1. Introduction
Vegetable oils have raised its interest due to the broad applications variety, uses as quenchants, biodiesel precursor, lubricant additives, among others [1-4]. The therapeutical properties of vegetable oils have been known since ancient times. In recent years, the use of vegetable oils in the cosmetic industry has been raised. Therefore, there are opportunities for development and innovation for the cosmetic sector keeping in mind the growing concern for personal well-being, the prevention of aging and a population with higher disposable income that prefers natural cosmetic products in which there is also a contribution to health and well-being. Also, it is necessary to understand more in deep its functionality to offer to final consumer more safer products. Nowadays it is common finding vegetable oils and vegetable derivatives in several cosmetic products. The use of vegetable products as starting materials for cosmetics has several advantages as biocompatibility, inherent stability, skin absorption, among others. Also, vegetable oils can be considered as an ecofriendly commodity for cosmetic industry [5,6]. Complex esters can be obtained from vegetable oils by transesterification from fatty accids [7]. Esters derived from vegetable oils can effectively increase the skin protection against sunlight, rise the skin hydration [8,9]. Esters can also serve as carriers of functional nanostructures [10] and precursors of drug-delivering systems such as liposomes [11]. Lacatusu and coworkers [12], stat the importance of oils rich in ω-fatty acids, especially linoleic and oleic acids indicating that the higher content in ω-6 and ω-9 fatty acids led to a beneficial emollient and regenerative skin action, and rejuvenating effect on the skin and an increased hydration effect. The skin protection rise due to vegetable oils is one of the main reasons for using vegetable oils in the cosmetic industry [10].
The main component of the vegetable oils is fatty acids. Its chemical structure is composed by a three-carbon backbone and attached to each carbon a long hydrocarbon chain. The differences among different vegetable oils are mainly due to the length of the hydrocarbon chain and the number of carbon-carbon double bonds on the chain, and the percentage of each fatty acid in the oil composition [13-16].
A huge analytical methods have been developed to determine the composition and some of the physicochemical properties of the oils such as Gas Chromatography coupled with Mass Spectrometry (GC-MS), Differential Scanning Calorimetry (DSC), Nuclear magnetic resonance (NMR) , acidity, Iodine Index, peroxide index, triglyceride profile by HPLC, Free fatty acids, Antioxidants content, Amount of solids, Melting temperature, Crystallization temperature, among others in order to guarantee the product quality [17]. But it is necessary to develop new, and more accurate measurement methods not only to assure the product quality, also to understand the effect of the molecular interactions in order to develop new products.
The properties such as melting point are affected by the length and degree of unsaturation of the carbon chain of fatty acids, also depends on whether the chain is even- or oddnumbered; the latter have higher melting points [16]. Also, the thermophysical properties are sensitive to the change of the molecular interactions [18]. Therefore, the thermal properties knowledge can give light in understanding from a thermal point of view of possible interactions between vegetable oils present in diverse cosmetic formulation and the human skin.
On the other hand , in different oil industrial scenarios the understanding of the thermophysical properties is important because, it is also required to design the equipment or control systems for the oil production, transport, and packaging process [19]. Also, for the utilization of such oils as sources of raw materials for diesel, food, pharmaceutical or cosmetic production as it was mentioned above.
Thermophysical properties can be divided into dynamic and static parameters being, static parameters like thermal conductivity the most reported because they are relatively easy to measure [20,21]. Thermal effusivity and thermal diffusivity are dynamic thermal parameters. On the other hand, the photopyroelectric methods have been proven suitable for measuring dynamic thermal parameters [20]. Thermal effusivity gives the sample thermal impedance; thus, it is an indicator of heat transition behavior between two objects in direct contact. Thermal effusivity is of particular interest when analyzing the heat exchange between two different materials as the case of moisturizing oil when contacting the skin.
In this work, photopyroelectric techniques are used obtaining thermal effusivity and thermal diffusivity of wheat germ, mamey seed, walnut, coconut, and linseed oils. Information was complemented by measuring density and calculating thermal conductivity; thus; an entirely thermal characterization is achieved.
2. Materials and Methods
2.1. Samples
Studied samples were obtained from a local supplier (F. Paris®, Merck, CDMX, Mexico,) all of them with 99% purity. It must be pointed out that the physicochemical properties of vegetable oils are affected by agro-climatic conditions as well as soil properties. Therefore, some variations can be found among the sample type [22]. Lipid oxidation is responsible for changing the organoleptic oil properties, the required amount for changing these properties is relatively low but, changes in bulk properties requires a considerable sample amount oxidation for taking place [23]. Main lipid autoxidation initiators are transition metal ions, certain enzymes and UV light [23,24]. Therefore, the studied samples were kept in the dark containers for avoiding light interaction and the consequent autoxidation.
The composition of the oil used in this study, according to the literature, is presented in Table I.
TABLE I Lipid profile for the oils used in this study.
