1. Introduction
Lithium nucleus is considered as one of the most interesting and widely used nuclei in lithium-induced nuclear reactions and scattering processes on various targets. One reason could be attributed to the well-known cluster structure of 6Li consisting of a core (α-alpha) and a valence (d-deuteron) orbiting the core. In the past decades, lithium scattering angular distributions have been available for a variety of targets and beam energies. There are several studies to reproduce the experimental angular distribution measurements using the phenomenological Woods-Saxon (WS) optical potential as well as double folding (DF) potentials [1-12]. The energy dependency of 6Li+16O was performed by Rudchik et al. [11], and the data on the effect of nucleus-nucleus interaction were obtained from the internal structure of the colliding nucleus. The general inferences based on the previous works on the lithium elastic scattering of different targets (including 16O nucleus) are: a) the capability of the optical model to reproduce the experimental data over a wide range of bombarding energies, b) Appreciable deviations generally occur for backward angles, c) For a given reaction, the potential parameters are either constant or change smoothly with bombarding energy, d) The observed discrete ambiguities of the families of potential parameters cannot be experimentally reduced unless data at higher bombarding energies are available, and e) Spin-orbit effects do not play a significant role.
Poling et al. [1] analyzed the angular distributions for 6Li+12C and 7Li+12C elastic scattering at beam energies ranging from 4.5 to 13.0 MeV using optical potentials of four varying parameters, with the imaginary potential depth varying linearly with energy. In another phenomenological study, Bindal et al. [2] analyzed 6Li+12C elastic scattering at energies greater than 20.0 MeV using optical potentials of six varying parameters, where both the real and imaginary potential depths varied linearly with energy. Poling et al. [3] measured and analyzed the angular distributions for 6Li and 7Li elastically scattered from 10B, 12C, 13C, 16O, and 28Si at energies between 4.0 and 13.0 MeV. The experimental data for the different nuclear systems considered in this study were reproduced using the optical model (OM). In certain cases, the back angles were not well fitted by parameter sets, which were otherwise useful for all energies. The effects of compound nucleus formation are hypothesized to be the cause of rapid energy variations in the angular distributions. Vineyard et al. [13] and Trcka et al. [14] measured and analyzed the angular distributions for 6Li+16O system at energies 25.7 and 50.0 MeV. The data were analyzed using both OM and double folded potentials with renormalization factors 0.61 and 0.65 at energies 25.7 and 50.0 MeV, respectively. In other words, the potentials were to be reduced in strength by about 37% to reproduce the data.
This paper is organized as follows. In Sec. 2, the theoretical calculations based on the experimental data are presented. Section 3 is devoted to the results of the theoretical analysis and discussions. The summary is given in Sec. 4.
2. Theoretical calculations
The angular distributions for 6Li+16O elastic scattering in the energy range of 13.0 - 50.0 MeV are reanalyzed using different phenomenological and semi-microscopic potentials such as optical potential (OP), double folding (DF), and cluster folding (CF) potentials. Firstly, the elastic scattering data for 6Li+16O at energies 13.0 MeV [3], 20.0 MeV [5], 25.7 MeV [13], 30.0 MeV [15], 32.0 MeV [16], 36.0 MeV [17], 48.0 MeV [18], and 50.0 MeV [14] are investigated within the framework of OM. The parameters defined by Trcka et al. [14] are taken as starting parameters. The analysis employed real and imaginary volume central potentials together with Coulomb potential. The spin-orbit interaction for 6Li has a little influence on the differential cross-section, thus it was not considered. The used potential has the following form:
where f(r, r x , a x ); x = V, W is the Woods-Saxon form factor which equals to [1 + exp[r - r x A 1/3)/a x ]]-1 and V c (r) is the Coulomb potential caused by a uniform sphere with a charge equal to that of the target nucleus and radius r c A t 1/3. Two parameters “r v and r w ” were fixed during the search to the values 1.37 and 1.88 fm, respectively, and the rest four parameters V 0 , a v , W o , and a w were allowed to be changed freely to minimize χ 2, defined by:
where N is the number of experimental data points σ(θ i )cal and σ(θ i )exp are the calculated and experimental cross-sections, Δσ(θ i ) is the uncertainty of the data. By employing this technique, we could obtain an energy dependence on both real and imaginary potential depths. All the calculations in the present work were performed using the code FRESCO [19], and SFRESCO search code was used in searching for the optimal potential parameters. The Coulomb radius parameter was taken as a fixed value at 2.3 fm in all calculations.
