PACS: 61.50.Nw; 61.05.cp; 68.37.Hk; 82.80.Pv; 78.40.-q; 76.30.-v
1. Introduction
In the course of recent decades, there has been much interest in vanadium oxides because of their rich crystal chemistry 1, they show some basic solid state phenomena such as; metal-insulator transitions 2, Spin-Peierls transitions 3, doping promoted ferromagnetism in vanadium oxide nanotubes (VONT’s) 4; more recently, novel technical applications based on these compounds have been reported 5,6. On the other hand, GeO2 exhibits many interesting physicochemical properties, such as photoluminescence, high values of dielectric constant and refractive index, good thermal stability and mechanical strength which make it suitable for applications in optical, electronic and optoelectronic devices 7,8,9,10,11, in particular, as transparent glasses their structural and specific optical properties, make it a valuable candidate for the construction of planar waveguides for optoelectronic communications 12,13,14.
As mixed oxides, the V2O5 and the GeO2 have been synthesized as vitreous materials for several years and their physical and chemical properties have been investigated systematically 15,16,17. Later, amorphous coatings of mixtures of these oxides were prepared by the route of sol-gel synthesis and characterized using different spectroscopic techniques 18,19. Although there is published work related to Ge-V-O based crystalline compounds (see 20,21 and references therein), the information on crystalline solid solutions of type Ge(
In this paper we present the characterization of the nonstoichiometric Ge0.74V0.21 □0.05O2 compound synthesized by our group some years ago 22. The characterization was performed through the following analysis techniques: X-ray diffraction spectroscopy (XRD), scanning electron microscopy (SEM), X-ray photoelectron spectroscopy (XPS), diffuse reflectance ultraviolet-visible spectroscopy (DRUV-VIS) and electron spin resonance (ESR) spectroscopy.
2. Experimental Details
The Ge0.74V0.21 □0.05O2 polycrystalline compound was prepared by conventional solid-state reactions, starting from quartz-type GeO2 (Cerac 99.999%) and V2O5 (J.T. Baker 99.7%). To set up the phase transition of q-GeO2 to r-GeO2, we employ the K2CO3 (J.T. Baker 99.9%) reactive as a catalyst. The educts were mixed and ground in an agate mortar in order to obtain a fine powder, which was placed on a platinum crucible and then heated in a furnace in air at 1123 K for two days. At the end of the heat treatment, a solidified mixture of crystalline and amorphous phases was obtained. The amorphous component was easily removed by dissolution, stirring and washing the mixture wth hot distilled water.
The X-ray powder diffraction measurements were performed on a Bruker D8 Advance Diffractometer: Cu K
al
radiation, and a goniometer with a lynx-eye detector. The data were collected from
The XPS measurements were performed at ultra-high vacuum conditions in a Thermo-VG SCALAB 250 system equipped with a monochromatic AlK
3. Results
After the washing process described above, the “beige” recovered powders (Fig. 1) were subjected to an X-ray diffraction analysis. The Rietveld refinement of the X-ray pattern (see Fig. 2), clearly matches with that of the crystalline model previously reported by Rosales et al. 22 for the rutile-type compound Ge0.74V0.21 □0.05O2. In that compound, some percentage of Ge4+ is replaced by V5+ ions and the charge neutrality is accomplished by the presence of cation vacancies. On the other hand, SEM images show that the synthesized powder consists of long faceted rectangular crystallites (Fig. 3), a shape frequently observed on rutile type nano-crystals grown by sol-gel methods in alkaline environments 23.

Figure 2. Experimental X-ray pattern obtained in the recovered microcrystalline powders compared with that reported by Rosales et al. 22.
In many compounds based on vanadium oxides it is very common to find the vanadium ion in different oxidation states. This leads to the well known chromophoric effect observed in both, glassy and crystalline materials 24,25, then, the “beige” color observed in the recovered powders, is probably caused by combined oxidation states of vanadium ions hosted in the crystal lattice. So, to determine the oxidation state of vanadium we perform an analysis of powders by XPS.
