INTRODUCTION
Bacteria, archaea, viruses, phages, fungi, protists, and nematodes among other microorganisms colonize the digestive tract to form the human gut microbiome, which has been highlighted for its profound role in human health. The gut microbiome has approximately 100 times the genes of the human genome1. Although evidence is inconclusive, most frequently a healthy gut microbiota is characterized by higher microbial diversity and richness, which can be important for metabolic function1,2. Beginning at birth, an individual’s signature microbiota composition is influenced by a variety of factors, including mode of birth and genetics, and continues to change over time with the influence of diet, environment, and lifestyle1. To this point, alterations in the gut microbiota, known as dysbiosis, are increasingly evident in the development and severity of metabolic disorders such as obesity and type 2 diabetes (T2D)3. In addition, seminal studies highlight the transferability of an obese phenotype to germ-free mouse models through transfer of human gut microbiota3. Despite evidence on the role of the gut microbiome in metabolic disorders and the transfer of obese phenotypes, the exact connection between gut microbiota and metabolic phenotypic outcomes in humans is largely unknown. The microbiota-gut-brain axis theory suggests gut microbiota and bacteria-derived metabolites influence metabolic health through modulation of the gut-brain axis4.
The microbiota-gut-brain axis refers to bidirectional signaling between the gastrointestinal tract and the brain. It is thought to be heavily influenced by the gut microbiota and involved in a multitude of homeostatic biological processes, including digestive function, hunger and satiety, and eating behavior. Gut microbiota and bacteria-derived metabolites interact with the gut-brain axis efferent and afferent pathways, while also exhibiting a high degree of interconnection and crosstalk (Fig. 1)5. The three predominant signaling pathways for gut-brain axis communication are neuronal, endocrine, and immune.
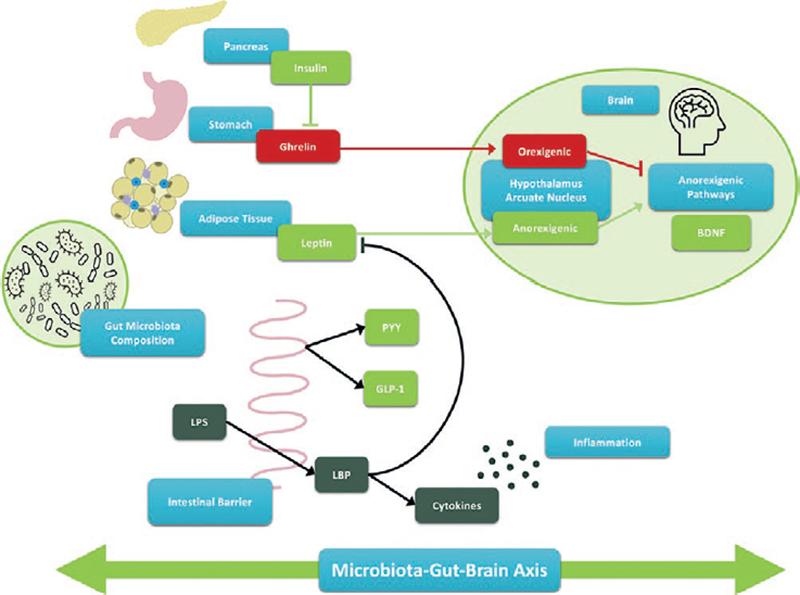
Figure 1. The microbiota-gut-brain axis. The microbiota-gut-brain axis is a bidirectional relationship between the gut microbiota, digestive system, and the brain. The neuronal, endocrine, and immune pathways are three intertwined components of the microbiota-gut-brain axis and are mediated by gut bacteria and bacteria-derived metabolites, impacting hunger and satiety, inflammation, and eating behavior.
Neuronal signaling pathway
Nutrients in the gastrointestinal tract transmit information to the hypothalamus through the vagus nerve of the autonomous nervous system. In response, the hypothalamus serves an essential role in the host’s homeostatic control, including regulation of appetite and energy balance, by sending feedback to the gut3.
