INTRODUCTION
Clinicians have long identified the relationship between excessive alcohol use and impairment of the organism's defense systems. For example, in 1884, Robert Koch found a higher mortality rate among individuals who drank alcohol during the cholera epidemic and concluded that it was due to a depression of the immune system (IS)1. In a 1913 publication, William Osler noted that "alcoholism is perhaps the most potent predisposing factor" in pneumococcal pneumonia2.
Moreover, the results of prospective and retrospective epidemiological studies carried out during the 20th century provided the first evidence that alcohol consumption is a risk factor for various types of cancer in humans. Today, the International Agency for Research on Cancer (IARC) recognizes alcohol as a Group 1 carcinogen3. This paper briefly describes the innate and adaptive immune system, how alcohol affects them, the different types of cancer associated with alcohol use, and the mechanisms of action responsible for the carcinogenic and tumorigenic effects of alcohol.
OVERVIEW OF THE IMMUNE SYSTEM
The IS is the group of organs, cells, and molecules that, acting in concert through organized processes that require cell-to-cell communication, recognize tissue damage, pathogens, and harmful stimuli in the body to orchestrate alerting and repairing responses to restore homeostasis after insults or aging4. The IS comprises specific tissues and organs where immune cells are generated, mature, and are activated, such as the bone marrow, thymus, lymph nodes, spleen, Peyer's patches, and Waldeyer's ring (Fig. 1). This system displays distinct reactions based on the type and time course of infections or tissue damage.
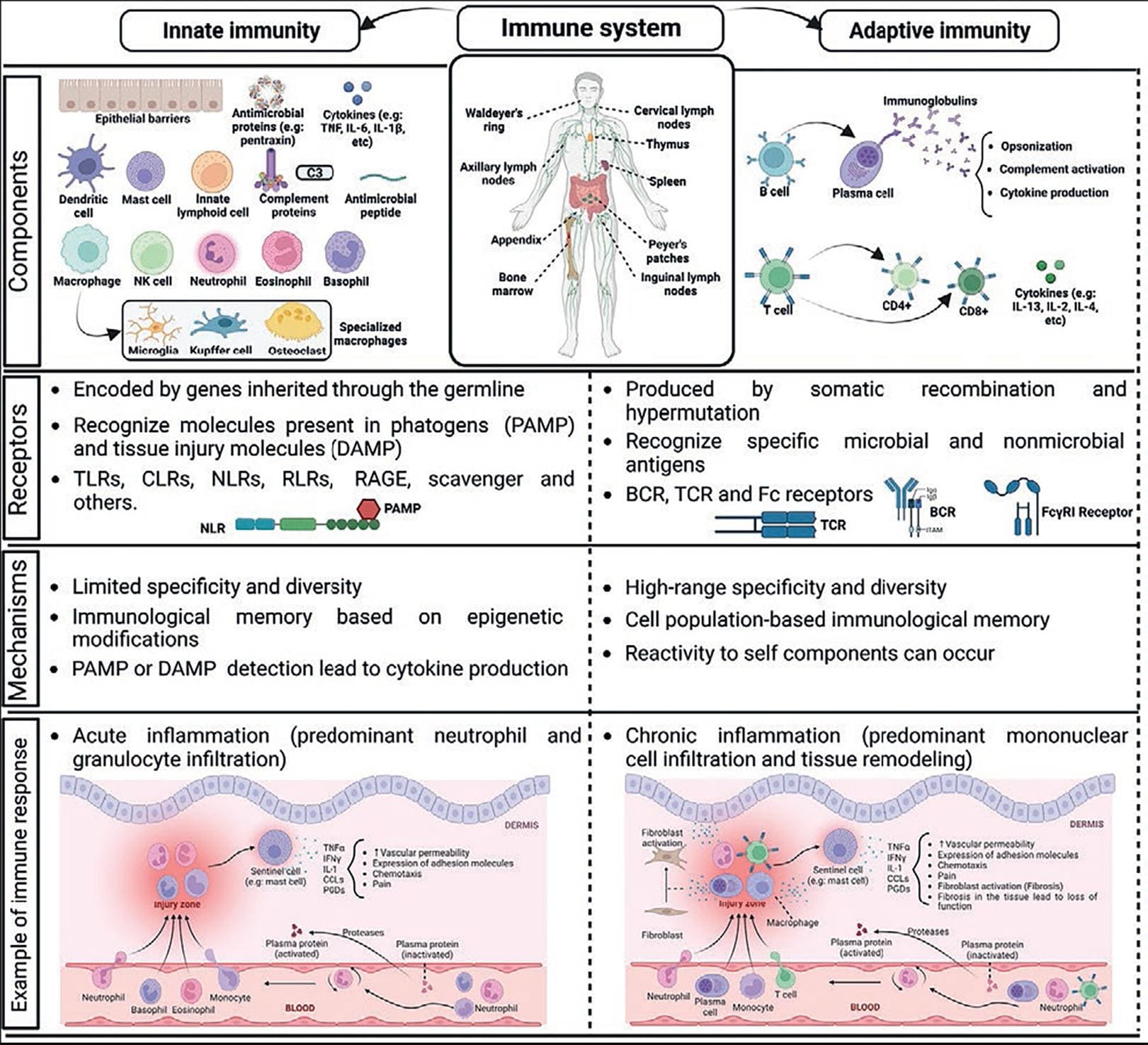
Figure 1. Overlook of innate and adaptive immune responses. Main elements of immune system and their most relevant responses are shown (see text for details).