The XPS spectra we obtained for this compound in the binding energy interval 510-540 eV, shows the characteristics Vp3/2, Vp1/2, Os1 bands assigned to vanadium and oxygen (Fig. 4). A detailed analysis through the deconvolution of the band corresponding to Vp3/2 zone (Fig. 5) clearly shows that this consists of two peaks located at 516 eV and 517 eV which have been assigned to V4+ and V5+ ions respectively 26, so we can assert that this two types of vanadium ions are present, at least, in the first atomic layers of synthesized microcrystals if we consider that the XPS is a surface analysis technique. Additionally, DRUV-VIS spectra taken on the recovered powders show broad absorption bands in the interval of 200 to 800 nm, which invariably can be matched by six Gaussian-shape bands centered at 230, 310, 400, 505, 620 and 751 nm (see Fig. 6). In this spectral region charge-transfer (C-T) and d-d transition bands of the V5+ and V4+ ions hosted in based vanadium oxide compounds have been reported 27.

Figure 5. Fitting of V2p3/2 band with two peaks assigned to V4+ and V5+ oxidation states of vanadium.

Figure 6. DRUV-VIS spectrum (displayed in absorbance) of recovered powders of the Ge0.74V0.21 □0.05O2 compound.
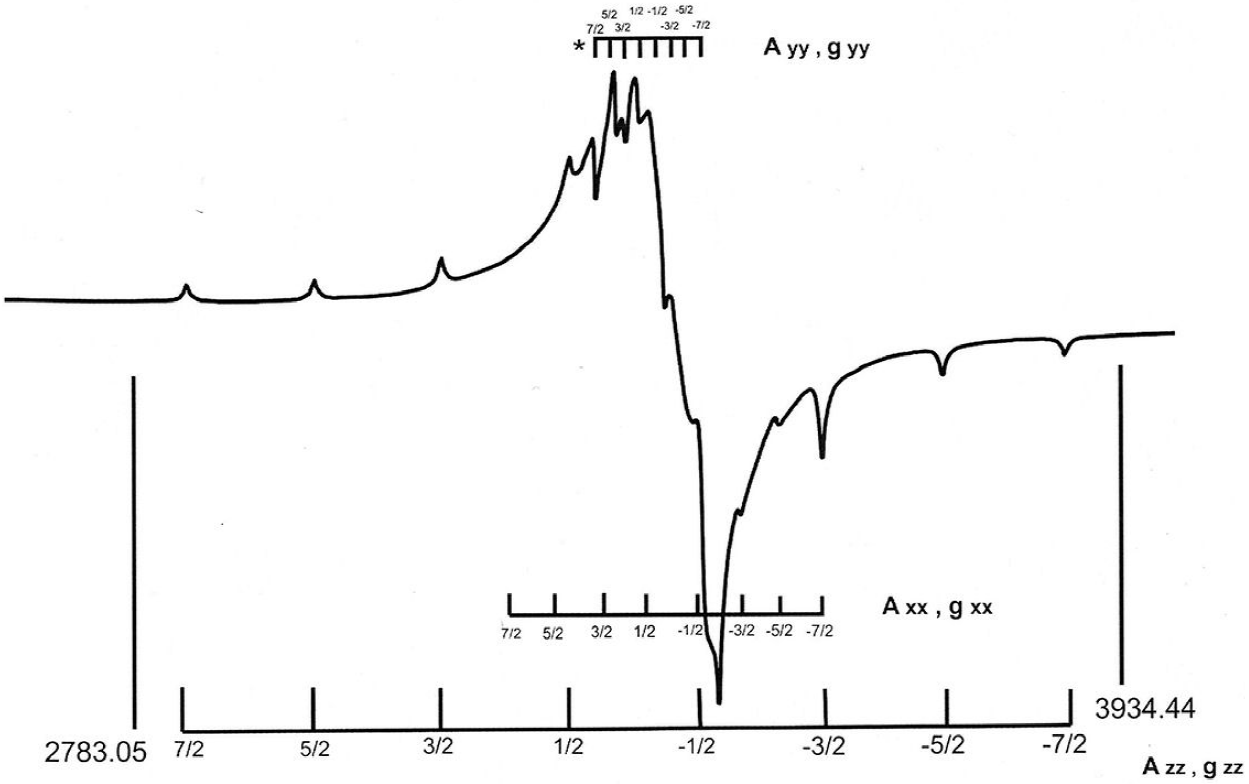
Figure 7. ESR spectrum taken on the synthesized powders of the compound Ge0.74V0.21 □0.05O2 compound.