Endocrine signaling pathway
Non-digestible carbohydrate fermentation by the gut microbiota produces short-chain fatty acids (SCFAs) which stimulate the secretion of anorexigenic factors such as glucagon-like peptide 1 (GLP-1) and peptide YY (PYY). Secretion occurs as a result of food ingestion but can continue long after meals are finished to prolong the feeling of satiety. To that end, individuals with obesity typically display decreased levels of GLP-1 and PYY, suggesting their essential role in the regulation of body weight, food intake, and metabolism6. In addition to the anorexigenic hormones secreted following SCFA production, other microbial products and metabolites influence the production of ghrelin, leptin, and serotonin through enteroendocrine cells3,5. Collectively, the gut microbiota and derived metabolites, like SCFAs, are key regulators in the production and secretion of hunger and satiety hormones.
Immune signaling pathway
The gastrointestinal tract is home to the densest population of immune cells4. Thus, the gut microbiota interacts with the innate and adaptive immune systems, both of which contribute to the maintenance of the intestinal barrier, as well as acute and chronic inflammatory processes. Markers of high intestinal permeability have been found in obesity and T2D, suggesting endotoxemia (or elevation of lipopolysaccharide in circulation stemming from increased gut permeability and leading to an activated inflammatory response) may be a mechanistic link between the microbiome, low-grade chronic inflammation, and development of cardiometabolic diseases3,7. As with the endocrine pathway of the gut-brain axis, SCFAs are also key players in the immune pathway, by enhancing the integrity of the intestinal barrier through the stimulation of mucus production and tight junction assembly. Evidence on the concentrations of SCFAs in metabolic disease outcomes has been inconsistent; however, most studies have suggested that SCFAs hold anti-inflammatory properties that can influence immune cells and reduce pro-inflammatory signaling by cells of the immune system4.
This review will examine evidence thus far about the potential practical applications of the microbiota-gut-brain axis in obesity prevention and treatment, highlighting opportunities for future research.
Practical and clinical considerations
Ever-increasing obesity rates pose a threat to public health, emphasizing a need for the continued exploration of sustainable and cost-effective interventions targeting obesity (Fig. 2). Obesity treatments incorporate a blend of surgical and non-surgical strategies including bariatric surgery, behavioral interventions, dietary changes, physical activity (PA), and pharmacotherapies8. Despite the variety of strategies for obesity treatment and prevention, each treatment incurs challenges and presents unique barriers to success. For example, bariatric surgeries are life altering, burdensome, and costly. Changes to behavior, diet, and PA can be unsustainable, difficult to implement, and/or ineffective particularly in weight loss maintenance8. Despite challenges in feasibility, most weight management strategies have been shown to influence the gut microbiota and promote healthy alterations to the gut-brain axis signaling factors9,10. Thus, an opportunity exists to maximize the potential benefit of current therapies or develop novel therapies that harness the microbiota-gut-brain axis to address obesity.
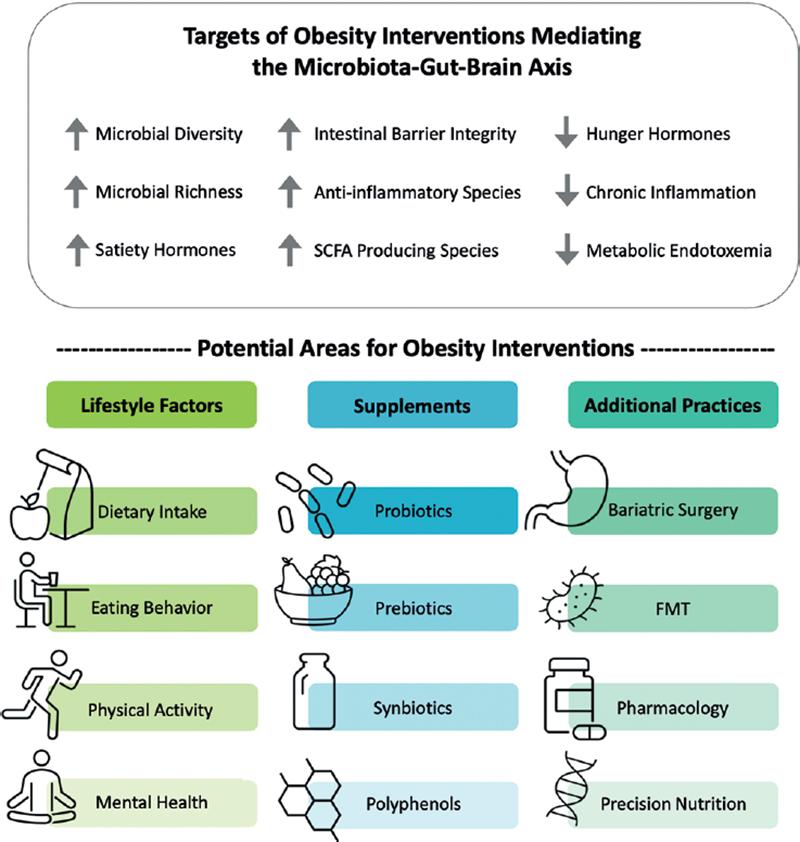
Figure 2. Impact of obesity interventions on the microbiota-gut-brain axis. Upper panel: Changes in the microbiota-gut brain axis that may result from obesity interventions. Lower Panel: Obesity interventions that are being explored as mediating the microbiota-gut-brain axis. Further research is needed to identify successful interventions in these key areas for future clinical applications to address obesity and metabolic diseases.
