Introduction
Most of the tsunamis are caused by earthquakes with magnitude >6.5 and are associated with reverse fault in an oceanic plate. In general, the tsunamigenic earthquakes are characterized by epicenters at <60 km depth (Bryant, 2008). The southwestern coast of Mexico has been affected by local as well as distal tsunamis linked to large earthquakes originated in the Cocos and Pacific plates. Tectonic activity in the Pacific coast of Central America and South America constitutes an additional source (Farreras et al., 2007). Subduction of the Cocos plate underneath the North American plate at a rate of ~5.3-5.8 cm/year (DeMets et al., 1994) causes the local tsunamis. Data provided by Singh and Suaréz (1986) suggest that the tsunamigenic earthquakes had a recurrence interval of ~34-38 years in the state of Oaxaca, ~32-56 years in the state of Guerrero and ~74 years in the state of Michoacán. In the last three centuries, the Pacific coast of Mexico witnessed at least 56 tsunamis (Sánchez and Farreras, 1993). Besides the relatively frequent <1 m high waves, the tsunami of November 16th, 1925, generated waves up to 10 m high in Zihuatanejo (Guerrero) (Borrero et al., 1997).
Tsunami waves generally leave sediments deposited along the coastal regions. Some of them are buried and preserve evidence of paleo-tsunami in the geological records. They also help to reconstruct recurrence interval of past tsunamis and extend the relatively short or non-existent historical records to the geological past. The observation of modern tsunami deposits provides clues to recognize paleo-tsunami events occurred over the last thousands of years. A tsunami deposit is usually identified by sedimentary context, i.e. larger grain size than the surrounding sediments indicating high-energy depositional conditions and spatial distribution. Depending upon the availability of sediments in the source region, the deposits can vary from fine sand to gravel. Tsunami sands overly peat and mud in coastal marsh stratigraphy (Atwater, 1987). Preservation of rooted plant material beneath the sand deposit indicates deposition of sand occurred after the subsidence (Atwater and Yamaguchi, 1991). Hutchinson et al. (1997, 2000) observed that the tsunami deposits in lakes usually consist of a bed of coarser sand layer between two organic rich finer mud layers. Sometimes the tsunami deposits are massive and may contain multiple fining upward sequences, e.g., the Cascadian margin (Benson et al., 1997). Tsunami deposits in Peru consists of multiple sand layers, rip-up clasts near the base of sand layers, erosional base, a mud layer between two sand layers, mud cap and normal grading (Jaffe et al., 2003). Apart from the sediment texture and structure, chemical composition (higher Br, Sr, and Na), abundance of heavy minerals and microfossils were used as proxies to distinguish the tsunami deposits (Goff et al., 2010, 2011; Morton et al., 2007; Roy et al., 2012; Ramírez-Herrera et al., 2012). However, chemical dissolution and diagenesis in sedimentary deposits of tropical regions reduce the possibility of persevering these proxies in geological records (Goff et al., 2011).
In this study, we present texture, mineralogy and chemical composition of sediments deposited by waves associated with the tsunami of September 21st, 1985, in Barra de Potosí village in southwestern Mexico. Calculation of hydraulic roughness of the terrain of inundation through estimation of the Manning’s number evaluates both the instrumental and eyewitness records of maximum wave height and inundation limit. We also compared results of this study with geological characteristics of sediments deposited by tsunamis of March 14th, 1979, and September 21st, 1985, in Zihuatanejo area (Ramírez-Herrera et al., 2012) and tsunami of March 11th, 2011, in several locations along the southwestern coast of Mexico (Roy et al., 2012).
Earthquake and tsunami of September 21 st , 1985.
Tsunamigenic earthquake of Mw 7.5 had its epicenter located at 17.6 km depth at the southeast ruptured region of Zihuatanejo with ~33 km length and ~66 km width (Mendoza, 1993). However, Corona-Esquivel et al. (1988) did not observe any uplifted segment near Zihuatanejo but noticed raised segments along the coast of Michoacan state (west and northwest of Guerrero state). The gauges of mareographic station at Acapulco registered tsunami waves with maximum height of 1.2 m and mean speed of 709 km/h (Sanchez Devora and Farreras-Sanz, 1993). The National Geophysical Data Centre of NOAA reports 1.2 m high waves in the Pacific Ocean and up to 2 run-ups. Recently, Ramírez-Herrera et al. (2012) reported that tsunami waves inundated the entire village of Barra de Potosí and identified the associated sand layer near Zihuatanejo (Figure 1).