The innate immune system
The innate IS, composed by the innate immunity responses, constitutes the first line of defense against germs or damage in the body. In general, it is nonspecific, acts rapidly, and has limited efficiency. It comprises physical barriers to prevent the entrance of external substances, specialized secretions, such as tears, mucus, and saliva. The innate IS involves the activation of tissue-resident sentinel cells, such as mast cells, macrophages, and natural killer (NK) cells, to later recruit neutrophils and distinct lymphocytes to the site of damage.
The inflammatory response is the most important innate immune process and usually precedes the activation of T cell-dependent and other adaptive responses. It is classically triggered by the activation of pattern-recognition receptors (PRRs) by pathogen-associated molecular patterns, such as bacterial lipopolysaccharide (LPS) or viral proteins, together with damage-associated molecular patterns (DAMPs), such as heat-shock proteins or intracellular proteins. Innate immunity is triggered by the activation of PRRs, such as the toll-like receptors (TLR), the RIG-1-like receptors, the C-lectin receptors, the receptor for advanced glycation end products (RAGE), and others, together with enzymatic cascades in plasma. PRRs' genes are codified in germline and normally variants of those molecules are not produced. Triggering of PRRs activates distinct signaling cascades, including the nuclear factor kappa light chain enhancer of activated B cells (NF-kB) that promotes the transcription of numerous genes coding for immune mediators (cytokines and chemokines).
Inflammation is a protective reaction that favors pathogen recognition and tissue repair, but it can also provoke tissue damage when it remains uncontrolled or becomes chronic.
The adaptive immune system
The adaptive IS orchestrates late and complex immunity responses, requiring the activation of T and B lymphocytes which initiate a differentiation process that includes proliferation, cytokine production, and (in the case of B cells) antibody production. T cell-mediated adaptive responses initiate after antigen presentation by dendritic cells to T-cell receptor (TCR), causing cytokine production, T cell proliferation, and T-cell differentiation in distinct effector subsets. Adaptive immune responses include B-cell-dependent antibody production, which starts after antigen recognition via the B-cell receptor (BCR). Once produced, antibodies participate in antigen neutralization (opsonization), activation of the complement cascade, and upon being recognized by Fc receptors, induce cytokine and pro-inflammatory cytokine production by immune cells. Alteration of the early and efficient innate immune response against pathogenic or mechanical insults contributes to an inadequate adaptive response that results in uncontrolled inflammation and host tissue damage. Ultimately, several mechanisms leading to tissue repair and removal of dead immune cells operate in the so-called resolution phase after generating an immune response.
The interrelationship between the innate and adaptive immune systems
Mediators with pro-inflammatory and regulatory functions orchestrate the effective connection between cells of the innate IS and the adaptive immune responses. These mediators include pre-formed compounds (such as histamine), arachidonic acid derivatives, reactive oxygen and nitrogen species (ROS and NOS), growth factors, and cytokines4 (Fig. 1).
Chemotactic cytokines, or chemokines, are a large family of secreted proteins that stimulate the recruitment of leukocytes to sites of infection or/and tissue damage. Pro-inflammatory cytokines alert the IS against invading pathogens and the nervous system (NS) of potential damage. This group of mediators includes several interleukins (small proteins secreted by leukocytes and other cells which modulate cell activation during inflammatory and immune responses), such as interleukin (IL)-1α, IL-2, IL-6, the tumor necrosis factor (TNF)-α (produced by monocytes and macrophages during acute inflammation), and chemokines such as IL-8, CCL2, and CCL5, among others. Anti-inflammatory cytokines limit the actions of pro-inflammatory ones and include IL-4, IL-10, the transforming growth factor (TGF)-β (which inhibits proliferation and induces apoptosis of several cells), and soluble cytokine receptors that capture active cytokines, limiting their actions (Table 1). On the other hand, anti-inflammatory cytokines act in concert with bioactive lipids, such as lipoxins and resolvins, endocannabinoids, and other pro-resolving lipids, to limit the damage produced by pathogens, tissue damage, or an excessive inflammatory response5.
Table 1. Major pro-inflammatory and anti-inflammatory mediators
Pro-inflammatory mediators | Anti-inflammatory mediators |
---|---|
IL-1a | IL-4 |
TNF-α | IL-10 |
IL-2 | TGF-β |
IL-6 | Type II IL-1 receptor |
IL-8 | Soluble TNF-a receptor |
CCL2 | |
CCL5 |
IL-1a: interleukin-1a; TNF-α: tumor necrosis factor-α; TGF-β: transforming growth factor-β.
ALCOHOL'S PLEIOTROPIC EFFECTS ON THE IMMUNE SYSTEM
Alcohol produces complex and multiple deleterious effects on innate and adaptive immune responses, which can be inferred from epidemiologic data. For example, alcohol use increases the incidence of infections, such as pneumonia, caused by antibiotic-resistant bacteria, HIV infection, and tuberculosis6. Furthermore, chronic alcohol consumption significantly increases the probability of developing acute respiratory distress syndrome7 and may also modify susceptibility and worsen COVID-19 infection8,9.
