1.Introduction
Manufacturing industries work with large machinery (electronics, tools, plastics, etc.). Two scales segment machinery: a) greater scale (as power plants, refineries, petrochemicals) or micro-scale (transistor manufacturer). These machines are prone to use any of their moving parts where they come into contact with various materials. A study carried out in the United Kingdom in 1997, showed that the cost by wear was 650 million Pound Sterling each year, which represented the 0.25% of its annual turnover, 1. Corrosion and wear are major failure mechanisms that affect performance, safety, and material integrity. The polyvinyl chloride (PVC) polymer manufacturing industry currently presents serious corrosion-abrasion problems due to high-temperature process conditions. The combined effect of wear and corrosion constitutes 5% of the causes of process operating infrastructure failure in this sector, making it the fifth most important degradation mechanism in terms of cost due to premature failure 2, 3. The increase in tool life due to coatings depends on the improved wear resistance at high cutting temperatures due to the reduced friction against the working material and the tool. The area of application of the coatings has been extended to the last few years, e.g., application on ordinary TiN-coated tool steels. Progress in thin-film preparation techniques has greatly increased the potential application areas for carbides and nitrides since they have a very low coefficient of friction and are very hard 4. For example, tungsten nitride (WNx) belongs to refractory metal nitrides that have the unique properties of excellent hardness (38.9 GPa), inert chemistry, high melting point, high chemical stability, and thermal conductivity 5. On the other hand, the titanium-tungsten nitride shows a good tribological behaviour with a low friction coefficient (0.4) 6. The multilayer is formed by the stacking of layers alternating two materials of different properties. It has been observed that the increase of parallel interlayers on the surface limits the propagation of cracks, allowing the toughness of the set, increasing the number limits phases associated with the decrease of grain size, increasing the toughness. This behavior is ruled by the Hall-Petch equation, which states that the elastic limit of a polycrystalline material (σ), is determined by the mean grain size (d) and (σ0) and k are two experimental constants. The hardness increase in the superior multilayers, is attributed by the law of mixtures, the hardness is given by the average of the two materials, according to the proportion of their respective volumes 7. For these reasons, the individual properties of each material are enhanced as a whole in a system providing better properties. On the other hand, AISI 1060 tool grade steel contains carbon 0.55-0.65 %, magnesium 0.60-0.90 %, phosphor < 0.04% and silicon 0.05 %. Rockwell B hardness 1.8 GPa and elastic module 90-210 GPa. It is used in agricultural implements, clutch discs, shafts, arrows, and cutting tools such as knives. Due to the absence of Nickel and Chrome alloys in steel, in contact with the polymeric matrix of Vinyl Chloride, the metal surface shows abrasive wear. One friction experiment carried out by 8, showed that below 60oC, the slip between PVC and steel, the surface damage was negligible. However, as the temperature between the contact pair increases, wear is generated in PVC waste and steel particles 0.444 mg of mass.
This study is the second part of a previously reported results 9, that found for the case XRD, the formation hexagonal structure δ-WN and WN phases. The crystal size of both coatings is similar between them (24 nm and 23 nm). Elemental composition was obtained by SEM-EDS containing atomic 50 %; thus, the formation W2N is not favorable. The microstructure showed columnar typical growth in the deposition PVD; it observes spherical cones and cracks on the top surface by SEM. The thickness for coating A and B measurements by cross-section SEM, was 6.6 and 6.4 micres, respectively. The grains size obtained by AFM is 45.3±1.9 nm coating A and 40.4 ± 0.1 nm coating B. For the case of dynamic polarization curves the coating A, the current result shows a density (1.89 x 10-7 A), it tends to be an ennoblement material compared with bare substrate steel. However, in the results obtained from spectroscopy impedance electrochemical showed that the coating B presents a high resistance to polarization (30 KΩ• cm2). Thus, this experiment consist to study the behavior of Ti and W metallic as buffer layer for adhesion improvement. The compounds of WN and WTiN to improve hardness, corrosion and tribological properties. The experimental design consists of the formation of two blocks of multilayer: The first block multilayers is deposited Ti/W/WN/ WTiN/Ti/ TiN ending with a system multilayer WTiN/W/WN, assigned as coating A. The second block experimental consist to interchanged the position of W, Ti as buffer layer using the next multilayer system W/Ti/TiN/ WTiN/W/WN conserving the similar end multilayer, assigning as coating B. For more details of experimental design, see the A. Gonzále’s work et al., 2018.