Figure 1 Tectonic setting of southwestern Mexico and location of Barra de Potosí village. Sixteen different pits/ trenches are distributed at El Potosí estuary margins and in farmlands away from it. Sites of pits located in estuary margins (11, 12, 13, 14, 15 and 16) were inundated by the September 21st, 1985, tsunami waves and the reconstructed inundated area is shown in a dotted line.
The village of Barra de Potosí is located at a distance of ~25 km southeast of Zihuatanejo and at ~180 km northwest of Acapulco (Figure 1). El Potosí estuary is located at its southern limit and it is separated from the Pacific Ocean by a shallow spit barrier. It is connected to the open sea during the intervals of high tide. During both the field works (April, 2010 and February, 2011), we interviewed the residents with ages between 46 and 64 years. Apart the variable wave height (~2.5 to 10 m), all the eyewitnesses agreed that tsunami waves inundated up to a distance of ~500 m from the shore and transported fishing boats from the shore into the estuary. The sites located away from the El Potosí estuary (i.e., farmlands) were unaffected by inundation and there was no loss of human life in this village.
Material and methods
A total of 16 pits and trenches up to a maximum depth of 1.2 m were dug both parallel and perpendicular to the shore. Ten of them (1, 2, 3, 4, 5, 6, 7, 8, 9 and 10) are located away from the estuary and six (11, 12, 13, 14, 15 and 16) were dug in the margin of El Potosí estuary (Figure 1). The pits/trenches dug in the estuary margin were shallow, as the ground water did not permit to dig deeper. We observed sediments deposited by the tsunami in these 6 different pits/trenches. A total of 12 samples representing tsunami deposits (6 samples by avoiding superficial layer of pedogenesis) and sediments deposited in an environment prior to the tsunami (6 pretsunami samples) were collected. Additionally, 4 different samples from 4 out of 10 different pits located away from the estuary were collected. Sedimentological and geochemical analyses were carried out in all the samples and mineralogical analysis was done only in 4 different samples. Sedimentological and mineralogical analyses were performed after oven drying the samples at ~40°C and sieving through 230, 120, 60 and 40 meshes. For the sedimentological analysis, we calculated mean grain size, standard deviation, skewness and kurtosis (Wentworth, 1922).
Mafic and heavy minerals were identified in two tsunami and two pre-tsunami samples collected from pits 12 and 15. Samples were separated in terms of medium sand (1.25-2 Φ), fine sand (2-3 Φ) and very fine sand (3-4 Φ). In each fraction, heavy and mafic minerals with density >2.8 g/cm3 and lighter minerals with density <2.8 g/cm3 were separated. Minerals with density >2.8 g/cm3 were counted in each fraction under an optical stereoscopic microscope Leica Mz APO after preparing resin based polished sections and expressed as %. For the concentrations of 10 major element oxides (SiO2, TiO2, Al2O3, Fe2O3, MnO, CaO, MgO, Na2O, K2O, P2O5) and Bromine (Br), all the oven dried samples were ground and homogenized in an agate mortar and measured in a Siemens SRS 3000 X-ray fluorescence (XRF) spectrometer.
Results
Stratigraphy
Pits (1, 2, 3, 4, 5, 6, 7, 8, 9 and 10) located away from the estuary had similar stratigraphy and consist of homogeneous massive sand and occasional heavy mineral layers (Figure 2). Most of the pits lack any primary structure (i.e., lamination) and some have a ~10-15 cm thick heavy mineral layer (e.g., at ~95105 cm depth of pit 1). We did not observe any sedimentary unit with characteristics of tsunami deposits and consider that sediments of all these pits belong to the unaffected area.
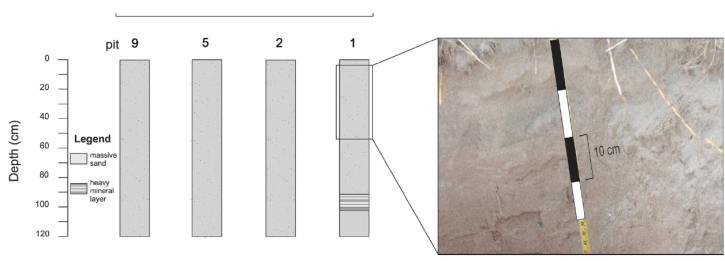
Figure 2 Stratigraphy of pits/trenches of sites located away from the estuary, Barra de Potosi, southwestern Mexico.
