Servicios Personalizados
Revista
Articulo
Indicadores
-
Citado por SciELO
-
Accesos
Links relacionados
-
Similares en SciELO
Compartir
Revista mexicana de ciencias agrícolas
versión impresa ISSN 2007-0934
Rev. Mex. Cienc. Agríc vol.6 spe 12 Texcoco nov./dic. 2015
Articles
Evaluation of microalgae for the production of economically useful biomass using produced water
1Ingeniería de Sistemas de Producción, Universidad Autónoma Agraria Antonio Narro, Calzada Antonio Narro 1923, Buenavista. Saltillo. Coahuila, México. C.P. 25315. Teléfono: (844) 411-02-00, Ext. 2353 y 2354. (gsalash@live.com.mx; abenmen@uaaan.mx; azermeno@uaaan.mx).
2Biorganix Mexicana, S.A. de C.V. Dirección: Aiera No 240 Ramos Arizpe, Coahuila, México. C. P. 25903 Tel: (844) 488-26-27. (aortad@gmail.com).
3Departamento de Estadística y Cálculo, Universidad Autónoma Agraria Antonio Narro. (fel1925@yahoo.com).
The production of microalgae for biodiesel and other valuable products is a topic devoting great efforts. However, microalgae production requires large volumes of water and for its sustainable production requires searching for other water sources that do not compete with food production. In this sense produced water generated from the oil industry could be an alternative source of water, so for the present study the adaptation and growth of three microalgae strains halotolerant in two sources of produced water at the Sabinas basin Northeast Mexico were evaluated. It was found that one of the sources of produced water, in the Monclova 1 station, has potential for exploitation using microalgae Nannochloropsis sp. which showed greater adaptability to this water being a good candidate for further research. Moreover, I. galbana did not tolerate the presence of produced water in the medium; however, when treatments were planted again showed a positive population growth response, showing with this the bioremediation ability of this strain.
Keywords: Dunaliella; Isochrysis; Nannochloropsis; biodisel; bioremediation
La producción de microalgas para la obtención de biodiesel y otros productos de valor es un tema al que se le dedican grandes esfuerzos. Sin embargo, la producción de microalgas requiere grandes volúmenes de agua y para que su producción sea sustentable se requiere de buscar otras fuentes de agua que no compitan con la producción de alimentos. En este sentido las aguas producidas generadas por la industria petrolera podrían ser una fuente de agua alternativa por lo que para el presente estudio se evaluó la adaptación y el crecimiento de tres cepas de microalgas halotolerantes en dos fuentes de agua producida de la cuenca de Sabinas al noreste de México. Se encontró que una de las fuentes de agua producida, de la estación Monclova 1, tiene potencial para su aprovechamiento utilizando la microalga Nannochloropsis sp. que presentó mayor adaptabilidad a esta agua siendo una buena candidata para futuras investigaciones. Por otra parte, I. galbana no tolero la presencia de agua producida en el medio; sin embargo, al resembrar los tratamientos presento una respuesta de crecimiento poblacional positiva, lo que evidenció la capacidad de bioremediación de esta cepa.
Palabras clave: Dunaliella; Isochrysis; Nannochloropsis; biodiesel; bioremediación
Introduction
Produced water generated by the oil industry could be used as a culture medium for microalgae, which would allow using a resource that represents high costs for treatment and disposal during the extraction of hydrocarbons. Besides the benefits to be gained from biomass produced; microalgae can reduce the toxic load of some compounds present in these water (Bacellar-Mendes et al., 2013) that pose risks for pollution in the region where extracted. Bioremediation capacity of microalgae has been demonstrated in other effluents removing nitrogen and phosphorus from municipal wastewater (Shi et al., 2007) and some heavy metal discharges from the mining industry (Das et al., 2009).
Some microalgae are used to produce biodiesel (Duong et al., 2012) as these organisms represent 4 to 50% of lipids in their cells, besides of a high growth rate that is two to 10 times higher than land plants. This difference is explained by the fact that all cells are photosynthetic, being able to double the population in 6 to 12 h. Microalgae production can be carried throughout the year when the conditions of temperature, lighting, water availability and nutrients are adequate, do not compete with food production and can be grown in wastewater taking advantage of dissolved nutrients present on this (Subramanian et al., 2013).
