Introduction
The fact that humans and animals we usually come in contact with do sleep has been known since long time ago. Research has also been carried out in some invertebrate animals, although it has been of behavioral nature and not aimed at identifying the reason for sleep.
Kayser and Steiner-Kayser1,2 showed that, under certain conditions, bees lose muscle tone, drop the antennae and remain still, a behavior they interpreted as a state of sleep. In turn, Tobler investigated numerous invertebrate animals and described that they spent several hours of the day motionless; she explained that passiveness as the sleeping behavior.3-5 Moreover, in numerous investigations on the Drosophila fly, periods of immobility were also interpreted as equivalent to sleep. However, there is no evidence that these animals had the sleep characteristics clearly outlined by Hobson.6
Our working group was not particularly interested in sleep in the crayfish, but in brain’s processing of sensory signals and their effect on behavior. For that investigation, a freshwater crustacean, the crayfish, was used because its size facilitates the implantation of electrodes onto its brain and the performance of electrophysiological records while the animal seems to live a more or less normal life.
Crayfishes were kept in aquariums with tap water from which chlorine was removed by strongly stirring (“old water”), which appeared not to bother the crayfishes, which can remain for several months under these conditions, even if the water gets muddy with their excretions; the water was changed when the latter occurred. Occasionally, the water in the aquariums evaporated and the animals tended to lay on their side on the surface when only 5 to 7 cm of water were left, a behavior frequently observed by those who work with crayfishes (Fig. 1). Having no explanation for that position, we ventured several theories, including that they might be sleeping. However, that seemed so unlikely that we abandoned the idea.
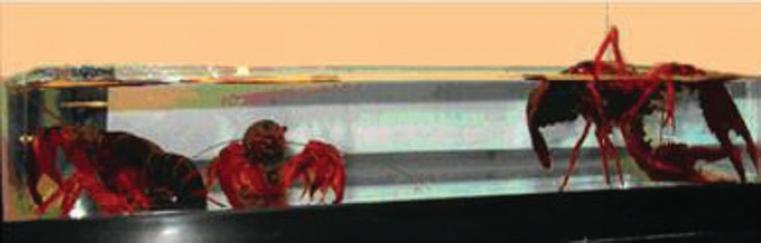
Figure 1 Crayfishes in a tank with vigorously agitated tap water and stored for later use. On the left, two crayfishes standing erected at the bottom of the aquarium. On the right, a crayfish lying in the water hanging on with its chelae.7
On one occasion, a crayfish with electrodes implanted onto the brain placed itself in that recumbent position; at that moment we thought it would be adequate to record its brain activity. Surprisingly, the oscilloscope records showed slow waves with a frequency of approximately 10 Hz. Shortly after, the animal regained its vertical position at the bottom of the aquarium and we observed the multitude of characteristic spikes of normal brain record. In view of this finding, we decided to wait for the crayfish to put itself on its side to obtain new records. We performed the corresponding tests on an animal while sleeping, to see if the pattern corresponded to that cerebral state (Fig. 2).

Figure 2 Records of a crayfish lying on the water surface. The black bar indicates the nocturnal period. A: Time during which the crayfish moves around the aquarium. Most movement corresponds to the hours around the light-dark and dark-light transitions; B: Intensity-duration curves obtained by applying vibratory stimuli when the animal is moving (curves 1-7). Curves 1-10 correspond to stimuli applied to animals standing still (8-11) and curve 12, to an animal lying on water; C: Records of the period during which the crayfish is lying on the water. A and C show an example among 12 recorded cases.7
Signal analysis
Initially, the records were analyzed using the fast Fourier transform to obtain frequencies, a strategy turned out not being the best for studying the encephalographic records, and thus using the wavelet transform was decided.
It should be remembered that the Fourier transform allows the analysis of a series of periodic signals, but only delivers a space of frequency and intensity. Conversely, the wavelet transform provides a much more complete space, where intensity, frequency and time coincide, and hence it is more useful for identifying where and when frequency changes occur in the brain records.
Sleep records
The first indications of a change in the cerebral state of the crayfish were postural change and immobility, as well as the change in the type of signals, which went from a continuous chain of spikes of between 20 and 50 µV in amplitude to a series of slow waves of between 10 to 20 cps (Fig. 3).

Figure 3 A: Photograph and electrophysiological record of a crayfish standing at the bottom of an aquarium. Note the spikes of up to 100-µV amplitude, corresponding to the cerebral field record; B: Crayfish lying on its side on the surface of the water in an aquarium and record of brain slow waves when the animal is in that position.7
In a subsequent stage, the crayfish brain was mapped to locate the region that produces the slow waves. The crayfish was placed in a tank with water where it was fixed to a stem leaving all its appendages free. We made a hole in the cephalothorax to expose the dorsal and anterior surface of the brain, in order to enable choosing the region to be recorded (Fig. 4).

Figure 4 Crayfish suspended in the water in a position that allowed electrophysiological record of the brain, while the animal was free to move the appendices. The recorded cerebral regions are indicated in the brain diagram (with symmetric dark dots from 1 to 8): E1 and E3, lateral protocerebrum; E2, protocerebral bridge; E4, central brain complex; E5 and E6, deutocerebrum accessory lobe; E7 and E8, tritocerebrum (image taken from Mendoza-Angeles et al.)8 The nomenclature is from Sandeman et al.9
To compare the sleep records of the crayfish with those of other animals, including humans, it is necessary to take into account various factors. Humans have two types of sleep, one called REM (rapid eye movements) and another non-REM (without rapid eye movements) during which dreams occur. Although it is possible for the crayfish to move some of its appendages, it is not possible to determine if it does, and this model would therefore be only applicable to the non-REM sleep state.
In summary, as it can be clearly appreciated in Figure 5, the origin of the transition from an active crayfish to a sleeping one occurs with the change of a record dominated by spikes to one with slow waves, which occurs in the central brain complex. Meanwhile, other brain regions continue with records dominated by unitary spikes. The central complex of the crayfish is homologous with that of insects.

Figure 5 Transition from active state to sleep in a suspended crayfish. A: Electric activity recorded in the central complex of the brain of a crayfish (E4) and in the deutocerebrum (E6) during the transition from wakefulness to sleep; B: Analysis by wavelet transform of the records in E4 and E6 (the gray boxes indicate the moment of transition). Before the transition (marked by the arrow), there are no changes in E4 (record and analysis by wavelet transform); conversely, in E6 there is a clear power decrease (gray box), corresponding to the appearance of slow waves and sleep onset (taken from Mendoza-Ángeles et al.10).