2.Materials and method
2.1.Materials and substrate preparation
Coatings are obtained through the use of discs 2-in diameter and 0.25-in thickness of Ti and W targets high purity. The substrate used corresponds to AISI 1060 carbon steel, whose elemental chemistry composition is 0.65 % carbon in square plates of 28 x 28 mm and 3 mm of thickness; while, the substrate is for mechanical measurement properties. To determine, the molecular interactions layers were deposited on a silicon wafer (100). The AISI 1060 substrates were prepared using sandpaper (grain / in2) from 100, 220, 600, 1000, 1500, and 2000. For the polish substrate, aluminum oxide (Al2O3) solution particle size 1 μm was used, while the process is carried out using a rotatory source. All substrates were submitted a soap industrial cleaning next to the ultrasonic process with alcohol and acetone, it drying with compressed air.
2.2.Equipment, deposition and coatings characterization process
The multilayer coatings deposition process is carried out using a chamber r.f vacuum sputtering magnetron system designed and adapted by the University of Guadalajara Researchers. This system is equipped with four magnetrons and a rotary holder. The generation of plasma was made through to use of power supply d.c. Advanced energy brand. To carrier deposition of coatings A and B were obtained applicating a current of 0.45 amperes, 456 V, and 461 V on target respectively. The previous deposition coatings are carried a high vacuum to 3 x 10-6 Torr. The work pressure was 11 and 69 mTorr, into chamber with a rate argon (Ar) and nitrogen (N2) gas of 60% and 40%, respectively. The substrates were put on rotatory holder sample are pre-heating around a temperature 200oC; these conditions deposition were reported previously by 9.
For optical properties is carried using by spectrometer Perkin Elmer using 4000 to 400 cm-1 range, it is plotting 2500 at 400 cm-1 with scanning number in a sample of 500, mirror speed at 100%. To obtain the Raman spectrum the Horiba Jobin Yvon model Libran HR distance focal of 800 mm, detector CCD analysis range 300-2000 cm-1 Raman shift, spectral resolution of 633 nm were used. Nanoindentation test, it is using a NANOVEA tribometer®, it is coupling a stylus indentator Bercovich diamond, it is applying a constant load of 20 mN. Oliver and Pharr’s method was used to calculate the hardness. Tribology test, was obtained using a CSEM tribometer Instrument, applying a constant load of 10 N, using a ball steel chromium (100 Cr6) radius diameter 10 mm, displacement distance of 500 m, and linear speed of 0.52 m/s. The environmental conditions were a temperature of 18oC and 57 % humidity. Scratch test is carried out by the Multiscratch NANOVEA® equipment, applying progressive loads starting at 0.1 until 35 N, with relation load 14 N/ min, speed 1.404 mm/min using Rockwell nanoindentation stylus, coupling in focus camera to obtain images.
3.Discussion and results
3.1.Optical analysis
3.1.1.FTIR spectra
In Fig. 1, for both coatings, it presents a bridging system of the W2O the weak, and sometimes broad bands observed in the 400 to 300 cm-1 range are then likely to be due to deformations of the tungsten-oxygen double bond systems, 9. Also, three regions appear for the WO3 vibrations at 900-600 cm-1, 400-200 cm-1, and below 200 cm-1, 10. In the frequency of 417 cm-1, it localized a weak band absorption corresponding to the bonding of W-O (δ W-O), this peak is assigned stretching band 11, 12. In the frequency 560 to 460 cm-1, it presents the band absorption bending vibrations of Ti-O-Ti groups, 13. In the frequency 555 cm-1, an absorption band is localized due to a stretching bond of TiC2 molecule in fundamental state at bond Ti-C2, 14. Besides, it observes a weak band of asymmetric absorption the bond W-N-W, both coatings, 15. In the bands situated at 679 cm-1 and 805 cm-1, which are assigned to stretching vibrations v (W-O-W) of the bridging oxygen atoms, 16. The frequency ∼1030 cm-1 is attributed at the presence of tungsten oxide (WO3), probably a consensuses contamination by residual oxygen in chamber previous an ionic sputtering, 17. In the absorption band located at ∼1297 cm-1 which is attributed to the C-C link stretching vibrations, 18. Only the coating A to appear the low frequencies ∼1459 cm-1 of double bond oxygen-carbon (C=O) stretching vibration band, 19, 20. On the other hand, the band absorption assigned ∼1650 cm-1, represents N-H bending vibrations, 21, 23.