Water requirements for the production of microalgae are considerable. Yang et al. (2011) estimated that to produce 1 kg of biodiesel requires 3 726 kg of water; however, if seawater or wastewater is used as culture media for the production of the biomass, these requirements are reduced by up to 90% and reduces the need for certain nutrients. This alternative of using wastewater for the production of microalgae to produce biodiesel has been studied by several researchers (DOE, 2010); however, there is still little information for the use of water produced as culture medium for microalgae.
Using produced water obtained after a treatment Arriada and Abreu (2014) achieved to grow microalgae Nannochloropsis oculata, while Sullivan et al. (2011) were able to grow Nannochloropsis salina without water treatment, using only a mix of water sources to obtain certain level of salinity. Meanwhile Hamawand et al. (2014) evaluated the possibility of using water drawn with gas associated to coal (Coal Seam Gas) to grow microalgae, concluding that it is feasible to apply a pre chemical treatment of water with acetic acid.
Produced water contains dissolved salts, such as sodium and calcium chloride, sodium carbonate, potassium chloride, calcium sulfates or barium, among others; it may even contain metal ions. Produced waters originally are congenital waters located in the deeper stratas of geological formations (Martel-Valles et al., 2013). When extracted during the gas or oil production process are added various substances such as surfactants, gels and inhibitors to facilitate the extraction and it is then that its composition is modified and is called produced water (Veil et al., 2004). Its composition varies widely between extraction sites even with nearby sites and most produced water has higher salinity than seawater (Martel-Valles et al., 2013), so its use for development of halotolerant algae is sought.
In this study tolerance, growth and the possibility to obtain usable biomass from three marine microalgae (Isochrysis Galvana, Dunaliella tertiolecta and Nannochloropsis sp.) were evaluated using produced water obtained from Sabinas Basin in northeastern Mexico, as an alternative to use this water resource potential.
Materials and methods
For this study, produced water from stations wells Monclova 1 and Buena Suerte located in the Sabinas Basin were used, which received contributions of water from up to 25 adjacent wells to each station and were previously characterized by Martell-Valles et al. (2013).
Microalgae evaluated Dunaliella tertiolecta, Isochrysis galbana and Nannochloropsis sp. obtained from the Institute of Oceanology Research of the Universidad Autonoma of Baja California, Ensenada, BC The experimental work was carried out at the premises of Biorganix Mexicana S. A. de C. V. in the municipality of Ramos Arizpe, Coahuila, Mexico.
The material was previously autoclaved, salt water was filtered with millipore filter of 0.22 µm and sowing was performed under a laminar flow hood previously sterilized in presence of flame. Experiments were performed in 250 ml erlenmeyer flasks with 90 ml culture medium for control and treatments. Seawater was prepared with artificial sea salt (Instant Ocean) dissolving 35 g of salt per liter of distilled water, the nutrient medium F/2 Guillard (Guillard and Ryther, 1962) was used in all treatments.
The experimental units were kept in a growth chamber at 20 ±1 °C with photoperiod of 12:12 h light:dark illuminated with light emitting diodes (LED) and halogen lamps with an emission of 7.7 and 14.3 µ mol m-2 s-1 respectively and only extracted to take samples for population counts, measuring pH and electrical conductivity (EC), thereafter returning them to their original location. Under these conditions were kept the primary cultures, which were renewed every 8 days taking 15 ml of inoculum and growing it in 135 ml of artificial seawater with culture medium F/2 keeping them in exponential growth phase. From these crops 10 ml inoculum to sow treatments were taken. Sowing density was dependent on strain size keeping the highest densities with Nannochloropsis sp followed by Isochrysis galbana and lowest with Dunaliella tertiolecta (5.53 x 105, 2.74 x105and 0.56 x 105 cells ml -1 respectively). All treatments were made by triplicate.
Population counts were made every 2-3 days performing three counts in Neubauer chamber by experimental unit with a phase contrast microscopy. Mobile strains were previously fixed with a solution of 4% formaldehyde for counting (FAO, 1996). pH and EC measurements were made with a potentiometer and conductivity meter Horiba Laqua twin models B713 and B771 respectively. PAR radiation was measured with a photometric sensor Li-cor quantum Q40808 and dataloguer LI-1400.
To calculate the specific growth rate the equation described by García et al. (2007) was used, the experimental design was randomized complete block to minimize the differences in growth that could be caused by the lighting conditions in the growth chamber. Mean comparison test Duncan. Data was analyzed using "R" (R Development Core Team, 2013).