The literature about the pre-clinical and clinical effects of alcohol misuse on immune function is abundant. Research analyzing innate and adaptive immune responses in cultured cells, ex vivo tissues, and laboratory animals allows for well-controlled conditions and precise determinations. However, distinct protocols of alcohol exposure make it difficult to compare some results. On the other hand, studies in humans face significant complications due to the difficulty of patient enrollment and inconsistencies in the definition of "occasional drinkers," "heavy drinkers," or "frequent users." Nevertheless, despite differences in the amount and frequency of alcohol consumption in protocols used in pre-clinical models and humans, it is well accepted that alcohol misuse affects the function of innate and adaptive immune responses in a direct way and in a dose- and time-dependent fashion, altering metabolism and effector capacities of cell lineages or interfering with communication processes between cells, disturbing cytokine networking and causing defective reactions, inhibiting in the long-term protective effects, and promoting non-resolving inflammation10.
Effects of alcohol on innate immunity
Moderate alcohol consumption is associated with reduced inflammation and improved responses to vaccination in humans, while chronic heavy drinking is associated with decreased lymphocyte levels and increased risk of both bacterial and viral infections11,12.
The mechanisms behind dose and time-dependent alcohol actions are still under investigation since systematic studies testing distinct patterns of drinking and alcohol doses are difficult to perform. However, pre-clinical studies exposing rodents to a pro-inflammatory insult (e.g., LPS) after ethanol exposure have corroborated the dose-dependent effect of alcohol on the inflammation triggered by macrophages.
In vivo studies have shown that rodents that received a single ethanol administration responded with decreased IL-6 and IL-12 production in response to distinct TLR ligands13. In addition, pre-incubation of human monocytes isolated from healthy men and women with 25 mM alcohol (approximately 0.1 g/dL blood alcohol concentration, a level enough to produce a level of intoxication that impairs driving) for 24 h inhibits pro-inflammatory cytokine production14 and increases the proteolytic degradation of positive mediators of the transcription factor NFkB15. Furthermore, chronic exposure of human peripheral blood monocytes to 25 mM ethanol for 7 days increased LPS-induced TNF production without changing IL-10 levels (pro- and anti-inflammatory mediators, respectively)16. On the other hand, monocytes isolated from a person after 30 days of moderate beer consumption had better phagocytic, oxidative burst, and intracellular bactericidal activity when incubated with E. coli compared to control levels17. Furthermore, monocyte-derived dendritic cells obtained from human volunteers after consuming alcohol showed a reduced ability to induce T-cell proliferation in response to enterotoxin B and other antigens, suggesting impaired antigen presentation18.
Regarding studies performed in humans, a single episode of alcohol consumption in experienced human volunteers caused a rapid (20 min) increase in peripheral blood monocytes and LPS-induced TNF production when blood alcohol levels were close to 130 mg/dL. At later times (2 and 5 hours) post-binge, the number of those cells was reduced and an increase in IL-10 was observed7. Recently, Lee et al. determined the blood cytokine content of 25 heavy-drinking individuals after an oral alcohol administration19. Measurements were performed at baseline, 3 h after the binge, and when breath alcohol concentrations returned to zero. In that study, there was a significant reduction in TNFα and an increase in IL-6 without any change in IL-18 or IL-1019.
In general, evidence from in vivo and in vitro experiments shows that ethanol modulates the function of innate immune cells in a dose- and time-dependent manner. The results indicate that acute high alcohol doses inhibit pro-inflammatory cytokine production. In contrast, long-term alcohol exposure stimulates pro-inflammatory cytokine production, contributing to losing protective responses against pathogens and deleterious chronic inflammation. In addition, consumption of moderate amounts of alcohol enhances bacterial phagocytosis and reduces inflammatory cytokine production, whereas chronic high consumption inhibits cytokine synthesis and effector activities of immune cells. To date, the consequences of those impaired immune reactions in developing specific chronic diseases, such as cancer, are a matter of intensive research.
Effects of alcohol on adaptive immunity
Effects of alcohol consumption on adaptive immunity responses, such as an impairment in the generation of specific T-cell populations or diminished numbers of B cells, were described in humans in early studies20,21. Alterations in the number of peripheral T cells and disbalance among distinct T-cell populations, including increased T cell apoptosis, have been observed in almost every study performed with humans or laboratory animals22. Furthermore, peripheral B cells diminished after continuous alcohol consumption, and B-cell disbalance is reflected in increased amounts of IgM and IgA found in individuals considered heavy drinkers23. Deregulated antibody production seems to be a common element in alcohol users since lower levels of IgG were observed even in moderate alcohol consumers11,22,23.
Effects of alcohol on immune responses in the central nervous system
Effects of prolonged alcohol consumption on immune responses inside the human brain were first detected using DNA microarrays24. In particular, there was an increase in the expression of genes associated with innate immune cells in cortex samples of alcohol-dependent individuals. A short time later, experimental approaches showed alcohol-induced cytokine production in the brain25. Since those initial studies, changes in neuroimmune signaling in the CNS that occurs during alcohol ingestion and withdrawal have been an active research field. Alcohol use causes neuroinflammation by releasing several DAMPs, such as HMGB1 protein, S100 proteins, heat shock proteins, and peptides derived from the degradation of the extracellular matrix. Those molecules are ligands of TLRs that, after activation, lead to microglial pro-inflammatory cytokine production, neuronal death, and sustained chronic inflammation26. Activation of TLRs is involved in the neuroinflammatory effects of alcohol. For example, TLR4 knockout animals do not induce pro-inflammatory cytokine production after chronic ethanol consumption27. Evidence shows that postmortem brain tissue from alcohol-consuming patients has increased TLR receptor expression compared to healthy individuals28.