Lifestyle factors
Physical Activity
Exercise prescription is a common, evidence-based treatment for both psychological and gastrointestinal disorders10. Specifically, moderate to high-intensity mixed aerobic and resistance training has been seen to reduce inflammation significantly in individuals with T2D and obesity, as well as to promote microbial diversity, though the reason for this is unclear11,12. In addition to improving gut health, regular exercise has also been found to ameliorate and promote cognitive function, improve outcomes in psychopathology, and reduce the risk of brain atrophy in older adults13.
By contrast, overly vigorous or strenuous exercise has been found to negatively impact health, such that prolonged high-intensity endurance activities were associated with increased propensity for endotoxemia11. Further, the disruptive impact of exercise-induced stress, though intentional (e.g., high-intensity training such as long-distance running), is indistinguishable in its negative impacts on gut health from stress in response to dangerous stimuli (e.g., running to avoid harm)13. The biological response to such stress activates the hypothalamic-pituitary-adrenal axis (HPA). Interestingly, hyperresponsiveness of the HPA can be mediated by the gut microbiota and bacteria-derived metabolites, given that the HPA is a main communication pathway along the microbiota-gut-brain axis13.
Thus, moderate exercise prescription serves as an effective intervention to promote the improvement of microbial diversity and cognitive function as well as to reduce inflammation in adults with T2D and obesity11-13. Although human clinical evidence lacks a complete understanding about the relationship between exercise and the microbiota-gut-brain axis in treating or preventing obesity, the HPA appears intertwined in this approach. Furthermore, additional research is required to identify safe and appropriate clinical applications of exercise and the points at which exercise intensity, duration, and frequency may become detrimental to the microbiota-gut-brain axis.
Stress
Beginning in utero, the impacts of stress on gut microbial diversity can be seen throughout the lifetime. Prenatal stress is associated with long-term modulations to microbial richness and chronic exposure to psychosocial stress relates to altered microbial profiles in adults14. Concurrently, early-life stress has been seen to increase the risk of obesity in adulthood and longitudinal analyses have found exposure to life stressors (e.g., financial stress) to correlate with higher BMI and waist circumference14. However, there is no evidence to date demonstrating a causal relationship between stress-induced microbial changes and weight gain nor obesity15.
Stress is further associated with disruptions in gut-brain function, such that stress elevates ghrelin levels, which influence hypothalamic satiety and stress centers through the neuronal and endocrine pathways. Despite this physiological response, pharmacological interventions targeting ghrelin are not recommended because the amount by which a medication may lower ghrelin levels is imprecise and may result in abnormally low levels15.
Chronic stress can additionally increase gut permeability, allowing the microbiota-gut-brain axis’ neuronal and endocrine pathways to influence hypothalamic satiety and stress centers, which may contribute to dysregulated appetite and uncontrolled eating behavior associated with obesity14,16. Specifically, eating behaviors associated with overweight, such as emotional eating, credited increased levels of ghrelin, and other peptides following acute periods of stress17. Given the role of ghrelin in appetite and metabolic regulation, concentrations of ghrelin may impact the gut microbiome to increase the risk of obesity. Thus, interventions targeting stress tolerance and emotion regulation may be useful in reducing stress-induced overeating and microbiome dysbiosis, though further investigation is required.
Dietary patterns
Diet is a critical determinant of gut microbiota composition and function4. Changes in diet have consistently been found to alter gut microbial composition in as little as 24 h4. Dietary patterns associated with core compositional traits of the gut microbiome provide substrates for the production of a wide array of bacteria-derived metabolites in the microbiota-gut-brain axis18,19. It is important to note that gut microbiota exhibits a high level of resiliency, such that short term diet modifications may result in some microbiota compositional changes, but post-diet microbiota composition often remains stable with regard to dominant taxa and falls within the same pre-diet enterotype classification20. This evidence may have important implications when developing dietary interventions for populations with obesity who may require a more personalized approach for best results.