3.1.2.Raman spectra
Figure 2, shows the Raman spectrum in multilayer coatings W/WN, TiN/TiN, and WiTiN obtained by DC magnetron sputtering. As well known, the inorganic compound has vibrational bands mainly below 1500 cm-1. In Fig. 2 of coating A, presents a weak peak in the dispersion band assigned at 260 cm-1 in the interval 250-435 cm-1, corresponding to transverse phonons (TA), of metallic ions vibrations of Ti for both coatings (A and B), 24. In the frequency 520 cm-1, an intense peak (very sharp) of dispersion acoustic phonons appears; this is a characteristic crystalline silicon wafer, where the angles, the power, and bonding energy are very ordered uniforms, 25, silicon like a diamond. It has only one first order Raman active phonon located at the Brillouin-zone center, long wavelength transverse optical phono (TO). On the other hand, at the 938 cm-1, a weak peak is observed, it indicates that Raman positions depended on the tungsten content, the higher frequency at which the band appears, and the removals of water cause a shift of Raman bands to higher frequency 12. For the case of coating B, it localized a band of 658 cm-1, it is attributed to the N-Phase, while, it is interacting with the oxide tungsten (WO3), [276]. The frequency at 811 cm-1 is assigned to the Raman band, characteristic of monoclinic WO3. It was obtained and not changed as a function of temperature indicating the formation of highly stable monocrystalline WO3, 27, 28.
3.2.Nanoindentation test
In 29, he found the direct proportional relationship between hardness and modulus of elasticity, i.e., greater hardness increases the elasticity of the material. To determine the hardness (H), and the modulus of elasticity (Er), the Oliver and Fharr method is used, 30, it is described in the next Eq. (1).
where H is the coating’s hardness
To calculate the resistance at plastic deformation (GPa), the next relation is used Eq. (2):
where H is the coating’s hardness (GPa), and E r , is the modulus of elasticity value of the material.
On the other hand, to calculate the elastic recovery (%), the next equation is used Eq. (3) 31:
where R is the elastic recovery (%),
Figure 3 shows the load and discharge curves of the nanoindentation tests for the multilayer A and B coating deposited by DC Sputtering. It can be seen that the A coating, applying a load of 20 mN, tends to penetrate to a depth of 500 nm. The material presents plastic deformation since the discharge curve does not return in the same direction in the load curve with a significant gradient between the two curves. However, for the case of coating B, the penetration is less than A (450 nm) and likewise, its plastic deformation is less. Table I shows the mechanical properties, while the results of the elasticity module and hardness are similar between them. However, coating B presents the best conditions of elasticity recovery (46 %) and maximum deformation resistance (5 %). The behavior of low hardness and the elastic module can be attributed by the surface fracture on coatings, it promoted compressible stress, due to the electron thermal diffusion, energy transfers to the lattice and heat is transported into WTi/Si target. Equalization of temperatures happened at approximately ten picoseconds. The thermalization properties of WTi thin film and Si substrate are quite different; thus, fast heating and cooling are presented, 32. On the other hand, 33, mention in their research, that, the low hardness in multilayer coating is due to the amortization associated with important increase of W-O (W25O75) bonding ionic obtained hardness values of 7.7 GPa.
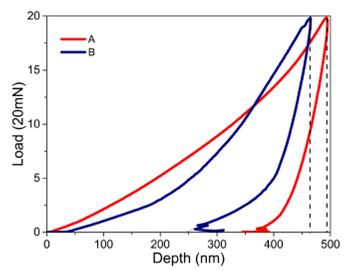
Figure 3 Typical nano-indentation curves (load) versus depth (nm) of multilayer coatings A and B on AISI 1060 deposited by DC magnetron sputtering.