Secondly, the available data sets for 6Li+16O angular distributions were analyzed using the double folding optical model (DFOM). In this model, the real part of the interaction potential was derived based on DF, where 6Li and 16O densities in their ground states are folded to nucleon-nucleon potential using the code DFMSPH [20]. The real part of the potential is calculated from the equation given below:
where v
nn
(S) is the effective nucleon-nucleon interaction with
The imaginary part of the potential was taken in the Woods-Saxon shape; the optimal imaginary potential parameters obtained from OM calculations were taken as it is in the DFOM calculations, and consequently, only one variable parameter N R “renormalization factor for the real part of the potential” was used in the calculations. The nuclear potential, in this case, has the following shape:
Thirdly, motivated by the well-known d + α cluster structure of 6Li, the existing experimental data for 6Li+16O elastic scattering in the energy range of 13.0 - 50.0 MeV were analyzed using the CF model potential as the basis. The basic ingredients needed to calculate the CF potential for 6Li+16O system are d + α binding potential, required to calculate the wave function for the relative motion of the d and α in 6Li, and d + 16O and α + 16O optical potentials, obtained by fitting the respective elastic scattering data for nearly the same value of E/A for the data of interest. The d + 16O and α + 16O optical potentials were taken from the literature [24,25]. The bound-state wave function for the relative motion of d and α in the cluster plus core configuration in 6Li represents a 2S state in a real Woods-Saxon potential with a fixed radius r = 1.15 and diffuseness a = 0.65 fm. The potential depth was adjusted to reproduce the binding energy of 1.4737 MeV of the cluster. Both the real and imaginary parts of these potentials were then folded over the cluster wave function to produce the 6Li+16O CF potential.
3. Results and discussion
One of the aims of the current study was to investigate the energy dependence of 6Li scattering from 16O to obtain energy-dependent optical potentials and global optical potential parameters for the 6Li + 16O system. In the last few years, more data have become available on energy dependence, and the upper energy limit has extended. The old energy-dependent optical potentials are not adequate to produce correct data, particularly, at large angles, and high energies. Thus, there is a need for improved average optical potentials for this nuclear system. We performed an (OM) analysis for the 6Li + 16O elastic scattering angular distributions in the energy range of 13.0 - 50.0 MeV using pure phenomenological Woods-Saxon potentials for both real and imaginary volume parts. The comparisons between the experimental data at energies (13.0, 20.0, 25.7, 30.0, 32.0, 36.0, 48.0, and 50.0 MeV) and the theoretical calculations using OM are shown in Figs. 1-2. The optimal extracted potential parameters are listed in Table I.

Figure 1 Comparison between experimental angular distributions (solid black circles) for 6Li + 16O elastic scattering and the theoretical calculations (solid red curves) using OM at E lab = 13:0, 20.0, 25.7, and 30.0 MeV.

Figure 2 Comparison between experimental angular distributions (solid black circles) for 6Li + 16O elastic scattering and the theoretical calculations (solid red curves) using OM at E lab = 32:0, 36.0, 48.0, and 50.0 MeV.
Table I Global optical potential parameters for 6Li + 16O nuclear system extracted from the OM analysis, the values of reaction cross-sections σ R as well as real J V and imaginary J W volume integrals are also listed.