Western-style diets, generally high in salt, sugar, and/or saturated and trans fats, exhibit a similar gut microbiota profile as individuals with obesity and are often characterized by dysbiosis and negative metabolic health outcomes21. Notably, Hildebrandt et al. found a high-fat diet (HFD) increased Proteobacteria and Firmicutes, accompanied by decreased Bacteroides in mice22. High animal-derived saturated fat was also associated to an increase in B. wadsworthia, which induces systemic inflammation4. Given the detrimental impacts of various diets, such as the Western diet and HFD, on gut composition and metabolic health, dietary intake serves as a salient prospective target for obesity treatment and prevention.
Many dietary recommendations may positively impact the microbiota-gut-brain axis and overall health. The Mediterranean diet emphasizes fruits, vegetables, legumes, nuts, whole grains, and healthy fats, and is associated with reductions in cardiovascular disease4. Among other characteristics, the Mediterranean diet induces changes on the composition and inflammatory potential of the gut microbiota. A randomized feeding trial in adults with overweight and obesity found whole grain (WG) and fruit/vegetable (FV) intervention diets to decrease levels of inflammatory markers such as lipopolysaccharide-binding protein. WG diets led to decreased tumor necrosis factor alpha (TNF-alpha) while FV diets related to both decreased IL-6 and increased alpha diversity. Thus, a diet rich in WGs, fruits, and vegetables may be protective against obesity through its various anti-inflammatory roles23. In addition to dietary quality, dietary diversity has been associated with fecal microbiota stability24.
Similarly to the effects of increasing WG and FV intake, diets with high protein intake can increase SCFA and branch-chain amino acid (BCAA) production associated with anti-inflammation, as well as increase Bacteroides to support amino acid proteolysis4. As a result, high-protein diets low in carbohydrates are commonly employed in weight loss interventions. However, despite their potential anti-inflammatory benefits, such diets have also been associated with gastrointestinal consequences from increased fermentation of undigested protein and lowered gut microbial diversity25. Furthermore, an increased potential for BCAA production, particularly by Prevotella copri and Bacteroides vulgatus, along with increased serum BCAA was associated with increased insulin resistance in non-diabetic individuals24.
Calorie restriction
Apart from interventions targeting macronutrient composition such as fiber or protein, caloric restriction has long been employed as a primary weight loss tactic. Calorie restriction has been shown to increase microbial richness in individuals with low richness prior to the intervention26. However, despite initial weight loss from lower calorie intake, interventions involving considerable caloric restriction, specifically very-low-calorie diets (VLCD; ~800 calories/day), may be detrimental to the gut microbiome. For example, a liquid form VLCD in a clinical trial of women with overweight and obesity showed weight loss, decreased adiposity, and improved glucose regulation but also contributed to gut microbiota (reversible) compositional restructuring and an overall loss in bacterial abundance27. Further, when post-intervention microbiota from women in this clinical trial were transplanted to mice it induced weight loss while also impairing nutrient absorption, resulting in decreased bile acids27, which may ultimately increase the risk of Clostridium difficile colonization. In addition, there was a reversible increase in abundance of genes involved in SCFA biosynthesis but a decrease in SCFAs, potentially attributed to decrease in colonization during the VLCD period27. It has yet to be elucidated whether the weight loss and metabolic outcomes of a restrictive diet outweigh the potentially negative impacts of the gut microbiota and gut-brain axis27. Although changes in the gut microbiome from calorie restriction have been studied, their direct role in human physiology remains to be fully described28. Furthermore, the resiliency of these changes and their potential role in weight regain and weight loss maintenance is a priority, given that low-calorie diets often require dramatic and unsustainable changes in dietary intake that frequently lead to subsequent weight regain. For example, a 10-week weight-loss program for adults with overweight or obesity found that after the intervention, satiety signaling factors decreased and appetite-inducing factors increased for 1-year after program completion29. A generalizable relationship between dietary changes in gut composition and the gut-brain-axis has yet to be delineated4. Monitoring and targeting microbiota-gut-brain axis endocrine factors in weight management may present novel approaches to modulate hunger and satiety signaling and metabolism, thereby preventing weight regain and increasing the effectiveness of weight loss treatment.