3.3.Tribology test
3.3.1.Pin on disk test
Figure 4 shows the values friction coefficient (COF) and the wear loss in multilayer coatings on a steel carbon substrate. For the case of the bare substrate, it presents a COF of 0.83 ±0.03, whose result is similar to the reported by 34. Coating A, presents a little friction between coatings and the ball, allowing a COF below of 0.70±0.04. Coating B presents a low COF of 0.61 ±0.03 compared with the bare substrate. These COF values are similar to those obtained by Polcar and collaborator’s work 35, using an Al2O3 ball as a counterpart reporting COF values in WN coatings of 0.6 and W87O13 of 0.73. Another factor, the COF of uncoated substrate influences the composition in the microstructure of material in the steel. This due to the presence of austenite grains, with uniform carbide precipitation in the microstructure formed by the perlite phase and a ferrite phase and Eutectoid composition, 36-38. The reason for the friction behavior using counterpart WN-100 Cr6 is attributed to a combination of factors, such as the initial contact area, the chemical reactivity of the contact surface, and the formation of debris, 39. Another factor, that has been reported, is the higher correlation directly COF versus roughness, thus, if the roughness increases, so do the COF, as well as, the generation of cracks on the top surface, promoting anti-slip agents to induce the increase of slide load and friction, 40, 41. In this case, morphological analysis of previous reported, the coating A and B have shown cracks with spheres domes sharp on the top surface; thus, it inducing increase COF 8.
3.3.2.Calculation of wear rate
To calculate wear rate is uses the next equation considering WN density 17.8 gr/cm3, the sliding distance of 500 m, an applicate load of 20 mN, 42, 43.2
where 𝑊 is wear rate in mm3/Nm,
In Table II, shows the mass loss and wear rate for coating A and B. For the case of coating A mass loss and wear rate were 0.00137 gr, and 7.7 x 10-3 mm3/Nm respectively. These results are similar to the ones obtained for the case of coating B, while values are 0.00140 gr., and 7.8 x 10-3 mm3/Nm, respectively. The values of mass loss and wear rate are high significantly can be influenced by the nature of the counterpart (100 Cr6) which tends to produce oxidation products with high and unstable friction. The variation in the instability of the friction process is due by wear particles (debris) expelled from the contact surface (as observed in the imagens of coating A and B).
3.3.3.Analysis contact pressure
To calculate medium contact pressure (
Where
In Table III shows the values of Hert’z contact stress. The maximum Hertzian contact pressure for coating A is 0.924 GPa, value similar for the coating B, which result is 0.910 GPa. This values compared their Young’s modulus is less, thus, does exist deformation elastic in the system pare tribomechanical.
3.4.Scratch test
Figure 5(a-b) shows the behavior of the multilayer scratch test of coatings A and B on AISI 1060 deposited by DC magnetron sputtering. Figure 5a, shows the friction coefficient curves in function at the increase of load. It is observed in coating A that the critical load (Lc1) is causing damage around 2 N and the second load localized (Lc2) coating detachment at 7 N. For the case of coating B, a sliding friction curve a peak is observed when a 7 N load is applied. This peak is attributed by the presence of spherical cones containing diverse hardness properties. For Fig. 5b, in all the cases, the evolution of the power Hertziana (dark shadow) is observed and produced for the application of progressive loads. Three main fails modes in the coating are identified; a) Irregular edges with longitudinal shading trajectory across the track (Lc1) at 0 - 2.3 N load, which is associated with the onset of Chevron-type cracking 47, indicating a cohesion failure in the coating; b) Semi-circular edge material peeling off the track with a diameter of ∼100μm (Lc2) when a load of 7.4 N is applied associated with the onset of chipping failure extending from tensile arc cracks, indicating adhesive failure between the coating and the substrate; and finally c) Coating detachment within the test track with loads greater than 10.7 N is observed- 35 N 48-50.
4.Conclusions
FTIR spectra analysis has shown absorption bands of W-O (tungsten oxide) and oxygen-carbon double (C=O) interactions typical in the deposition by PVD. Raman spectra analysis confirmed the presence of a weak peak of Ti metallic ion vibrations a low frequency and dispersion band corresponding to bonding WO3 monoclinic. The hardness properties by nanoidentation, shown low improvement; however, it is better than bare steel (AISI 1060). The tribology test by a pin on a disc, shown similar values of COF in 0.65 to 0.85 for both coatings. This behavior can due probably the unsuitable selection of ball (steel 100 Cr6), promoting the generation debris by contact. Another factor is the due presence of cracks and spherical domes on the surface top in both coatings. The mass loss and wear rates showed high values in the two coatings. For Scratch test were identified three mechanics of wear: a) Irregulars border appear dark shadow (chevron); b) Semicircle detachment on track and c) Centre detachment on way track in function to displacement. For improvement tribo mechanical properties, it recommended decreases thickness to three micres to eliminate tension stress.