E | V0 | r V | a V | W 0 | r W | a W | χ2/N | σ R | JV | JW |
(MeV) | (MeV) | (fm) | (fm) | (MeV) | (fm) | (fm) | (mb) | (MeV.fm3) | (MeV.fm3) | |
13.0 | 186.01 | 1.37 | 0.719 | 7.14 | 1.88 | 0.949 | 7.4 | 1183 | 477.0 | 46.3 |
20.0 | 185.87 | 1.37 | 0.666 | 9.11 | 1.88 | 0.93 | 8.1 | 1288 | 456.3 | 58.3 |
25.7 | 183.13 | 1.37 | 0.716 | 11.23 | 1.88 | 0.93 | 11.5 | 1438 | 468.5 | 71.9 |
30.0 | 180.06 | 1.37 | 0.734 | 11.65 | 1.88 | 0.93 | 16.6 | 1477 | 467.6 | 74.6 |
32.0 | 182.15 | 1.37 | 0.721 | 12.59 | 1.88 | 0.93 | 7.5 | 1496 | 467.9 | 80.6 |
36.0 | 182.46 | 1.37 | 0.701 | 13.64 | 1.88 | 0.93 | 17.9 | 1513 | 460.9 | 87.4 |
48.0 | 188.0 | 1.37 | 0.664 | 14.09 | 1.88 | 0.95 | 16.8 | 1530 | 460.8 | 91.3 |
50.0 | 185.6 | 1.37 | 0.708 | 16.25 | 1.88 | 0.862 | 5.2 | 1439 | 471.6 | 100.0 |
We have investigated the energy dependence on the values V 0 and W 0 for the 6Li + 16O system, as shown in Fig. 3 and can be approximated by: V 0 (MeV) = 183.06 + 0.0346E (MeV), and W 0 (MeV) = 5.035 + 0.218E (MeV). The available experimental data for 6Li+16O are then analyzed within the framework of DFOM; the data were fitted using only one parameter “N R ” while the imaginary phenomenological potential parameters were taken the same as those obtained from OM calculations. The comparisons between the experimental angular distributions data for 6Li + 16O nuclear system at the different concerned energies and theoretical calculations using this model are shown in Figs. 4-5. It is clear that using such hard constraints, the quality of fitting got worse “see the values of χ 2/N extracted from the OM and DFOM calculations”. The data analysis using DFOM showed and emphasized the necessity for reduction N R by about 40%. The optimal potential parameters used in the DFOM calculations are listed in Table II.
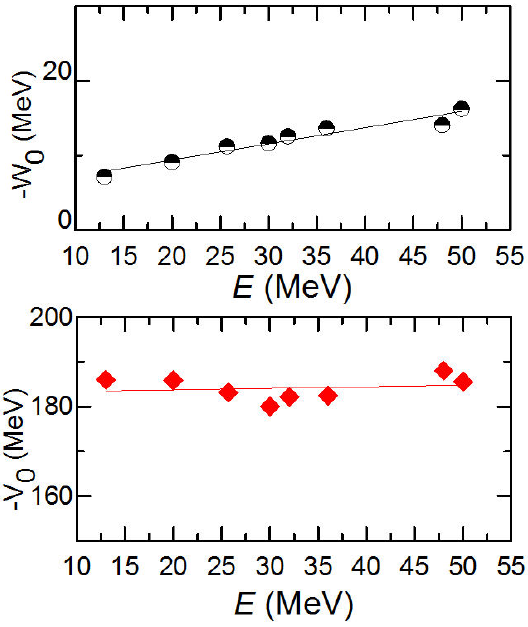
Figure 3 Energy dependence on the real and imaginary potential depths extracted from the OM calculations.
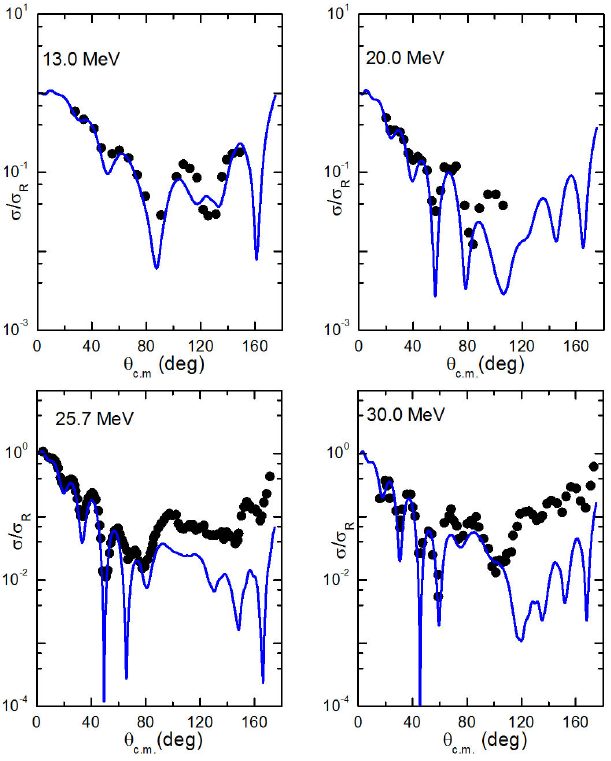
Figure 4 Comparison between experimental angular distributions (solid black circles) for 6Li + 16O elastic scattering and the theoretical calculations (solid blue curves) using DFOM at E lab = 13:0, 20.0, 25.7, and 30.0 MeV.