To evaluate the capability of microalgae to tolerate and thrive in produced water the work was divided into two experiments (Table 1), the treatments were sow again after 7 to 10 days using the initial sowing as a pretreatment to reduce possible toxic load supplied by produced water (Das et al., 2009; Bacellar-Mendes et al., 2011). Produced water dilutions and concentrations used were selected based on preliminary test. The growth rate in the second experiment phase was estimated at 4 time periods, 0-9, 9-18, 18-29 and 29-36 days after the experiment began.
Table 1 Treatment preparation of the two experimental stages; pH and EC correspond to that recorded in the treatments before planting.

AP= agua producida, CE y pH registrados en los testigos y tratamientos antes de inocular las microalgas; BS= agua producida de la estación Buena Suerte; M1= agua producida de la estación Monclova 1.
Results and discussion
Experiment 1: Selection of produced water
In water treatment from Buena Suerte at 5% reported a change in pH from 6.8 to slightly above 8 after two days of exposure (Table 2), EC from the treatments showed a slight decrease of just over 2 mS cm-1 towards the end. All strains flocculated forming a precipitate in the bottom of the flask (Figure 1), without achieving growth of microalgae when culture medium was re-inoculated and without identifying the flocculant. Flocculating microalgae could be due to the presence of metal ions, which have the ability to neutralize cell surface negatively charged, some ions having this effect in microalgae derive from aluminum sulfate and ferric chloride (Udhaya et al., 2014), the pH of the culture medium not only affects the charge of cell surface of microalgae, but also the action of chemical flocculants. The decrease of EC in this case could be due to precipitation of some ions along with the flocculation of microalgae. The increase in pH can be explained by the contribution of OH- by the cells of living and dead microalgae, as has been reported in natural environments (Li et al., 2008).
Table 2 pH and EC variation during exposure at 5% of produced water in experiment 1.

D.t= D. tertiolecta; I.g= I. galbana y N. sp.= Nannochloropsis sp.
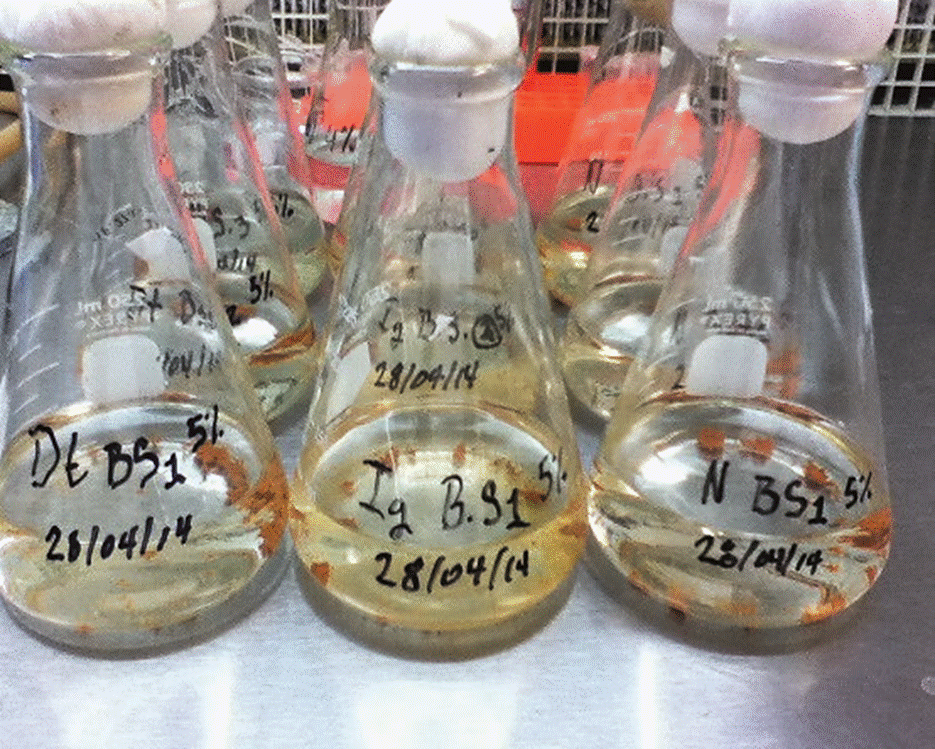
Figure 1 D. tertiolecta, I.galbana and Nannochloropsis sp flocculation in treatment with 5% of produced water from Buena Suerte in experiment 1.