Pits located in the estuary margins (11, 12, 13, 14, 15 and 16) comprise of three distinct sedimentary units (Figure 3). The basal unit has organic rich dark gray sand and it is overlain by oxidized massive yellow sand with a transitional contact. The uppermost unit has massive gray sand with remnants of roots, plants and shell fragments. This unit has variable thickness (~15 cm in pit 15 to 70 cm in pit 13) and is characterized by an erosive base with respect to the underlying unit. Based on the stratigraphy, we interpret the uppermost massive sand unit with an erosive base as tsunami sediments and the underlying oxidized massive sand unit as pre-tsunami sediments.
Sediment texture
Table 1 and Figure 4 present texture of tsunami and pre-tsunami sediments and sedimentary deposits in the unaffected area.
Table 1 Texture of sediments deposited by tsunami waves of September 21st, 1985, pre-tsunami sediments and sediments deposited in unaffected area in the village of Barra de Potosí, southwestern Mexico (see Figure 1 for pit locations).
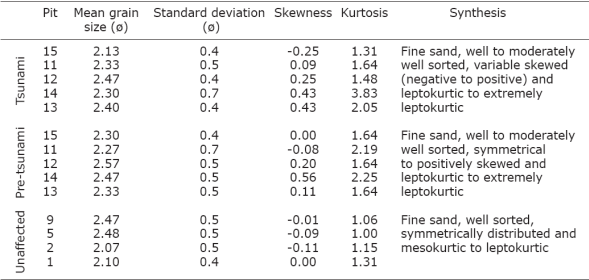
Tsunami sediments: Mean grain size varies between 2.13 and 2.47 Φ and standard deviation ranges between 0.4-0.7 Φ. Kurtosis ranges between 1.31 and 3.83 and skewness varies between -0.25 and 0.43. Sedimentological parameters suggest that tsunami sediments are well to moderately well sorted, fine sand with leptokurtic to extremely leptokurtic distribution and highly variable skewness. Sample with negative skewness has coarser fractions and samples with positive skewness contain higher amounts of finer silt and clay.
Pre-tsunami sediments: Texture of pretsunami sediments is similar to the tsunami sediments (Figure 4). Pre-tsunami sediments are also well to moderately well sorted (standard deviation: 0.4-0.7 Φ) fine sand (mean grain size: 2.27-2.57 Φ). Kurtosis (1.64-2.25) suggests leptokurtic to extremely leptokurtic distribution. Skewness ranges between symmetrical (-0.08) and positively skewed (0.56) and it suggests higher fractions of fine sand in some samples and more abundance of silt and clay in others.

Figure 4 Distributions of (A) mean grain size vs. standard deviation and (B) kurtosis vs. skewness in sediments deposited by tsunami waves of September 21st, 1985, pre-tsunami sediments and sediments deposited in unaffected area in the village of Barra de Potosi, southwestern Mexico.
Unaffected area: Sediments deposited in unaffected area are well sorted (standard deviation: 0.4-0.5 Φ), and fine sand (mean grain size: 2.10-2.50 Φ). All of them have mesokurtic to leptokurtic (kurtosis: 1.0-1.3) and symmetrical distribution (skewness: -0.11-0). Both of the parameters suggests that these sediments are better sorted compared to tsunami and pre-tsunami sediments and have higher fraction of fine sand (Figure 4).
Geochemistry
Table 2 presents chemical composition of tsunami and pre-tsunami sediments and sedimentary deposits in the unaffected area.
Table 2 Chemical compositions (oxides in % and Br in ppm) of sediments deposited by the tsunami waves of September 21st, 1985, pre-tsunami sediments and sediments deposited in unaffected area in the village of Barra de Potosí, southwestern Mexico (see Figure 1 for pit locations).