Figure 5 Comparison between experimental angular distributions (solid black circles) for 6Li + 16O elastic scattering and the theoretical calculations (solid blue curves) using DFOM at E lab = 32:0, 36.0, 48.0, and 50.0 MeV.
Table II Potential parameters for the 6Li + 16O system extracted from the DFOM analysis, the values (σ R , J V and J W ) are also listed
E | N R | W 0 | r w | a w | χ2/N | σ R | J V | J W |
(MeV) | (MeV) | (fm) | (fm) | (mb) | (MeV.fm3) | (MeV.fm3) | ||
13.0 | 0.795 | 7.14 | 1.88 | 0.949 | 12.7 | 1237 | 403.9 | 46.3 |
20.0 | 0.584 | 9.11 | 1.88 | 0.93 2 | 4.3 | 1354 | 293.7 | 58.3 |
25.7 | 0.584 | 11.23 | 1.88 | 0.93 | 38.0 | 1449 | 291.3 | 71.9 |
30.0 | 0.594 | 11.65 | 1.88 | 0.93 | 42.6 | 1475 | 294.5 | 74.6 |
32.0 | 0.587 | 12.59 | 1.88 | 0.93 | 16.0 | 1498 | 290.2 | 80.6 |
36.0 | 0.522 | 13.64 | 1.88 | 0.93 | 30.1 | 1511 | 256.6 | 87.4 |
48.0 | 0.546 | 14.09 | 1.88 | 0.95 | 62.2 | 1543 | 263.7 | 91.3 |
50.0 | 0.555 | 16.25 | 1.88 | 0.862 | 21.3 | 1473 | 267.3 | 100.0 |
The elastic scattering data for 6Li + 16O system plotted as a function of momentum transfer (Fig. 6) showed that the interference peaks and valleys line up whereas, if we plotted the data as a function of angle there is no apparent pattern.
We used the following formula: q = 2ksin(θ c.m. /2) for calculating momentum transfer, where k is the wavenumber. As shown in Fig. 6, the plotted data as a function of momentum transfer exhibit repeated dips at momentum transfers around q = 0.63, 1.4, and 1.6 fm-1. Consequently, we should be able to show that the forward angle dips are produced by the same potential “real part”; and the differences between the cross-sections at different energies at larger momentum transfer (angles) are due to the differences in the absorption at the larger values.
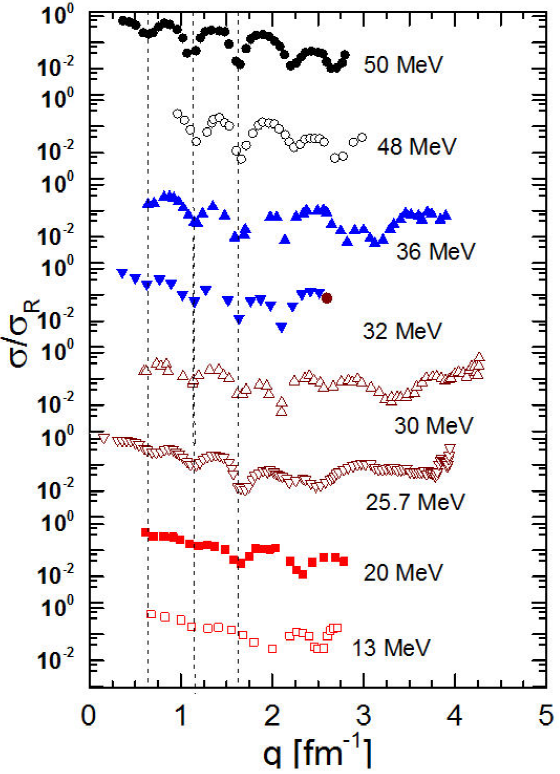
Figure 6 Experimental angular distributions for 6Li + 16O system plotted as a function of momentum transfer at energies E lab = 13:0, 20.0, 25.7, 30.0, 32.0, 36.0, 48.0, and 50.0 MeV.