When treatments were sown again with produced water from Buena Suerte staion at seven days there were no changes EC and pH; however, there was no growth in any of the three strains. Martel-Valles et al. (2013) reported higher average on middle petroleum fraction content in produced from Buena Suerte (103.2 mg ml-1). Toxic effects of diesel have been reported in marine microalgae at concentrations of 1 to 5 ppm (Mahoney and Haskin, 1980), so the presence of middle petroleum fraction thus the presence of flocculating agents in produced water from Buena Suerte station, could be the main factors limiting its use to grow microalgae.
Furthermore by preparing treatments at 5% of produced water from Monclova 1 station an EC of 54 mS cm-1 and a pH of 7.9 was recorded. EC of these treatments was above 46.2 mS cm-1 recorded in the culture medium for control, being this a hyperosmotic medium which could be a stress factor coupled with the presence of other components such as metals and hydrocarbons in produced water. In treatments with I. galbana and Nannochloropsis sp. pH showed a slight decrease at the beginning of the experiment and increased slightly over 8 towards the end of this, this variation in pH could be the result of exchanging ions of cells with hyperosmotic medium.
High salinity causes ionic imbalance to cells by water loss, causing an overproduction of species reactive to oxygen that causes oxidative stress. Resilience capacity to stress varies from one species of microalgae to another and involves the induction of antiporters as those of H+/Na+ and the protection of photosynthetic systems with antioxidants (Martinez-Roldan et al., 2014).
By re-sowing the treatments with produced water from Monclova 1 station with 10 ml of inoculum achieved populational growths of I. galbana and Nannochloropsis sp. (Table 3), this growth could be due to a decreased toxic load by the effect of bioremediation from microalgae at the beginning of the experiment. Among the physicochemical characteristics of produced water from the Monclova 1 station reported by Martel-Valles et al. (2013), which could limit the growth of microalgae, the large amount of total dissolved solids (153,000 mg L-1) and the presence of heavy metals (Pb, 1.77; Ni, 1.22; Cd, 0.37; Cu 0.148 and Cr, 0.39 mg L-1). Bioremediation with microalgae of waters contaminated with heavy metals occurs in two phases, the first is adsorption, a biophysical process that can be completed in seconds to a few minutes and can be carried out even in non-viable cells, then absorption occurs which is internalisation of metal ions into cell cytoplasm and is dependent on metabolism (Dwivedi, 2012).
Table 3 Growth rates in exposures to produced water from Monclova 1 station at 5% (Stage 1), the treatments showed population growth after replanting the treatment.

*D tertiolecta no mostró crecimiento en esta etapa. TEC= tasa especifica de crecimiento.
Considering the results obtained it was decided to continue experimenting with produced water from Monclova 1 station and discard the produced water from Buena Suerte station, because the latter would require different strategies to find its use as culture medium. Despite not having obtained growth of D. tertiolecta in control by selecting produced water, the strain was considered for the next stage as the results obtained for this strain cannot be fully explained by treatments with produced water. It is likely that D. tertiolecta did not adapt to low lighting.
This lighting was handled as it could increase the possibilities of adaptation to these waters taking into account that the increase of this as for temperature could increase the toxicity caused by hydrocarbons in some algae (Lewis and Pryor, 2013). In this regard Martel-Valles et al. (2013) reported for produced water from Buena Suerte and Monclova an oil content of petroleum middle fraction of 103.2 and 1.8 mg L-1 and fats and oils of 18.1 and 10.4 mg L-1 respectively.
Experiment 2: viability of microalgae in the presence of produced water from Monclova 1 station
Microalgae interaction with the environment was evidenced by changes in pH and EC of the treatments. The most marked changes occurred in the first four days of exposure (Figure 2), where pH decreased after planting and increased towards the fourth day with a steeper decrease in the concentration of 7% of produced water, being in this case slower the increase of pH. This variation in pH is similar to that found in stage 1, only that in this time it was detected in all three strains. Moreover, EC showed fluctuations in the first four days with a tendency to decline and with the most pronounced fluctuations in the concentration at 7% of produced water. These changes do not directly reflect population growth rate of the strains under study (Table 4), but are the result of the physicochemical and metabolic interactions of cells with treatment.

Figure 2 Changes in pH and EC, recorded during experiment 2 in exposures at 3, 5 and 7% of produced water from Monclova 1 station.