Fatty acid Composition Wt (%) | ||||||||||||
Oil | C6:0 | C8:0 | C12:0 | C14:0 | C16:0 | C16:1 | C18:0 | C18:1 | C18:2 | C18:3 | C20:0 | Reference |
Linseed | - | - | - | - | 6.68 | - | 4.43 | 18.5 | 17.3 | 53.2 | - | [13] |
Mamey | - | - | - | - | 10.7 | - | 26.5 | 53.5 | - | 5.6 | - | [14]] |
Walnut | - | - | - | - | 7.2 | 0.2 | 2.7 | 17.6 | 57.1 | 11.9 | 0.1 | [15,25]] |
Wheat germ | - | - | - | 0.1 | 15.9 | 0.2 | 0.6 | 11.1 | 52.7 | 7.1 | 0.3 | [25]] |
Coconut | 0.52 | 7.6 | 50 | 19.9 | - | - | 2.7 | 6.2 | - | - | - | [25]] |
The main difference between the studied oils from the chemical composition point of view is the quantity of saturated and unsaturated fatty acids.
2.2. Density
An electronic balance (Shuler Scientific, SAS-225.R) and a calibrated Gay Lussac pycnometer of 25 ml (Kimble, 15123N-25) were used to obtain the weight and volume of all samples; measurements were performed at 25◦C.
2.3. Thermal diffusivity
Thermal diffusivity was obtained using the so-called thermal wave resonator cavity (TWRC). In the TWRC experimental setup, the sample under study is enclosed between a pyroelectric detector and a modulated heat source (Fig. 1). When the distance between the heat source and the pyroelectric slab is varied, the pyroelectric signal is a function of the distance between detector and heat source. The mentioned distance is at the same time, the sample thickness l s and takes the form [25]:
where A is an instrumental factor, η s is the nonradiative conversion efficiency for the absorbing sample, κ p is the thermal conductivity of the pyroelectric sensor, b sp = e s /e p , and ω 0 the angular frequency of the laser beam (ω 0 = 2πf).
When the modulation frequency is fixed, the pyroelectric signal depends on the α s l s product and an instrumental constant, then by performing a sample thickness scan the, sample thermal diffusivity can be obtained.
2.4. Thermal effusivity
In the inverse photopyroelectric (IPPE) configuration, the sample is placed in direct contact with the pyroelectric detector, Fig. 2. The modulated light strikes on the opposite side of the pyroelectric detector. In this experimental configuration and assuming the sample thickness is enough to be considered as thermally thick (i.e., l s >> µ s ) being µ s the sample thermal diffusion length, the pyroelectric detector output can be expressed as [26]:
where σ
p
is the complex thermal diffusion coefficient (σ
p
= (1 + j)/µ
p
, j = (−1)
1/2
and µ
p
= (α
p
/(πf))
1/2
), with α
p
the pyroelectric thermal diffusivity), l
p
is the pyroelectric thickness, b = e
s
/e
p
, g = e
g
/e
p
and e
s
, e
g
and e
p
, are thermal effusivities for sample, surrounding gas and pyroelectric detector, respectively. Using the relationship
3. Results
Figure 3 shows the TWRC results; symbols represent the experimental results, and solid lines are the best fit using Eq. (1) for each data set.

FIGURE 3 TWRC results (phase of the pyroelectric signal as a function of the sample thicknesses), experimental signals are represented by symbols: circle coconut, square linseed, tringle mamey seed, rhombi walnut, star wheat germ, the solid line represents de best fitting of the Eq. (1) to the experimental data of each sample.
As it can be shown in Fig. 3, all the samples present an inverse trend between phase and sample thickness. Coconut oil present the lowest phase of the pyroelectric signal, behavior associated with the high presence of C:12 fatty acids (Table I).