Data pointing to a crucial role of a particular PRR, the toll receptor 3 (TLR3), in the pathogenesis of alcoholism has risen since its expression is increased in postmortem samples of the orbitofrontal cortex of individuals suffering from that condition28 Similarly, TLR3 expression was increased in brain samples of rodents exposed to alcohol. Moreover, the administration of a TLR3 agonist gradually increased the amount of voluntary ethanol consumption29. In addition to the classical activation of innate immunity receptors by DAMPs produced by alcohol-damaged cells, recent studies indicate that extracellular vesicles (EVs) may play a role in the damage produced by alcohol consumption. EVs are small non-classical secretory vesicles allowing intercellular communication, delivering their cargo (proteins, metabolites, or nucleic acids) to recipient cells. For example, EVs derived from microglia mediate the increase in TNFα and IL-1β production associated with ethanol intake. Moreover, the blockage of EV secretion inhibited the ethanol-induced pro-inflammatory activation of microglia30,31.
Special interest has been recently placed on the study of the effects of alcohol on elderly persons because binge alcohol consumption is frequent among older individuals32. Although there are no available animal models to analyze the effects of alcohol in older individuals, it has been proposed that they could be more deleterious due to an increase in microglial activation in the aging brain33. In addition, the effects of alcohol on immune functions increase with age due to distinct factors, such as immune cell senescence and gut barrier dysfunction10,34.
ALCOHOL METABOLISM
Alcohol is a small water-soluble molecule (CH3-CH2-OH), and, as such, it is rapidly absorbed in the gastrointestinal tract. Alcohol distribution in the body is also rapid, with tissue levels approximating the blood concentration. In general, the volume of distribution of alcohol is lower in women than in men due to their higher percentage of body fat, so after drinking the same amount of alcohol, a woman would have a higher maximum blood alcohol level than a man with the same body weight35.
After ingestion, alcohol reaches maximum blood concentrations in approximately 30 min in fasting individuals. The presence of food in the stomach delays alcohol absorption because it delays gastric emptying. Once alcohol reaches the intestine, it rapidly enters the bloodstream. The kidneys and lungs eliminate only 2% to 10% of alcohol; the rest is oxidized in the body, mainly in the liver. The extrahepatic metabolism of ethanol is negligible, except in the stomach. A minor fraction of the absorbed alcohol undergoes non-oxidative metabolism resulting mainly in phosphatidylethanol and fatty acid ethyl esters36.
The oxidative metabolism of alcohol involves three enzymes, alcohol dehydrogenase (ADH) in the cytosol, cytochrome P450 2E1 (CYP2E1), and catalase. The enzyme ADH transforms ethanol into acetaldehyde, and aldehyde dehydrogenase (ALDH) converts acetaldehyde into acetate37 (Fig. 2).
Alcohol dehydrogenases
Alcohol dehydrogenase (ADH) belongs to a five-class superfamily of enzymes found mainly in hepatocytes, and in smaller amounts in the stomach and other tissues. ADH is responsible for approximately 80% of alcohol metabolism in the body. Members of the ADH family have been classified into five distinct classes, from ADH1 to ADH5. The literature on this topic can be confusing because it has changed along time (Table 2)37,38,39. In alcohol oxidation, it utilizes the coenzyme NAD+, thus increasing the NADH/NAD+ ratio. The class I ADH forms are mainly responsible for the oxidation of alcohol. Some of the orally ingested alcohol does not enter the systemic circulation (first-pass metabolism), as it is oxidized primarily in the stomach by ADH classes I, III, and IV isoforms (mainly σADH). Women have lower gastric levels of ADH than men, which explain why they have increased alcohol bioavailability. Genetic variations of this enzyme family (e.g., the ADH1B*2 allele) favor the rapid conversion of alcohol to acetaldehyde, which is a highly toxic, mutagenic, and carcinogenic substance37,40 (see below). Limited data in Native American and Mexican-American populations suggest that the ADH1B*2 allele is rare in these groups41.