Importantly, hunger and satiety signaling, as well as traits of eating behavior such as uncontrolled eating and restraint, are associated with dietary intake and gut microbiota composition. The existing evidence is reviewed in a later section.
Eating behavior
In addition to dietary intake, eating behaviors, such as eating frequency, are also targets for obesity prevention and treatment. For example, intermittent fasting (IF), a process in which food intake is restricted for 16–24 h at a time, is credited with inducing weight loss, improving insulin response, and reducing cardiometabolic disease risk30. These beneficial effects are considered only partially attributable to calorie restriction and are thought to result also from restructuring and remodeling of the gut microbiome from fasting behavior31,32. Other forms of IF include Ramadan IF (RI), an annual faith-associated fasting period prone to investigation for its regularity31. One such investigation found that fecal samples from RIF participants exhibited an increased microbiome diversity from gut remodeling, ultimately upregulating SCFA-producing capacity/species (butyric acid)31. Zouhal et al. found RIF, in a sample of males with obesity, to improve microbiota-gut-brain factors, including pre-to-post fasting leptin, GLP-1, PYY, and cholecystokinin, but found no effect on ghrelin33. Given its support of SCFA production and effect on microbiota-gut-brain factors, IF may be an effective strategy at improving body composition in populations with obesity by targeting the microbiota-gut-brain axis; however, more evidence is needed to understand what types of IF are most beneficial.
Probiotics, prebiotics, and symbiotics
Probiotics
Probiotics are live microorganisms that, when consumed in appropriate quantities, are expected to confer beneficial effects such as glycemic control through the introduction of beneficial species to the gastrointestinal tract34. Research suggests specific bacterial strains may reduce inflammation, leptin levels, and endotoxemia implicated in the microbiota-gut-brain axis pathology of metabolic diseases3. Bifidobacterium, Lactobacillus, and Akkermansia muciniphila are among the most promising species to have been tested as probiotics and to influence host metabolism3.
A comprehensive systematic review of randomized control trials investigating the anti-obesity properties of probiotic supplementation in overweight and obese populations concluded that high-dose probiotics are a promising intervention, with the most common significant outcome as a moderate but significant reduction in BMI (on average about half a kilogram across interventions)34. Potential underlying mechanisms include strengthening of the intestinal barrier, modulation of chronic inflammation, and production of metabolites that influence the microbiota-gut-brain axis34. There is also evidence, particularly from rodent studies, of probiotics having an effect in reducing anxiety and depressive symptoms4. The question of a potential effect on behaviors and psychological symptoms associated with obesity warrant investigation in humans. Additional factors that may be involved in the potential anti-obesity properties of probiotics include supplement amount, duration of usage, and strain specificity34. Since most studies use a mix of species in probiotics supplements administered such as VSL #3 (combination supplement of Lactobacilli, Streptococcus thermophilus, and Bifidobacterium), further research is needed to identify the best practice of probiotic supplementation for improving metabolic diseases35. Future investigations will need a heavier focus on human populations as findings from mouse models are not readily applicable to key human components, including the intestinal mucosa. In addition, there are unknown long-term effects of probiotic supplementation use and often contradictory evidence.
Prebiotics
Prebiotics are defined as “non-digestible food ingredients that beneficially affect the host by selectively stimulating the growth and/or activity of one or a limited number of bacterial species already established in the colon, and thus improve host health”8. Fiber intake, and specifically intake of microbiota-accessible carbohydrates, has been identified as a key dietary driver of gut microbiota composition and metabolite production. Dietary fiber includes prebiotics such as inulin, fructooligosaccharides (FOS), galactooligosaccharides, resistant starch, and other soluble dietary fibers, unhydrolyzable or unabsorbable by the small intestine. Common sources of dietary fiber include fruits, vegetables, and grains4.
Dietary fiber serves as a primary energy source for gut microbiota, which drives production of SCFA, primarily acetate, propionate, and butyrate, through fermentation. SCFAs have anti-inflammatory, immunomodulatory, and metabolic effects; however, there remains conflicting evidence regarding the role of SCFAs in metabolic diseases2. For example, studies in both rodents and humans have shown, on the one hand, that acetate suppresses appetite and is beneficial for metabolic health but on the other hand, may also have obesogenic and hyperglycemic effects2. These diverging effects may be related to the mode of acetate administration, but further research is needed to elucidate fully the role of microbe-produced acetate in human metabolic health. Although the role of SCFA is not completely understood, fiber intake and SCFAs potentially influence metabolic functions in the human host, including glycemic control, hunger and satiety signals to the brain, and inflammatory pathways.
