seen that the theoretical results obtained for this reaction are quite consistent with the experimental data. The 16O + 90Zr system potential for core-target interaction was reproduced from Ref. [16]. It was seen that the breakup effect of 17O is remarkable and occurs at large scattering angles; the coupling slightly increased the differential cross-sectional value. However, at small angles, there was very little effect. In this case, the effect of excited channels was considered to be small. The angular distribution for the 17O + 124Sn system at 340 MeV on the Coulomb barrier is presented in Fig. 3. Experimental data were taken from Ref. [17]. The solid curve shows the results of single-channel calculations: the dashed curve shows multichannel calculations, and the red diamonds show the experimental data. As seen in Fig. 3, the excited channel effect is rather small, and the results of CDCC calculations were quite in agreement with experimental data.

FIGURE 2 The elastic scattering angular distributions for the 17O +90Zr system at 340 MeV using the CDCC method. The experimental data was taken from Ref. [15].
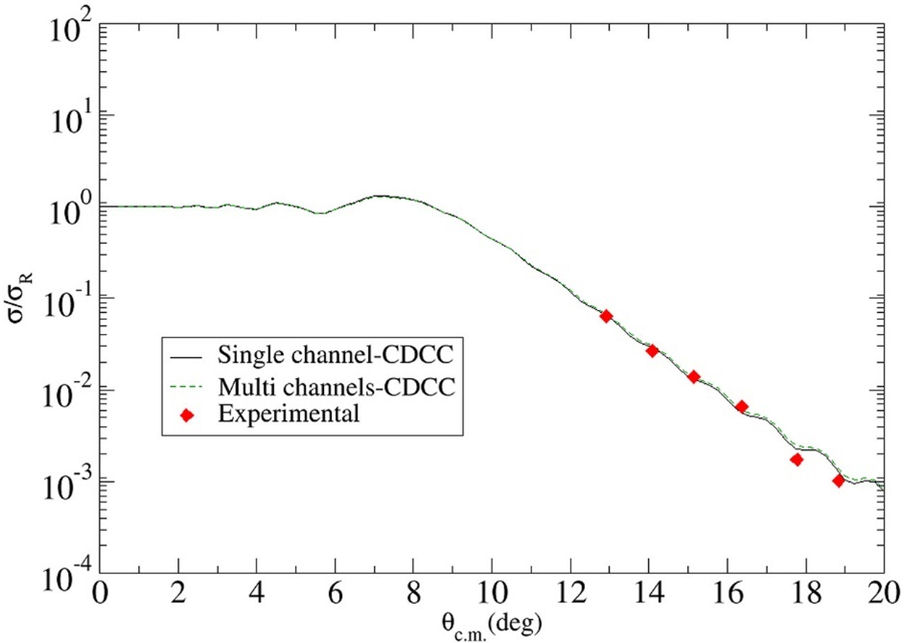
FIGURE 3 The elastic scattering angular distributions for the 17O + 124Sn system at 340 MeV using the CDCC method. The experimental data was taken from Ref. [17].
The results obtained from the 17O + 208Pb interaction at 340 MeV on the Coulomb barrier are presented in Fig. 4 The experimental data were taken from Ref. [18]. It was seen that the results are close to the experimental data. It was also seen that the results obtained through the single-channel calculation were better than multi-channel calculation.

FIGURE 4 The elastic scattering angular distributions for the 17O + 208Pb system at 340 MeV using the CDCC method. The experimental data was taken from Ref. [18].
In this reaction, the effect of the excited channel is higher compared to the other two systems.
4. Conclusion
The CDCC method is remarkably good for investigating the reactions that involve weakly bound nuclei. In conclusion, the CDCC method was performed for three-body 17O elastic scattering by three different nuclei at 340 MeV above the Coulomb barrier. The CDCC method was performed for a three-body system by taking the projectile as 17O→ 16O+n, and calculations were made accordingly. The optical potential was used for the interaction potentials of the three reactions. 17O+target interactions at 340 MeV displayed good agreement with experimental data. The contribution of excited channels for 17O + 90Zr and 17O + 124Sn systems was quite small. For these systems at the 340 MeV, the results show that the difference between single-channel and multichannels is not significant. However, it was observed that excited channels affect the 17O + 208Pb system. The result is remarkable for this system at 340 MeV, and single-channel calculation explains better than multi-channel calculation the experimental data quite well. The results of these calculations show the importance of the structure of a weakly bound 17O projectile nucleus.