On the other hand, it is well known that some physical properties of compounds based on vanadium oxide such as electronic conductivity and magnetic susceptibility are linked with the presence of vanadium ions in mixed oxidation states (as an example see Ref. 28), so to understand the physical processes that give rise to these properties, it is first necessary to determine the crystalline sites occupied by the different vanadium ions.
As we saw, the XPS measurements performed on the nonstoichiometric Ge0.74V0.21 □0.05O2 compound detected the presence of V4+ ions hosted, at least, in the first layers of crystallites. Taking into account that V4+ is a paramagnetic ion, from ESR measurements it is possible to obtain information about the environment of the crystalline sites occupied by these ions trough the analysis of the spin Hamiltonian data.
The paramagnetic V4+ ion has the 3d1 electronic configuration and an electronic spin S = 1/2, and a nuclear spin for its V 51 isotope (natural abundance 99.5%) of I = 7/2, then an eight component hyperfine structure is expected in the ESR spectra of synthesized compound.
In this case, we used a full spin Hamiltonian to describe the spectra of V4+:
where
The spin Hamiltonian parameters deduced from computer simulation for the three sets of eight hyperfine transitions are: The parallel site to the z axis, has a hyperfine splitting A
zz
= 146.54 gauss with a
The ESR spectra of V4+ ions at crystalline sites with this kind of ligand symmetry are characterized by Aiso and giso values in (60-90) and (1.920-1.95) intervals respectively 29. In our case, the calculated Aiso (69.02) and giso (1.945) values fall within these ranges, and are very similar to those reported in a pioneering research related to a paramagnetic resonance in polycrystalline and single crystals of rutile-type GeO2 slightly doped with V4+ ions 30,31.
Finally, following the quantification procedure described in the experimental section, the V4+/Vtotal proportion was estimated as 0.002.
4. Discussion
To prepare the polycrystalline Ge0.74V0.21 □0.05O2, we employed K2CO3 as flux and catalyst. This carbonate decomposes in K2O and CO2 at temperatures reached in our synthesis procedure, then, the microcrystals were grown from the melted mixtures of quartz-type GeO2 and V2O5 in an alkaline ambient and in a reducing atmosphere. This would account for the shape of crystallites obtained, as in the case of sol-gel methods of synthesis of rutile nanoparticles in alkaline environments 23 and for the reduction of V5+ to V4+, which diffuses in small quantities into crystallite lattice, as we detected by ESR.