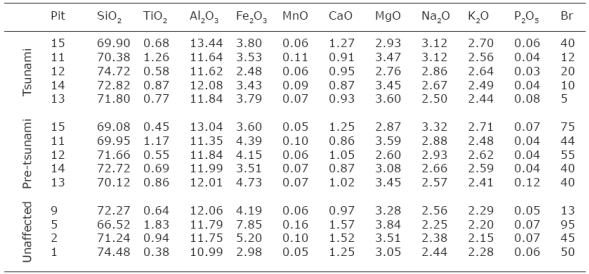
Tsunami sediments: Sediments have 69.90-74.72% of SiO2, 0.68-1.26% of TiO2, 11.62-13.44% of Al2O3, 2.48-3.80% of Fe2O3, 0.06-0.11% of MnO, 0.87-1.27% of CaO, 2.76-3.60% of MgO, 2.50-3.12% of Na2O, 2.44-2.70% of K2O, 0.03-0.08% of P2O5 and 5-40 ppm of Br.
Pre-tsunami sediments: In general, pretsunami sediments have less SiO2 and TiO2 and more Fe2O3 and Br compared to tsunami sediments (Figure 5).
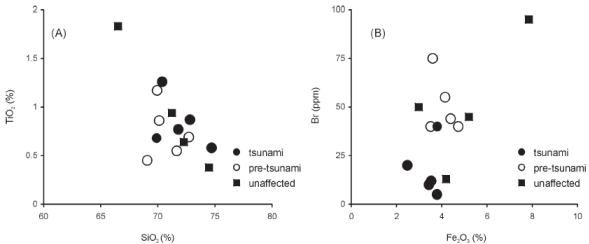
Figure 5 Concentrations of (A) SiO2 (%) vs. TiO2 (%) and (B) Fe2O3 (%) vs. Br (ppm) in sediments deposited by tsunami waves of September 21st, 1985, pre-tsunami sediments and sediments deposited in unaffected area in the village of Barra de Potosi, southwestern Mexico.
Contents of MnO, CaO, K2O and P2O5 are comparable both in tsunami and pre-tsunami sediments. Pre-tsunami sediments have 69.08-72.72% of SiO2, 0.45-1.17% of TiO2, 11.35-13.04% of Al2O3, 3.51-4.73% of Fe2O3, 0.05-0.10% of MnO, 0.86-1.25% of CaO, 2.60-3.59% of MgO, 2.57-3.32% of Na2O, 2.41-2.71% of K2O, 0.04-0.12% of P2O5 and 40-75 ppm of Br.
Unaffected area: Sediments deposited in unaffected area have more variable SiO2, TiO2, Fe2O3, MnO and Br compared to the tsunami and pre-tsunami sediments. However, concentrations of MgO, Na2O, K2O and P2O5 are homogeneous compared to both tsunami and pre-tsunami sediments. These sediments have 66.52-74.48% of SiO2, 0.38-1.83% of TiO2, 10.99-12.06% of Al2O3, 2.98-7.85% of Fe2O3, 0.05-0.16% of MnO, 0.97-1.57% of CaO, 3.05-3.84% of MgO, 2.25-2.56% of Na2O, 2.15-2.29% of K2O, 0.05-0.07% of P2O5 and 13-95 ppm of Br.
Mineralogy
Minerals with density <2.8 g/cm3 comprise quartz, feldspars, clay minerals and carbonates. The assemblage of minerals with density >2.8 g/cm3 consists of amphibole, pyroxene, epidote, lithic fragments, biotite, chlorite, garnet, zircon, sphene, rutile, apatite, sillimanite, tourmaline and chlorite. Both the tsunami and pre-tsunami sediments have similar associations and comparable abundances of mafic and heavy minerals with density >2.8 g/cm3 (Figure 6).
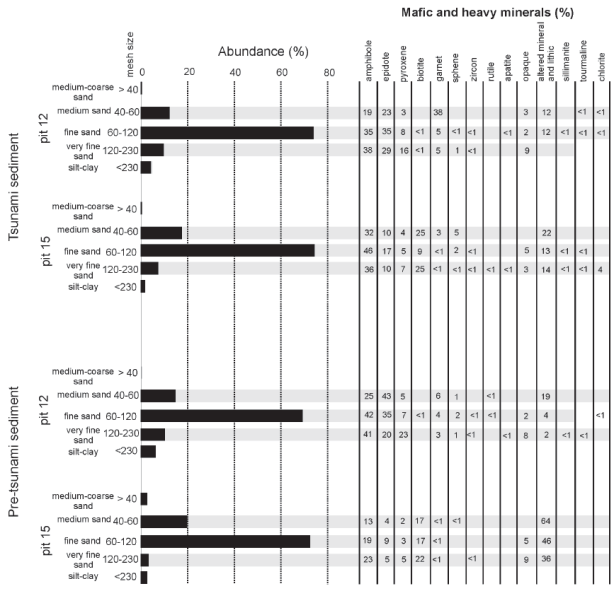
Figure 6 Mafic and heavy minerals identified in tsunami and pre-tsunami sediments collected from pits 12 and 15 in the village of Barra de Potosi, southwestern Mexico.