Table 2. The five classes of alcohol dehydrogenase isoenzymes in humansa
Class | Gene Nomenclature | Subunit type | Tissue distribution | Ethnicity | Characteristics | Comments |
---|---|---|---|---|---|---|
I | ADH1A (Former ADH1) | α | Liver | |||
ADH1B (Former ADH2) | β | Liver, lung | ||||
ADH1B*1 (Former ADH2*1) | β1 | Caucasian and Black populations | Normal enzyme activity | Reference allele (ADH wild type) | ||
ADH1B*2 (Former ADH2*2) | β2 | Asians (Chinese, Japanese and Koreans). 25% of people with Jewish ancestry | High enzyme activity | It has an Arg48His substitution (↑↑↑ turnover rates that encoded by AD1B*1). High activity even at low alcohol concentrations. It produces large amounts of acetaldehyde | ||
ADH1B*3 (Former ADH1B*3) | β3 | African descendants | High enzyme activity (catalytic activity when the alcohol reaches high concentrations) | It has an Arg370Cys substitution (↑↑↑ turnover rates that encoded by AD1B*1). Rapid conversion of alcohol to acetaldehyde at high alcohol concentrations | ||
ADH1C | γ | Liver, stomach | ||||
ADH1C*1 (Former ADH3*1) | γ1 | All groups | ||||
ADH1C*2 (Former ADH3*1) | γ2 | Caucasian population | ||||
II | ADH4 | π | Liver, cornea | Low affinity for alcohol | It does not play a role in the metabolism of exogenous alcohol. Responsible for the oxidation of long chain alcohols. | |
III | ADH5 | χ | Most tissues | χ | Low affinity for alcohol | Does not participate in the oxidation of alcohol in the liver. Participates in first-pass metabolism |
IV | ADH7 | σ, μ | Stomach, esophagus | Responsible for alcohol first-pass metabolism (mainly σADH) | Involved in first-pass metabolism. It is found in significantly higher concentrations in men than in women | |
V | ADH6 | – | Liver, stomach | – |
aBased on references (37-39). The five classes of alcohol dehydrogenase isoenzymes in humans (ADH1-5). Class 1 ADH isoenzymes are the most relevant for alcohol oxidation (approximately 70% of alcohol metabolism). ADH class 2 isoenzymes have an intermediate affinity for alcohol, and ADH class 3 isoenzymes have a low affinity for alcohol and do not oxidize alcohol in the liver. Class 4 ADH is present in the human stomach, and the isoenzyme σADH, together with classes I and III ADH, is involved in the first-pass metabolism of alcohol. Due to the extremely labile activity of ADH class 5, several of its characteristics have not been established and it does not contribute significantly to alcohol oxidation.
Cytochrome P450 E1 (CYP2E1)
CYP2E1 belongs to the cytochrome P450 superfamily of drug-metabolizing enzymes. It is highly expressed in liver mitochondria and usually plays a minor role in alcohol metabolism. However, CYP2E1 is an inducible enzyme that becomes particularly important in the metabolism of high alcohol concentrations or alcohol-dependent patients. Since CYP2E1 uses NADP+ as a coenzyme, alcohol metabolism elevates the NADPH/NADP+ ratio and limits the availability of NADPH for the regeneration of reduced glutathione. This results in an effect in oxidative stress42.
Catalase
Catalase enzyme is located in cell bodies called peroxisomes, which play a significant role in ROS detoxification. This enzyme can oxidize ethanol in the presence of hydrogen peroxide (H2O2) generating systems, such as the enzyme complex NADPH oxidase or the enzyme xanthine oxidase. Therefore, this is considered a minor route of alcohol oxidation37.
Acetaldehyde
Regardless of the enzyme involved, acetaldehyde is the resulting active metabolite of alcohol oxidation. Acetaldehyde is an organic chemical compound (CH3-CHO) that induces several toxic, pharmacological, and behavioral responses. Although acetaldehyde is short lived because it is rapidly broken down to acetate by intermediation of ALDH, it can cause noticeable damage to cells and tissues. Acetaldehyde has three main modes of action concerning carcinogenesis: (1) By forming carcinogenic DNA adducts, (2) by inhibiting DNA repair, and (3) because it has significant effects on epigenetics (DNA methylation)43.
Aldehyde dehydrogenase
The aldehyde dehydrogenase (ALDH) group of enzymes converts acetaldehyde to acetate (Table 3)37,39,44. Two genes, ALDH1 and ALDH2, encode enzymes significantly associated with acetaldehyde oxidation in the liver using NAD+ as the cofactor, which is reduced to NADH. The ALDH reaction is irreversible, and the circulating levels of acetaldehyde are low under normal conditions. From the liver, the acetate passes to peripheral tissues, where it is metabolized to acetyl-CoA; it can then be included in the tricarboxylic acid cycle or fatty acid synthesis.
Table 3. The three classes of aldehyde dehydrogenase isoenzymes in humansa
Class | Gene Locus | Genetic Polymorphism | Tissue distribution | Activity | Comments |
---|---|---|---|---|---|
I | ALDH1 | Mainly in liver; other tissues | Responsible for the oxidation of intracellular aldehydes (cytosolic). | ||
II | ALDH2 | Liver and other tissues | |||
ALDH2*1 (Glu487) | Normal catalytic activity | Reduce the accumulation of acetaldehyde. | |||
ALDH2*2 (Lys487) | Inactive | ↑ Blood acetaldehyde concentrations after intake moderate amount of alcohol. | |||
ALDH2*2 homozygotes present severe manifestations associated with acetaldehyde accumulation (nausea, vomiting and facial flushing) due to which they do not tolerate alcohol intake. | |||||
Heterozygous individuals (ALDH2*1/2*2) may tolerate alcohol despite the flushing reaction, (acetaldehyde levels may triple with respect to normal metabolizers). | |||||
ALDH2*2 heterozygotes or homozygotes have ↑ risk of cancer (e.g., upper digestive tract and liver) among the drinking and smoking populations. | |||||
III | ALDH3 | Liver, stomach, cornea | Low or non-detectable level in the liver and other normal human tissues. |
aBased on references (37,39,44). Aldehyde dehydrogenases (ALDH) belong to a superfamily of isozymes and play important roles mainly in the detoxification of endogenous and exogenous aldehydes. Mitochondrial ALDH (ALDH2) plays a key role in the detoxification of acetaldehyde from alcohol metabolism. There are two variants of ALDH2: (a) ALDH2*1 with normal catalytic activity, and (b) ALDH2*2 which lacks activity.