The IPPE signals are presented in Fig. 4, by fitting the Eq. (2) to the experimental data (symbols), thermal effusivity values were obtained. In this case, according to the IPPE signal for mamey seed oil evidence a less increase of the amplitude as the frequency increase. This behavior was associated with the presence of high levels of C18:0, and C:18:1 fatty acid (Table I)
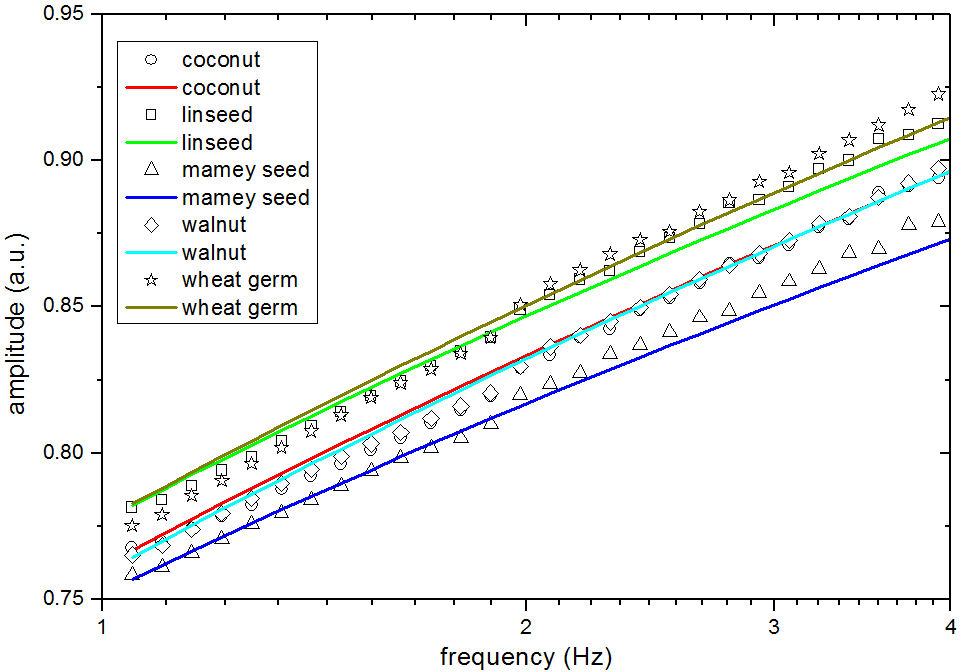
FIGURE 4 Amplitude of the IPPE signal as a function of the frequency, experimental signals are represented by symbols: circle coconut, square linseed, tringle mamey seed, rhombi walnut, star wheat germ, the solid line represents de best fitting of the Eq. (1) to the experimental data of each sample.
The summary of obtained thermal effusivity, thermal diffusivity, and thermal conductivity are shown in Table II. Also, in this table, the carbon number and the percentage of the main constituents of oils were added for comparison purpose.
TABLE II Thermal properties of the studied samples, thermal conductivity values were calculated.
Sample | Density (Kg/m3) ×102 |
Thermal Diffusivity (m2/s) ×108 |
Thermal Effusivity (Ws1/2/m2K)×102 |
Thermal Conductivity (calculated) W/mK ×10−1 |
The carbon # of the main constituent* |
Total % of the main constituent* (%)* |
Saturated fatty acids |
Unsaturated Fatty acid (%)* |
Linseed | 8.90±0.01 | 7.89±0.06 | 5.41±0.05 | 1.51±0.14 | 16 18 |
6.6 93.4 |
11 | 89 |
Mamey seed | 8.91±0.01 | 8.75±0.06 | 5.40±0.07 | 1.59±0.21 | 16 18 |
10.7 85.6 |
37.2 | 59.1 |
Walnut | 9.07±0.01 | 9.02±0.05 | 5.84±0.05 | 1.75±0.15 | 16 18 |
7.4 89.3 |
9.9 | 86.8 |
Wheat germ | 9.13±0.01 | 8.86±0.05 | 5.69±0.06 | 1.71±0.18 | 16 18 |
16.1 71.5 |
16.5 | 71.1 |
Coconut | 8.77±0.01 | 7.29±0.06 | 5.69±0.04 | 1.53±0.11 | 12 14 18 |
50.0 19.9 2.7 |
80.7 | 6.2 |
∗Theoretical values according to Table I.
The thermal diffusivity increases as the unsaturated fatty acid increase in the oil composition (Table II), following the trend coconut< Mamey seed< Wheat germ< Walnut oils except for linseed oil. For the other hand, the thermal effusivity for Mamey seed, Wheat germ, and Walnut oils increase following the trend as for the thermal diffusivity. The Coconut and linseed oil depart from this trend. Deviations between the different data sets can be associated with the purity, plant variety, industrial processing as it was reported by Werner et al., [19].
From the molecular point of view, the geometrical arrangement of the fatty acids within the triglyceride molecule affect the molecule shape [19]. Also, the intermolecular attractive/repulsive forces due to the polar group and the presence of the van der Waals forces between the long molecule chains affects the diffusive contribution to the heat flux due to the diminished of the intermolecular vibration because of the steric hindrances. Therefore, it is expected that the thermal diffusivity increases as the unsaturation and the length of the fatty acid increase.
The thermal effusivity results obtained in this work are similar to those reported for olive, and avocado, in vegetable oils by Balderas et al. [27], Lara et al. [28]. The calculated thermal conductivity is in the range of all values reported by Balderas and coworkers [27].
4. Conclusions
In this work, a full thermal characterization was achieved for the studied samples; the obtained results are close to other vegetable oils like olive or avocado, previously reported. The vegetable oils thermal properties present similar values; despite those values strongly depend on the origin region, the overall bulk thermal properties remain close for similar samples, type of sample, and management processes. Because all studied samples have similar thermal properties, it is hard to distinguish any oil species only by its thermal properties. By obtained thermal values, more knowledge is gain about oils behavior.