Tsunami sediments: Very fine sand fraction (24-26.5%) has relatively more mafic and heavy minerals compared to other fractions (medium sand: 3-7% and fine sand: 8-14%). Both the analyzed tsunami samples (pits 12 and 15) have similar assemblage of mafic and heavy minerals. Amphibole (20-45%), epidote (10-35%) and lithic fragments (10-25%) are more abundant compared to pyroxene (515%) and garnet (<5-40%). Zircon, sphene, rutile, apatite, sillimanite, tourmaline and chlorite are present in traces (<5%). Biotite is present as an abundant mineral (10-25%) in one of the samples (pit 15), whereas it is absent in other (pit 12). Similarly, abundances of amphibole, epidote and pyroxene are more in fine and very fine sand. Garnet is present in higher concentrations in the medium sand fraction.
Pre-tsunami sediments: In one of the samples (pit 12), abundance of mafic and heavy minerals is higher in very fine sand fraction (24.5%) compared to the rest (3-9%). Another sample (pit 15) has more mafic and heavy minerals both in very fine sand (30%) and medium sand (24.5%) fractions compared to fine sand (11%). Abundances of amphibole (15-40%), epidote (5-45%), pyroxene (<525%) and lithic fragments (<5-65%) are variable. Garnet, zircon, sphene, rutile, apatite, sillimanite, tourmaline and chlorite are present either as traces (<5%) or absent. Biotite is abundant (15-25%) in one sample and it is absent in another. Abundances of amphibole and pyroxene are higher in very fine sand. Lithic fragments are present in higher concentrations in the medium sand fraction.
Discussion
Limit of inundation and hydraulic roughness
Record of the National Geophysical Data Center reported maximum tsunami height of 1.2 m both in Acapulco and Zihuatanejo during the tsunami of September 21st, 1985, (http://www.ngdc.noaa.gov/nndc/struts/form?t=101650&s=167&d=166). It also documented inundation of 200 m inland at Zihuatanejo. However, the eyewitnesses claim that the minimum wave height was 2.5 m and the waves swept a distance of 500 m inland at Barra de Potosí. Similar to the eyewitness records, the sedimentary unit representing tsunami was observed at a distance of ~600 m inland near El Potosí estuary (Figure 1). We evaluate wave heights reported by instrumental record and eyewitness by using empirical formula for calculating the inland flooding limit (Xfl) of a tsunami (Hills and Mader, 1997; Figure 7):
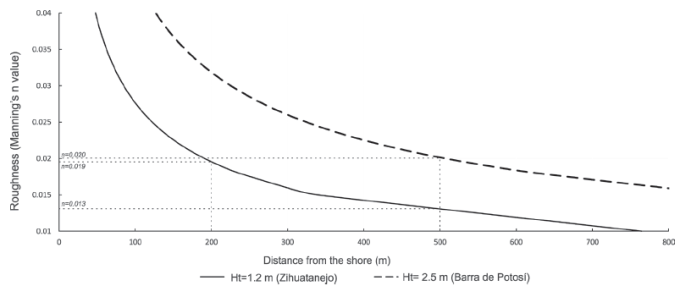
Figure 7 Calculation of Manning’s number indicating hydraulic roughness of the terrain affected by tsunami using instrumental (1.2 m) and eyewitness (~2.5 m) records of wave heights. The reconstructed inundated area is shown in dotted line in Figure 1.
Where Ht is tsunami height at coastline, n is Manning’s number and k=0.06 (a constant for tsunamis). Manning’s number is a coefficient expression of the micro-topography and sinuosity of the surface. It represents hydraulic roughness of the terrain that causes resistance to the water flow by creating retarding force (Chow, 2009). Arcement and Schneider (1989) estimated approximate values of Manning coefficient for different terrain typology and suggest that roughness of any terrain varies over time. Both river flood models and shallow water tsunami inundation models have incorporated the importance of varying roughness values for different land use types (Bricker et al., 2015).