The calculated CF potential discussed in Sec. 2 was then used to fit the experimental 6Li + 16O elastic scattering angular distributions using two approaches. In the first approach, namely, “CF1”, the real part derived besed on CF and an imaginary potential -which was taken the same as those obtained from OM analysis- were used to reproduce the experimental data. The data analysis using the CF1 approach was performed only with one adjustable parameter N RCF “renormalization factors for the real part of CF potential”; the potential thus had the following form:
The comparisons between the experimental angular distributions data for 6Li + 16O nuclear system at the different concerned energies and theoretical calculations using this CF1 approach are shown in Figs. 7-8. As shown in Table III, the value of N RCF ranges between 1.01 and 1.05, and it is weakly dependent on bombarding energy.

Figure 7 Comparison between experimental angular distributions (solid black circles) for 6Li + 16O elastic scattering and the theoretical calculations (solid red curves) using CF1 approach at E lab = 13:0, 20.0, 25.7, and 30.0 MeV.

Figure 8 Comparison between experimental angular distributions (solid black circles) for 6Li + 16O elastic scattering and the theoretical calculations (solid red curves) using CF1 approach at E lab = 32:0, 36.0, 48.0, and 50.0 MeV.
Table III Potential parameters for 6Li + 16O system extracted from CF1 approach, the values (σ R , J V and J W ) are also listed.
E | N R | W 0 | r w | a w | χ2/N | σ R | J V | J W |
(MeV) | (MeV) | (fm) | (fm) | (mb) | (MeV.fm3) | (MeV.fm3) | ||
13.0 | 1.05 | 7.14 | 1.88 | 0.949 | 18.3 | 1121 | 471.3 | 46.3 |
20.0 | 1.03 | 9.11 | 1.88 | 0.93 | 7.6 | 1310 | 462.4 | 58.3 |
25.7 | 1.04 | 11.23 | 1.88 | 0.93 | 12.8 | 1421 | 466.8 | 71.9 |
30.0 | 1.01 | 11.65 | 1.88 | 0.93 | 20.2 | 1448 | 453.4 | 74.6 |
32.0 | 1.02 | 12.59 | 1.88 | 0.93 | 15.4 | 1481 | 457.9 | 80.6 |
36.0 | 1.03 | 13.64 | 1.88 | 0.93 | 20.5 | 1511 | 462.4 | 87.4 |
48.0 | 1.05 | 14.09 | 1.88 | 0.95 | 42.2 | 1545 | 471.3 | 91.3 |
50.0 | 1.05 | 16.25 | 1.88 | 0.862 | 7.8 | 1477 | 471.3 | 100.0 |
In the second approach, namely, “CF2”, the data were reproduced fully microscopically using only two adjustable parameters N RCF and N ICF “renormalization factors for the real and imaginary parts of CF potential”; the potential thus had the following form:
The variation in cluster folding potential for both the real and imaginary parts with radius is shown in Fig. 9. The optimal values for N RCF and N ICF at different concerned energies were obtained by minimizing χ 2 using SFRESCO, the searching version of FRESCO. The best fit values are listed in Table IV. The comparisons between the experimental data and the theoretical calculations using the CF2 approach at different concerned energies are shown in Figs. 10-11. The data analysis using the CF2 approach also showed that the value of N RCF is weakly dependent on energy and ranges between 1.03 and 1.06, while N ICF is strongly dependent on energy (Table IV). In other words and as shown in Fig. 6, the data at forwarding angles could be reproduced by the same potential “real part”; and the differences between the cross-sections at different energies and larger angles are due to the differences in the absorption at the larger values.
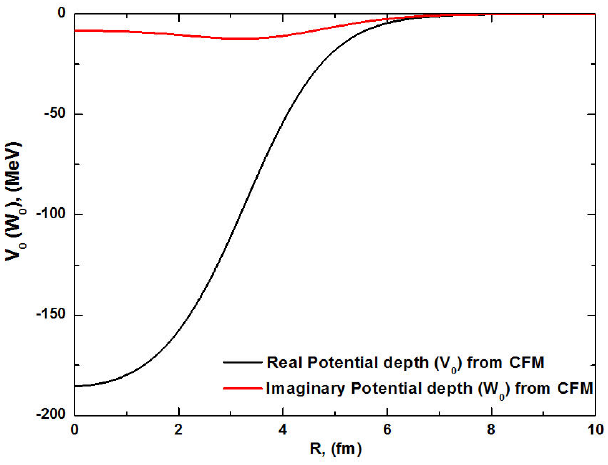
Figure 9 Variation in the real (V 0) and imaginary (W 0) potential depths derived from CF Model at different radii.