Broadly, prebiotic intake is protective against metabolic diseases and results in favorable gut microbiota outcomes. Prebiotics have been shown to alter the gut composition by reducing Firmicutes and Bacteroidetes and increasing Lactobacillus and Bifidobacterium. Compositional changes correlated with improvements in microbiota-gut-brain factors (entero-endocrine cell activity, glucose homeostasis, and leptin sensitivity) important in addressing obesity8. Specifically, FOS (oligosaccharides commonly found in fruit) were used in a double-blind intervention study in women with obesity that found increased levels of Bifidobacterium and Faecalibacterium prausnitzii, which are often reduced in populations with obesity36. However, such studies examining metabolic health outcomes and microbiota compositional changes from fiber supplementation have yielded conflicting results and are scarce37. Inconsistent results of responses to fiber may be attributable to gut composition38. Thus, further evidence is needed to support recommendations of specific prebiotics for clinical applications.
Fiber intake is additionally associated with microbiota-gut-brain axis factors that could reduce inflammation and improve hunger and satiety signaling in obesity. The RESOLVE study involved participants with metabolic syndrome to a 3-week intensive diet-exercise residential intervention followed by a 1-year free-living period. Dietary fiber was the only nutritional component that was significantly predictive of health outcomes and decreased serum CRP levels, indicative of reduced inflammation. Therefore, its adequacy should be prioritized in future diet-weight reduction interventions39. Fiber may also play a role in increased brain-derived neurotrophic factor and appetite suppression; however, this is largely supported in mouse models with limited evidence in humans4. Existing human evidence stems primarily from observational research, thus, there remains a need to investigate and identify specific microbial metabolites and pathways involved in prebiotic impacts on the microbiota-gut-brain axis.
Symbiotics
Symbiotics are probiotic supplements that also contain prebiotic components25. A randomized control trial of participants with obesity and T2D found 24 weeks of symbiotics supplementation produced no significant changes in inflammatory markers when compared to the control group. The supplementation did induce changes in the gut microbiome by increasing counts of specific beneficial bacteria and altering the concentrations of acetic and butyric acids40. Furthermore, a randomized clinical trial (RCT) exploring symbiotic supplementation in individuals with T2D found significant reductions in bacterial translocation through the intestinal barrier, proposing combined symbiotic/probiotic and prebiotic supplement regimen as a potential therapeutic strategy to control low-grade inflammation40. However, studies on symbiotics are limited and evidence suggests other factors, including symbiotic administration timing, may be related to success.
Polyphenols
Polyphenols are noted for their anti-inflammatory and antioxidant capabilities, among many other properties (cardioprotective, cancer chemopreventive, and neuroprotective)41. These beneficial compounds contribute to human health through a bidirectional phenolic-microbiota pathway, producing bioactive metabolites and modulating gut microbiota composition. The functions of the gut microbiome and polyphenols are intertwined, as the colon’s microbiota provides enzymes essential for polyphenol metabolism, before their absorption41. Polyphenols impact microbiota composition by increasing Bifidobacteria, Lactobacillus, and F. prausnitzii, a butyrate producer, among others. Furthermore, polyphenols can increase A. muciniphila, which plays a role in insulin sensitivity7. Given their ability to selectively reduce the growth of particular species, polyphenols have been found to reduce the abundance of LPS producers and thus decrease metabolic endotoxemia7. Though evidence remains inconclusive, specific phenolic compounds have shown promise in their anti-obesogenic effects targeting the gut-brain axis42.
Notably, resveratrol, a natural polyphenol often found in grapes and berries, can mediate the microbiota-gut-brain axis through anti-inflammatory properties, GLP-1 secretion promotion, regulation of serotonin 5-hydroxytryptamine signaling, and modulation of gut microbiota composition. For example, resveratrol improves the integrity of intestinal tight junction proteins by influencing gut microbiota diversity, thus enhancing gut permeability function. Resveratrol stands as a promising future natural therapeutic for obesity-related intestinal dysfunction, but more evidence is necessary42. One investigation found ferulic acid (FA), a polyphenolic compound, prevented weight gain and attenuated dyslipidemia in mice. FA exerts hypolipidemic abilities by suppressing cholesterol synthesis and increasing the HDL/LDL ratio. Although slight changes in the gut microbiota structure were observed, the anti-obesity effects of FA were not associated with the changes in gut microbiota and diversity43. Therefore, FA may be a useful therapeutic target in treating obesity; however, its involvement in the microbiota-gut-brain axis still requires further research.