A hydraulic roughness of ~0.02 was calculated to achieve an inundation limit of 200 m with a wave height of 1.2 m at Zihuatanejo (Figure 7). Roughness calculated for Barra de Potosí is also similar with a wave height of 2.5 m and an inundation limit of ~500 m. A Manning’s number of 0.02 represents farmland (Kotani et al., 1998) and lagoons have relatively lower hydraulic roughness (0.01-0.015; Arcement and Schneider, 1989). Similarly, rocky coasts, mangroves and forest have much higher roughness (> 0.047; Arcement and Schneider, 1989). However, both the eyewitness and stratigraphy of pits suggest that inundation of Barra de Potosí occurred from the open sea into the estuary (shown in a dotted line in Figure 1). Farmland located away from the estuary and parallel to the shore were not affected. Recalculation with an inundation limit of ~500 m and tsunami wave height of 1.2 m yield a Manning’s number of ~0.013 and this value is comparable for lagoons. We consider that eyewitness inundation limit is close to the real maximum inundation limit but it overestimates the wave height.
Geological characteristics of tsunami sediments and comparison
Tsunami deposit at Barra de Potosí is located in margins of the El Potosí estuary and it is characterized by massive well to moderately well sorted fine sand with an erosive base (Table 1, Figure 3). Sediments have variable skewness and leptokurtic to extremely leptokurtic distribution. They also contain shell fragments and remnants of roots and plants. Compared to sediments deposited in the unaffected area (well sorted fine sand), tsunami deposits are poorly sorted. However, the pre-tsunami deposits have sedimentological characteristics almost similar to tsunami deposits. They are characterized by well to moderately well sorted and leptokurtic to extremely leptokurtic oxidized fine sand but lack of shell fragments. Except for SiO2, TiO2, Fe2O3 and Br, both tsunami and pre-tsunami deposits have similar chemical composition (Table 2). Tsunami deposits have higher concentrations of SiO2 and TiO2, whereas pre-tsunami deposits have more Fe2O3 and Br (Figure 5).
Both the tsunami and pre-tsunami sediments have similar association and abundances of both mafic and heavy minerals (Figure 6). We did not observe higher abundance of any of the heavy minerals only in tsunami deposits compared to the pre-tsunami deposits. Higher abundance of garnet observed in medium sand fraction of tsunami deposit in one pit was not observed in tsunami deposit of the other nearby pit. Similar association of the mafic and heavy minerals (amphibole, epidote, pyroxene, biotite, garnet, sphene, zircon, rutile, apatite, sillimanite and chlorite) suggests that the tsunami as well as pre-tsunami deposits were reworked sediments sourced from similar provenances. Fluvial activity in the southwestern Mexico transports sediments into the coast and continental shelf by eroding the basement rocks (i.e., Xolapa and Guerrero complexes; Pérez-Gutiérez et al., 2009; Martini et al., 2010) and wave actions rework them from continental shelf or coast into the coastal lagoons during both tsunami and non-tsunami events.
Sedimentological and geochemical characteristics of tsunami and pre-tsunami deposits of the Barra de Potosí are compared with previously reported tsunami and pre-tsunami deposits from the southwestern Mexico in order to observe similarities and identify geological characteristics that might be useful to identify paleo-tsunamis in the region (Table 3). Ramírez-Herrera et al. (2012) reported sedimentological and chemical composition of deposits by the tsunamis of March 14th, 1979, and September 21st, 1985, by studying samples in a pit near Zihuatanejo. Similarly, Roy et al. (2012) analyzed grain size, mineralogy and geochemistry of sediments by collecting samples immediately after the March 11th, 2011, Japan tsunami in seven different sites along the southwestern coast of Mexico. We converted concentrations of main elements of Ramírez-Herrera et al. (2012) into oxides for comparison and observed anomalous values. In XRF analysis, concentrations of all the oxides together with loss on ignition should add up to 100%. Total concentrations of all main element oxides of Ramírez-Herrera et al. (2012) show much higher values (Table 3). As Ramírez-Herrera et al. (2012) overestimated Al2O3, Fe2O3 and K2O by 2 to 4 fold compared to the average continental crust (e.g., Taylor and McLennan, 1985), we consider that their elemental concentrations do not represent correct chemical composition of the tsunami sediments. However, we used their data in order to document the relative enrichment/depletion in tsunami and pre-tsunami sediments.