Figure 10 Comparison between experimental angular distributions (solid black circles) for the 6Li + 16O elastic scattering and the theoretical calculations (solid black curves) using CF2 approach at E lab = 13:0, 20.0, 25.7, and 30.0 MeV.
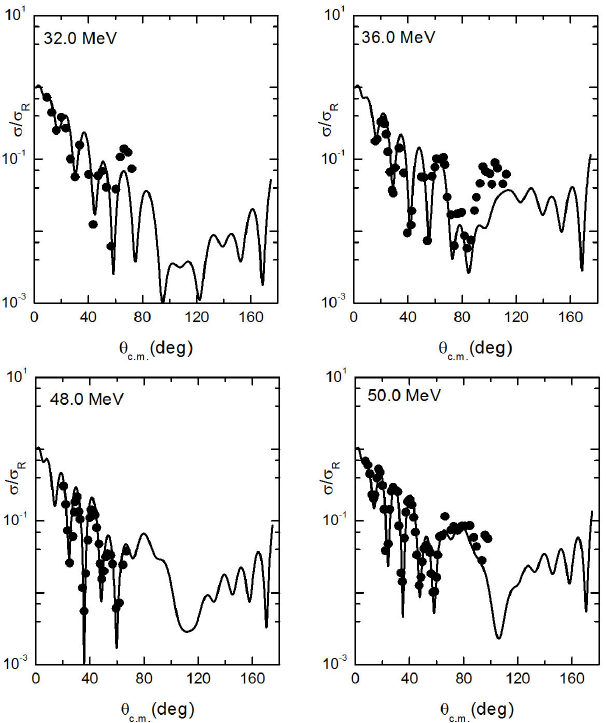
Figure 11 Comparison between experimental angular distributions (solid black circles) for the 6Li + 16O elastic scattering and the theoretical calculations (solid black curves) using CF2 approach at E lab = 32:0, 36.0, 48.0, and 50.0 MeV.
Table IV Potential parameters for 6Li + 16O nuclear system extracted from CF2 approach, the values (σ R , J V and J W ) are also listed.
E | N RCF | N ICF | χ2/N | σ R | J V | J W |
(MeV) | (mb) | (MeV.fm3) | (MeV.fm3) | |||
13.0 | 1.06 | 0.58 | 15.9 | 972.5 | 475.8 | 48.5 |
20.0 | 1.04 | 0.75 | 11.1 | 1186 | 466.8 | 62.8 |
25.7 | 1.06 | 0.92 | 25.5 | 1279 | 475.8 | 77.0 |
30.0 | 1.03 | 0.92 | 31.5 | 1298 | 462.4 | 77.0 |
32.0 | 1.06 | 1.3 | 39.7 | 1375 | 475.8 | 108.8 |
36.0 | 1.03 | 1.15 | 60.1 | 1356 | 462.4 | 96.2 |
48.0 | 1.05 | 1.33 | 47.7 | 1397 | 471.3 | 111.3 |
50.0 | 1.05 | 1.27 | 14.7 | 1477 | 471.3 | 106.3 |
4. Summary
The available experimental data for 6Li + 16O elastic scattering angular distributions in the energy range of 13.0-50.0 MeV were reanalyzed within the framework of different potentials: OM, DFOM, and CF potentials. The comparisons between the experimental data and theoretical calculations using the different concerned models were found to be fairly good. The data analysis using DFOM showed that the potentials needed to be reduced in strength by about 40%. This fact was also previously reported for various 6Li +X nuclear systems [7]. The available data for 6Li + 16O system were plotted as a function of momentum transfer. In this case, the data exhibited repeated dips at momentum transfers at q = 0.63, 1.4, and 1.6 fm-1. This means that the forward angle dips should be reproduced by the same potential “real part” and the differences between the cross-sections at different energies and larger angles are due to the differences in the absorption at the larger values. In the present work, we carried out further microscopic analysis of the 6Li + 16O elastic scattering based on the CF potential. We hope to minimize what are probably the largest sources of the model dependence of the extracted renormalization factor for this system. The renormalization factors of the real and imaginary parts of the CF optical potential were tuned to give the best agreement with the elastic scattering data. The data analysis using CF1 and CF2 approaches showed that the value of N RCF is about 1.0, and it is weakly dependent on energy.