Polyphenols also play a role in SCFA production and their beneficial effects on metabolic health. Production of fecal succinate is induced by dietary polyphenols such as curcumin and dietary fiber (in rats)44. Succinate induces intestinal gluconeogenesis, which activates portal glucose signaling, which in turn decreases hunger and promotes insulin sensitivity. This phenomenon had also been described with propionate, showing that activation of intestinal gluconeogenesis was necessary for the beneficial effects of SCFA45.
The therapeutic potential of probiotics, prebiotics, symbiotics, and polyphenols, remains highly promising, yet underexplored in necessary experimental studies. Not only do they maintain the potential to treat metabolic disorders but also prevent their development, through mechanisms implicating the microbiota-gut-brain axis. However, there is a great need for well-designed RCTs in humans to understand further their role and effectiveness in targeting metabolic disorders and better characterize the underlying role of the microbiota-gut-brain axis in these processes.
Precision and personalized nutrition
Dietary interventions for obesity often fail to consider inter-individual heterogeneity in their design. Studies assessing fecal microbiota before and following intervention have found on the one hand, resilience in the core microbiome composition throughout time, and on the other, vastly different responses to identical dietary interventions, such that two individuals following identical dietary plans may experience drastically different physiological responses and changes to their gut microbiota46. Thus, dietary interventions tailored to individual gut microbiota composition may maximize metabolic benefits. To this point, Hjorth et al. found a Nordic diet yields a greater body-fat loss among individuals characterized by dominant Prevotella genera compared to Bacteroides-dominant microbiomes, illustrating differences in responsiveness to diets based on dominant taxa38. In view of this, precision or personalized nutrition (PN) seeks to customize dietary interventions for obesity by accurately predicting metabolic responses. PN utilizes dietary interventions predominantly focused in (1) increasing fiber intake, (2) restricting caloric intake, or (3) adding pre- and probiotics46. These tactics resemble generalized interventions; however, PN evaluates individual past responses to dietary interventions to develop a new diet plan, rather than the conventional one-size-fits-all approach. PN often evaluates the microbial response (e.g., increased abundance of Prevotella), changes in body weight, body composition, BMI, fat percentage, and other indicators of health such as the glycemic response47. Prior works have documented the accuracy and efficiency of algorithms and machine learning in large-scale implementation of personalized dietary interventions48. One such study developed an algorithm that significantly predicted blood sugar levels in response to prospective diet plans, over and above predictions formulated from dietary assessments such as the glycemic index49. Although the microbial response is a measure of PN intervention success, PN is still widely unexplored within the realm of the microbiota-gut-brain axis. In addition, more long-term studies are needed to examine long-term dietary change impacts on the core gut microbiota composition and corresponding biochemical profile regarding the gut-brain axis components (e.g., hunger hormone levels and inflammatory cytokines) in the context of obesity and T2D.
Additional practices: FMT, Bariatric surgery, and pharmacology
Fecal microbial transplant
Standard fecal microbiota transplant (FMT) in humans involves transferring intestinal microbiota from a donor to a recipient, typically through colonoscopy. A successful FMT is defined as the establishment of a donor-like microbiome in the recipient. FMT from a research perspective has enabled “humanization” of rodent models for mechanistic investigations of the human gut microbiome. This method has elucidated transferable behavioral phenotypes and has linked gut microbial composition to metabolic disorders4. Hartstra et al. found that donor FMTs from Roux-en-Y gastric bypass (RYGB) donors were capable of mediating the microbiota-gut-brain axis in humans with obesity. Those receiving the FMT exhibited altered dopamine and serotonin transporters and alterations in gut microbiota composition, underscoring the potential role of FMT in treating obesity by targeting the microbiota-gut-brain-axis1. However, FMT has primarily been used thus far in the treatment of Clostridioides difficile infection, though it holds significant promise in the treatment of other GI-related diseases and conditions. Further, autologous FMT, in which a patient has their fecal matter saved before surgery and receives their own “healthy” microbiota during their recovery, is considered a potential tactic to mediate individual changes in gut microbiota composition4.