A common polymorphism of this enzyme is the ALDH2*2 variant allele, which has significantly decreased activity. This variant is prevalent in various Asian ethnic groups (mainly East Asians). When people carrying this allele drink alcohol, they experience the unpleasant effects of acetaldehyde accumulation, including facial flushing, hypotension, tachycardia, and nausea45. It has been estimated that the presence of the ALDH2*2 allele is uncommon among Native-American and Mexican-American populations41.
ALCOHOL AND CANCER
According to the World Health Organization (WHO), alcohol use accounted for 4% of cancer diagnoses worldwide in 202046. In 1988, the International Agency for Research on Cancer (IARC) declared alcohol a carcinogen47. Scientific evidence in several subsequent studies has confirmed this assertion. Cancer types related to alcohol use include cancers of the head and neck, esophagus, liver, breast, colon, and rectum. The amount of alcohol consumed over a lifetime is related to the relative risk of being diagnosed with alcohol-associated cancer. According to recently published data, although heavy alcohol consumption is associated with the highest percentage of cancer, moderate consumption is also associated with that risk48 (Table 4). Thus, there is increasing evidence that daily alcohol consumption, even at lower levels, increases the risk of developing cancer later in life, which has led to reevaluating the idea of a safe consumption threshold. Another consideration is the possible impact of combined alcohol and tobacco use on the increased risk of various alcohol-associated cancers. According to the results of several studies, excessive consumption of alcohol and tobacco has a multiplicative increase in the occurrence of cancer49.
Table 4. Global burden of cancer in 2020 attributable to alcohol consumption
Alcohol consumption | Estimated percentage |
---|---|
Heavy drinking (> 60 g/day) | 46.7% |
Risky drinking (20-60 g/day) | 39.4% |
Moderate drinking (> 20 g/day) | 13.9% |
An estimated 741,300 of all new cases of cancer were attributable to alcohol consumption during 202044.
MAIN TYPES OF CANCER ASSOCIATED WITH ALCOHOL CONSUMPTION
Head-and-neck cancer
Alcohol use is a significant risk factor for some head-and-neck cancers, particularly those affecting the oral cavity, pharynx, and larynx. People who consume 50 g of alcohol or more daily (approximately 3.5 drinks or more per day) have at least a two to three times greater risk of developing these cancers than nondrinkers. Notably, the risk of developing these types of neoplasms is substantially higher in people who also use tobacco50.
Esophageal cancer
Any level of alcohol consumption increases the risk of esophageal cancer, mainly squamous cell carcinoma; however, the higher the alcohol intake, the greater the likelihood of developing esophageal cancer. Compared to non-consumption, excessive alcohol use increases the risk of developing esophageal cancer by up to five times. In addition, the variant allele of the aldehyde dehydrogenase enzyme ALDH2*2 confers a nearly four-fold increased risk of esophageal cancer among drinkers compared to ALDH2*1 carriers51.
Colorectal cancer
Moderate to heavy alcohol intake is associated with a 1.2-1.5 times higher risk of colon and rectal cancer than no alcohol consumption. The mechanisms of action for the effect of chronic alcohol consumption on the development of colorectal cancer appear to be diverse and are not well elucidated. There is evidence that acetaldehyde may be carcinogenic in colonocytes. Alcohol may also act as a solvent for cellular penetration of dietary or environmental carcinogens, affect hormone metabolism, or interfere with retinoid metabolism and DNA repair mechanisms. More recent research has focused on the impact of chronic high alcohol intake on gut microbiome dysbiosis and the weakening of intestinal barrier function52,53.
Liver cancer
Chronic hepatitis B virus and hepatitis C virus infection are other major causes of liver cancer occurrence. Individuals with alcohol-related diseases have a higher risk of liver cancer than the general population, and alcohol consumption is positively associated with liver cancer risk. Its estimated risk of occurrence is 1.3 times in chronic consumers of 2 drinks/day of alcohol. There are several possible mechanisms by which alcohol can induce hepatocarcinogenesis, including changes in the immune response, mutagenic effects of acetaldehyde, and ROS production due to excessive hepatic iron deposition. In addition, alcohol accelerates hepatic carcinogenesis through several signaling pathways, including the gut-liver axis54,55.
Breast cancer
Several studies have invariably found an increased risk of breast cancer associated with alcohol consumption56. For example, a meta-analysis of more than 50 studies involving 58,000 women with breast cancer found that women who drank more than 45 g of alcohol per day (equivalent to approximately 3.5 drinks or more/day) had 1.5 times the risk of breast cancer compared to those who did not. The risk of developing breast cancer was even higher in women who consumed less alcohol. For every 10 g of alcohol consumed per day, the researchers observed a 7% increase in the risk of developing breast cancer; other studies have found that this increase can be as high as 12%57.