The deconvolution of the XPS spectra taken on recovered powders of synthesized compound, invariably shown the presence of vanadium as V5+ and V4+ ions, whose electronic configuration are [Ar] 3d0 and [Ar] 3d1 respectively. It is known that the V4+ reacts readily to form some oxy-cations such as the VO2+, this molecular oxy-cation can be thought as consisting of a V4+ (3d1) ion and a closed shell O2- ion. The VO2+ ion has a single d electron, which gives raise the free ion term 2D. In a crystal field of octahedral geometry, this electron occupies the
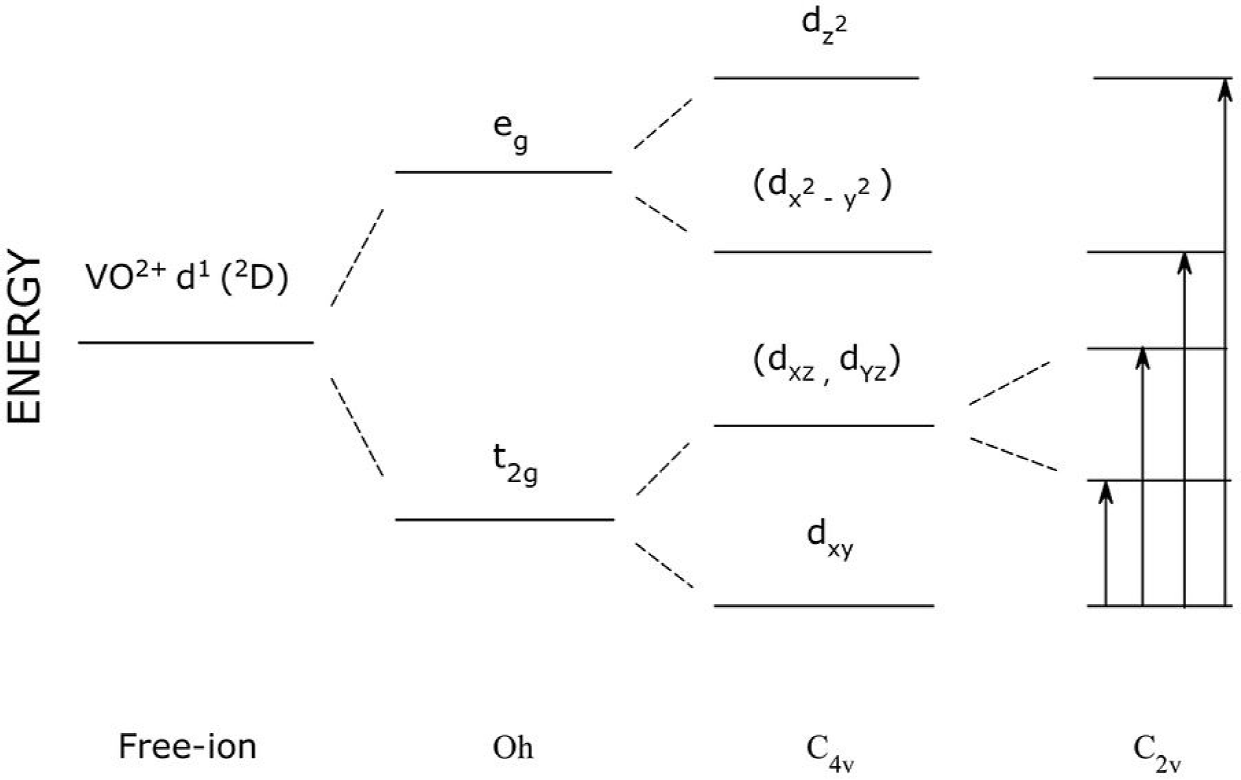
Figure 8. Diagram of energy levels with the assignment of the optical transitions of VO2+ in the symmetry C2V.
As we see, the absorption spectra taken in the Ge0.74V0.21 □0.05O2 compound we synthesized, invariably can be adjusted with four bands in the region of d-d transitions of the V4+ ion. This result could indicate that this ion is hosted as VO2+ oxy-cation with a (C 2v ) rhombic symmetry in the crystal lattice of the rutile-type Ge0.74V0.21 □0.05O2 compound. Moreover, a ESR spectrum with three sets of hyperfine lines are expected for VO4+ in a (C 2v ) symmetry 27, just as we from our ESR measurements on the Ge0.74V0.21 □0.05O2 compound. Similar absorption bands and ESR signals have been reported for the VO2+ cation hosted in sites of (C 2v ) symmetry on CsCl single crystals 32.
In a subsequent paper we will make a detailed theoretical analysis of our ESR and DRUV-VIS results.
5. Conclusions
The characterization of microcrystalline powders of the Ge0.74V0.21 □0.05O2 by scanning electron microscopy (SEM), X-ray photoelectron spectroscopy (XPS), diffuse reflectance ultraviolet-visible spectroscopy (DRUV-VIS) and electron spin resonance spectroscopy (ESR) let us conclude:
The synthesized powders of compound Ge0.74V0.21 □0.05O2 grow as long faceted rectangular micro-crystals.
In these micro-crystals, the Ge4+ ions are partially replaced by V5+ and V4+, the latter, to a lesser extent as deduced from the ESR measurements.
The detection of four absorption bands on the DRUV-VIS spectra in a region of d-d transitions of ion V4+ indicates that this ion possibly is hosted in the lattice of the micro-crystallites as the VO2+ oxy-cation.
The three sets of hyperfine lines obtained in the ESR spectra and the four bands of the DRUV-VIS spectra, points out that the symmetry of crystalline sites occupied by VO2+ cations must be the rhombic (C 2v ).