Despite its currently limited clinical application, optimized FMT interventions remain a research focus for future use in the medical field. Example of such optimization is the use of broad-spectrum antibiotic cocktail pretreatments, which provide FMT-administered microbes a less competitive environment by depleting the recipient’s gut microbiota and increasing FMT efficacy4. The impact of FMT on microbiota-gut-brain axis components needs to be established.
Bariatric surgery
Bariatric surgery is now regarded as the most effective treatment for significant and sustained weight loss in severe obesity20. Multiple bariatric surgeries currently exist, with RYGB, adjustable gastric banding, and sleeve gastrectomy being the most common3. The BRAVE effect (alterations in bile flow and gastric size, anatomical changes, vagal nerve adaptations, and enteric gut hormone modifications) results from each type of bariatric surgery through a combination of anatomical rearrangements and changes to the digestive tract20.
Evidence suggests that bariatric surgery can impact host metabolism, gut hormone secretion, and insulin sensitivity through alterations in gut microbiota composition and resulting alterations in SCFA production50. Moreover, bariatric surgery can modify hormonal secretion and inflammation, thereby reducing adiposity, improving insulin sensitivity, and increasing microbiota diversity20. Human bariatric surgeries show increases in Gammaproteobacteria and Verrucomicrobia (Akkermansia), while abundance of Firmicutes has consistently decreased20.
In addition to compositional changes in the gut microbiota, the modulation of enteric hormones significantly influences the microbiota-gut-brain axis. Common modifications include reductions in ghrelin and increases in GLP-1 and PYY. RYBG has been shown to increase specifically GLP1 and PYY levels, while sleeve gastrectomy is associated with decreased ghrelin20.
Antibiotic use before bariatric surgery is a common practice but has the potential for both short-term and long-term impacts on the gut microbiota20. In addition, targeting the gut microbiota with probiotics, post-surgery, is thought to increase bacterial diversity and further benefit the host50. Mouse models have shown a connection between improved outcomes in diabetes and obesity post bariatric surgery and have suggested probiotic may be an effective strategy to improve obesity-related disease after bariatric surgery. However, this connection is understudied and has not yet been shown in humans20.
Therefore, not only does bariatric surgery provide the most significant weight loss outcomes, but it can also influence the microbiota-gut-brain axis. Bariatric surgery remains an important tool in addressing obesity and may provide a new perspective into the role of the gut microbiome in metabolic health.
Pharmacology
In addition to antibiotics, many non-antimicrobial drugs also influence gut microbiota composition, such as various hormones, antidepressants, antihistamines, among others. Forty-four drug categories were associated with impacting the gut microbiota, including metformin, statins, and laxatives51. Furthermore, the largest variance in fecal microbiome in healthy populations was attributed to medications in a combined analysis of the Belgian Flemish Gut Flora Project (n = 1106) and the Dutch LifeLines-DEEP (n = 1135) cohorts52. Current anti-obesity medications have also shown profound impacts on the gut microbiome composition, but they remain under explored in regard to the microbiota-gut-brain axis. Medications that often cause weight gain also cause variations in the gut microbiota4. Probiotic/prebiotic interventions could be explored as avenues for circumventing the negative side effects of medications on the gut microbiota and weight status4. While the microbiota-gut-brain axis remains a promising target for polypharmacy and targeted drugs to intervene in the pathology of obesity, current evidence is generally limited to animal models. Thus, well designed clinical trials targeting the microbiota-gut-brain axis with pharmacological approaches are warranted, and the impact of existing anti-obesity drugs on the gut microbiome should be further explored.
CONCLUSION
The microbiota-gut-brain axis provides an untapped potential target for therapeutic interventions in obesity. From a genetic standpoint, the gut microbiome is vastly larger than the human genome and is modifiable by a wide array of factors53. However, evidence is insufficient to identify the temporal associations between gut microbiota and obesity, and the relationship appears bidirectional20. Nevertheless, it is necessary to investigate the effects of predominant obesity prevention and treatment methods on the gut microbiota and gut-brain axis to understand mechanistic links and increase efficacy, and eventually effectiveness, of interventions. Current microbiota research is too limited to independently translate into clinical applications and inform recommendations. The future goals in this field include expanding the reach into diverse populations with a shift in focus to functional capacity of the gut microbiota to obtain a deeper characterization of the microbiota-gut-brain axis in obesity. Future research should also be proactive in including vulnerable populations often underrepresented in research, who bear the strongest burden of obesity-associated metabolic diseases.