Table 3 Comparison between average chemical composition of sediments (n = number of samples) deposited during tsunamis of March 14th, 1979 (Zihuatanejo; Ramírez-Herrera et al., 2012) and September 21st, 1985 (Barra de potosi; this study, Zihuatanejo; Ramírez-Herrera et al., 2012) and March 11th, 2011 (along the coast of southwestern Mexico ; Roy et al., 2012).

All the tsunami deposits have relatively coarser sediments than those deposited in an environment previous to the high-energy events. Even though both tsunami (2.13-2.47 Φ) and pre-tsunami (2.27-2.57 Φ) deposits of Barra de Potosí are fine sand, there is slight difference in gran size. Tsunami sediments from the Zihuatanejo and Barra de Potosí are enriched in SiO2 and depleted in Fe2O3 compared to the pre-tsunami sediments. However, average values of sediments deposited along the southwestern coast during the March 11th, 2011, tsunami are depleted in SiO2 and enriched in Fe2O3 compared to pre-tsunami sediments. Tsunami deposits have more TiO2 at Barra de Potosí and less TiO2 at Zihuatanejo compared to pre-tsunami deposits. Compared to pretsunami deposits, tsunami deposits along the southwestern coast have more Na2O and Br, whereas sediments from Barra de Potosí have lower Br. Both Na and Br are soluble elements and reflect influence of the seawater through precipitation of salts (e.g., Peters et al., 2001). The difference between tsunami sediments of both studies might be due to the fact that samples of the March 11th, 2011, tsunami were collected immediately after the event, whereas samples of the September 21st, 1985, tsunami were collected after two decades. Interaction of tsunami sediments with rainwater might have washed away the salts and associated soluble elements. Ramírez-Herrera et al. (2012) reported higher concentrations of Ca, Sr and Ba in tsunami deposits of Zihuatanejo. However, some of the sediments deposited prior to both their tsunami events have either higher or equivalent concentrations of these elements. During the comparison, we did not observe any other geological characteristics that might be useful for identifying paleotsunamis in the region except for the grain size (i.e., coarser sediment) and stratigraphy (i.e., erosive base).
Conclusion
Sedimentological, mineralogical and geochemical characteristics of deposits associated with the tsunami of September 21st, 1985, were analyzed and compared with geological characteristics of both pre-tsunami deposits and sediments deposited in unaffected areas of Barra de Potosí village in southwestern Mexico. Both the tsunami and pre-tsunami sediments have similar sedimentological and mineralogical characteristics. Compared to pre-tsunami sediments, we observe higher concentrations of SiO2 and TiO2 in tsunami sediments. More specifically;
I. Tsunami deposits are massive well to moderately well sorted fine sand with shell fragments and contain remnants of plants and roots. They have leptokurtic to extremely leptokurtic distribution and are characterized by variable skewness.
II. Pre-tsunami deposits are also massive well to moderately well sorted sand but they are oxidized and lack of shell fragments. These sediments are also leptokurtic to extremely leptokurtic in nature.
III. Both the tsunami and pre-tsunami sediments have similar association and variable abundances of mafic and heavy minerals comprising amphibole, epidote, pyroxene, biotite, garnet, sphene, zircon, rutile, apatite, sillimanite and chlorite.
IV. Compared to the pre-tsunami deposits, tsunami deposits have more SiO2 and TiO2. Pre-tsunami deposits in general have higher concentrations of Fe2O3 and Br. V. A comparison with other studies of tsunami deposits in the region suggests that sediments associated with at least 3 different tsunamis (i.e., March 14th, 1979, September 21st, 1985, and March 11th, 2011) have different geochemical characteristics. Except for stratigraphy (i.e., erosive base), we did not observe any other geological characteristic useful for identifying paleotsunamis in the region.
VI. Absence of any characteristic mineralogical and geochemical signature for sediments associated with tsunami deposits in the southwestern Mexico suggests that both tsunami and non-tsunami sediments have similar provenances. However, higher concentration of some oxides might represent higher abundance of some minerals associated with higher energy events.