The mechanisms by which alcohol may increase the risk of breast cancer remain uncertain and appear diverse. The hepatic metabolism of alcohol may influence its ability to metabolize various host hormones. Alcohol may increase circulating levels of estrogen, which is an established risk factor for breast cancer. Alcohol can also be metabolized in breast tissue and form acetaldehyde leading to ROS causing potential DNA damage. There is evidence that breast tissue is more susceptible to the deleterious effects of acetaldehyde than other organs58.
In vitro studies with human tumor cells have identified signaling molecules that may contribute to the effects of alcohol in favoring breast cancer. They include ROS, acetaldehyde, matrix metalloproteases, ErbB2/Her2/Neu receptor tyrosine kinase, as well as E-cadherins, cytosolic protein kinases, adenylyl cyclase, estrogen receptors, and different transcription factors59.
Other types of cancer
Numerous studies have evaluated whether there is an association between alcohol consumption and the risk of developing other types of cancer. For stomach, ovarian, and uterine cancers, no association with alcohol consumption has been found, or the evidence for an association is still inconsistent. There appears to be no relationship between alcohol consumption and adenocarcinoma of the esophagus or with endometrial, urinary bladder, and kidney cancer. In contrast, there is evidence that alcohol use increases the risk of melanoma and prostate and pancreatic cancer60.
MECHANISMS OF ALCOHOL-INDUCED CARCINOGENESIS
Although the precise causes of ethanol-associated carcinogenesis are still unknown, there are several biological mechanisms that may explain the relationship between alcohol and cancer development (Fig. 3).
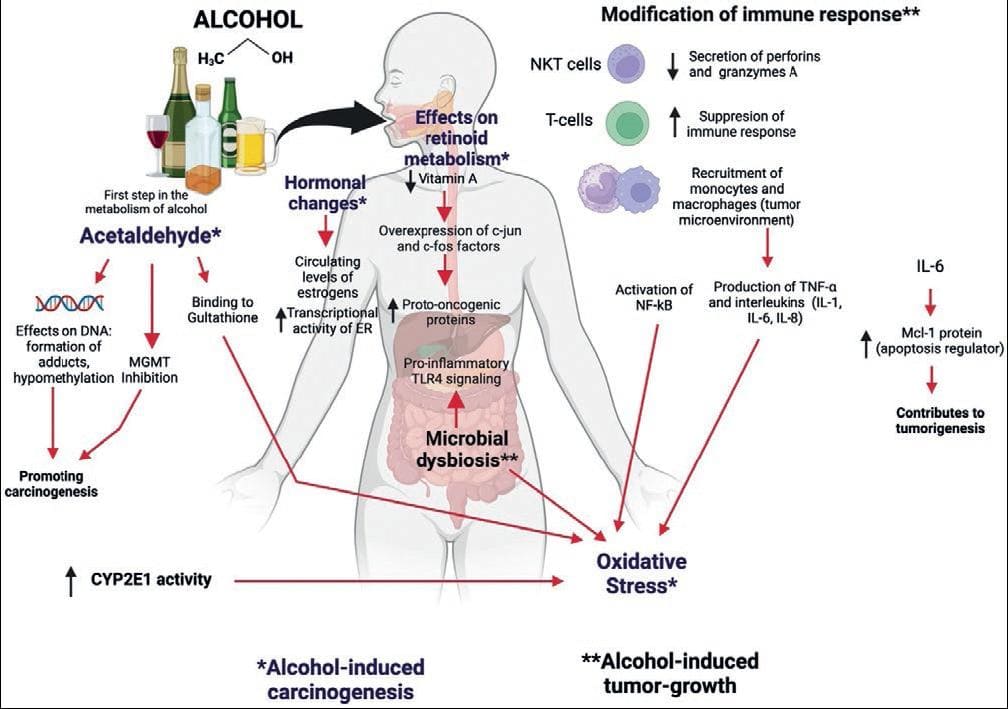
Figure 3. Main mechanisms proposed for alcohol-induced carcinogenesis and tumor growth (see text for details).
Acetaldehyde (AA)-induced DNA adducts
The IARC (International Agency for Research of Cancer) identifies acetaldehyde as a carcinogen47. Some evidence is that acetaldehyde can bind to DNA and form DNA adducts, i.e., pieces of DNA covalently bond to a chemical that modifies its physical shape, potentially blocking its synthesis and repair61. These DNA adducts can produce DNA point mutations, double-strand breaks, sister chromatid exchanges, and structural chromosome changes. In addition, some adducts can form additional highly genotoxic structures, such as DNA interstrand bonds and DNA-protein crosslinks that can promote carcinogenesis. Acetaldehyde can also bind directly to proteins such as glutathione, a protein involved in reducing oxidative stress. As for the DNA repair process, acetaldehyde directly inhibits a critical enzyme in the repair of DNA adducts caused by alkylating agents (O6-methylguanine-DNA methyltransferase, known as MGMT).
Alcohol and acetaldehyde can change the expression of oncogenes and tumor suppressor genes by affecting DNA methylation62. In particular, acetaldehyde can inhibit the activity of DNA methyltransferase (DNMT), which is essential for normal DNA methylation62. People who drink alcohol and carry the variant ALDH2*2 allele (the variant with low activity to convert acetaldehyde to acetate; see Table 3) have a substantially increased risk of esophageal and upper aerodigestive tract cancer63.
Acetaldehyde contributes to the destruction of folate, which, associated with a low intake, can alter DNA synthesis by reducing the availability of nucleotides. The effect of alcohol on carbohydrate and folate metabolism may be an important factor in the development of colorectal cancer64.
Oxidative stress
Alcohol can contribute to carcinogenesis by inducing oxidative stress by activating specific pathways that produce ROS, such as superoxide anion and hydrogen peroxide65. One of the main pathways of ROS generation is a result of increased CYP2E1 activity during the process of ethanol oxidation to acetaldehyde66. High alcohol consumption is known to induce CYP2E1 expression, which increases the activity of this enzyme. In addition, ROS favors lipid peroxidation with the generation of aldehydes that, upon binding to DNA, form highly mutagenic adducts that cause changes in several genes involved in cell cycle regulation and tumor suppression. Several researchers have demonstrated the correlation between increased CYP2E1 activity and the formation of etheno-DNA adducts in pre-clinical models and human tissues related to ethanol-mediated carcinogenesis in the esophagus, liver, and colorectum66.
Other ROS targets in tumor progression and metastasis include Src kinase, RhoA GTPase, and the nuclear transcription factors NF-kB and STAT-3. In addition, activation of NF-κB promotes cell proliferation and metastasis by interfering with mitogen-activated protein kinase signaling pathways and upregulating vascular endothelial growth factor (VEGF) and chemokine CCL2 (also known as monocyte chemotactic protein-1, MCP-1), which stimulates angiogenesis, a process necessary for tumor growth67.
Alterations in DNA methylation
DNA methylation plays a crucial role in controlling gene activity: Hypermethylation has a gene-silencing effect, and hypomethylation increases gene expression. Methylation is carried out by three enzymes (DNA methyltransferases or DNMTs) that transfer the methyl group from SAMe (S-Adenosyl-Methionine) to DNA.
Alterations in DNA methylation are present in many diseases, including cancer. Alcohol and acetaldehyde can reduce the activity of enzymes involved in this process62 and decreasing folate concentrations68.
Modifications in retinoid metabolism
Chronic alcohol consumption decreases Vitamin A absorption and affects retinol metabolism leading to overexpression of factors such as c-Jun and c-Fos that favor the increase of proto-oncogenic proteins, mainly in hepatocytes. In addition, alcohol increases the activity of CYP2E1, which also metabolizes retinoic acid, producing various toxic metabolites69.
MECHANISMS OF ALCOHOL-INDUCED TUMOR GROWTH
Alcohol consumption has also been related to tumor growth, mainly due to modifications it produces in the IS and intestinal microbiota (Fig. 2).
Modification of the immune system
As mentioned in previous sections, alcohol has several deleterious effects on the host's IS. For example, it can alter perforins and granzymes A and B, necessary for NK cells to act on potentially neoplastic cells70. Alcohol can also affect NKT cells, which play a critical role in cancer cell death, and suppres T-cell immune responses, resulting in impaired anti-tumor regulation.
Chronic alcohol consumption favors the recruitment of monocytes and macrophages in the tumor micro-environment, generating TNFα and various interleukins (IL-1, IL-6, and IL-8) that increase oxidative stress. Significantly, IL-6 stimulates the production of Mcl-1 (anti-apoptotic protein), which reduces cell death.
Changes in the immune system caused by alcohol can accentuate the damage caused by viral infections such as hepatitis C71.
Changes in the intestinal microbiota
High alcohol consumption can induce microbial dysbiosis and promote bacterial overgrowth in the gastrointestinal tract72. These changes produce greater susceptibility to pathogenic organisms due to the compromise of the intestinal barrier, inflammatory changes, and the generation of various carcinogenic substances. Changes in permeability favor bacterial products, such as lipopolysaccharides and peptidoglycans, from entering the intestine into the hepatic circulation. Once in the liver, a chronic inflammatory state involving hepatocytes, endothelial cells, Kupffer cells, and stellate cells is promoted, and pro-inflammatory TLR4 signaling pathways associated with hepatocarcinogenesis are induced73.
CONCLUSIONS
Alcohol consumption exerts various deleterious effects on the immune system, limiting effective responses against bacteria, viruses, and other infectious agents. Alcohol-induced modifications of the innate immune system are dose- and time-dependent. The main effects are defective pathogen recognition, aberrant cytokine synthesis, and induction of chronic inflammation. Regarding adaptive immunity, alcohol alters T-cell populations and B-cell function, resulting in defective antibody production.
Alcohol consumption is an established risk factor for several malignant neoplasms, and alcohol itself is considered a Group 1 carcinogen by the International Agency for Research on Cancer; this group also includes agents such as tobacco, radiation, and asbestos. Alcohol causes cancer through various biological mechanisms derived from its metabolism, so any beverage containing alcohol poses a risk for the drinker of developing cancer. In general, a higher alcohol consumption is related to a significant risk of cancer; however, recent statistics have found that even moderate drinkers have an increased occurrence of the disease compared to teetotalers. Therefore, it is essential to raise awareness among health professionals and patients and consider it alcohol use a modifiable risk factor, especially in at-risk populations.