Table 4 Comparison of pecific growth rate of the three strains exposed at 3, 5 and 7% of produced water from Monclova 1 station in four time periods within experiment 2. D. p: (Dunaliella tertiolecta), I. g (Isochrysis galbana) N. sp.= (Nannochloropsis sp.). Equal letters without significant differences within time period (Duncan p> 0.05).

The first response of microalgae when exposed to the culture medium is the interaction of the cell wall with dissolved ions, as a tendency to dehydration and cell shrinkage if the medium is hyperosmotic, within this stabilization process of cells regarding to the medium could orginate the changes related to pH and EC. The greater the concentration of produced water, the metabolic demand was higher for microalgae to maintain their homeostasis, this was reflected in the growth rate achieved at nine days in D. tertiolecta and Nannochloropsis sp., where the best growth rate was recorded in control followed by treatment of D. tertiolecta 3%. The lowest growth rates and no significant differences were detected within this period in Nannochloropsis sp at 3, 5 and 7% and D. tertiolecta at 5 and 7%. On the other hand I. galbana showed no growth in any of the treatments except for control in the first 9 days of the experiment (Table 4).
After replanting the treatments there was a better response from I. galbana in treatments with 3 and 5% of produced water, while at 7% of produced water this strain only showed growth until the third period of time (18 to 29 days), having achieved growth in the three treatments although with a final cell density with significant differences between each treatment and smaller as concentration of produced water increased (Table 5). This same effect of decreased cell concentration in response to increased concentration of produced water was detected with D. tertiolecta, only that growth was recorded during the first time period (0 to 9 days).
Table 5 Maximum cell concentration obtained at the end of experiment 2 in exposures at 3, 5 and 7% of produced water from Monclova 1. Equal letters without significant differences (Duncan p> 0.05).

For D. tertiolecta after replanting the treatments no substantial improvement was detected in growth rate, a declining population when exposed to 7% of produced water, suggesting that a deficiency was generated in this strain, possibly by the interaction of the nutrient medium with produced water, than a toxic effect. The best response to presence of produced water was detected with Nannochloropsis sp. showing a positive growth rate in treatments throughout the experiment, although very low compared with that obtained with control in the first period, remained constant obtaining a higher cell density in the control followed by treatment at 7 and 5%, (Table 5).
The toxicity induced by heavy metals dissolved in culture medium is in function of cell density. Debelius et al. (2009) used the term toxic cellular quota as the mass of metal per cell, in the case of Cu++ by increasing cell density less copper is adsorbed per unit area of cell wall surface, so there is less disorder in cell division, this would explain the increased toxicity of the metal by decreasing cell density. The sowing density was much lower in treatments of Dunaliella t (0.86 x105 cel ml -1) than with Isochrysis g (5.46 x105 cel ml -1) and that Nannochloropsis sp. (22.96 x105 cel ml -1), this for the big difference in cell size between the three strains, which could be an advantage for smaller microalgae when interacting the cell surface with an adverse medium by presenting a higher adsorption surface per unit biomass.
Although in this study were not identified the factors of produced water that caused a toxic effect on microalgae, the interaction of microalgae was evident and mainly from Isochrysis g with this possible factor or toxicity factors that somehow was mitigated by the Initial planting and allowed the growth of the same strain when replanted the medium.
In this study highlights the results obtained with Nannochloropsis sp. and in this regard Roleda et al. (2013) points out Nannochloropsis oculata as the most robust strain among six species of oleaginous microalgae to grow and accumulate lipids over a wide range of environmental conditions. In the results obtained by Arriada and Abreu (2014) with Nannochloropsis oculata exposed to produced water in Brazil at 50 and 100% reported growth rates of 0.12 and 0.06 respectively, whereas control recorded a growth rate of 0.13, with an experimental period of 18 days. In this study the maximum growth rate of 0.19 in the first period (Table 4) was obtained with control, while treatments showed their maximum growth rate in the time periods 2 and 3 being 0.1, 0.078 and 0.089 for treatments at 3, 5 and 7%, with an experimental period of 36 days. The variability of the physicochemical characteristics from produced water is a determining factor for their possible use in the production of microalgae, as it can be appreciated in the results obtained in this work with the produced water from Buena Suerte and Monclova 1 station and its contrast with the results of the research with produced water in Brazil.
Conclusions
The option of produced water from Monclova 1 station has greater potential for use than produced water from Buena Suerte station. Isochrysis galbana strain had the best recovery after replanting the treatments at 3, 5 and 7% of produced water from Monclova 1 station, so its potential could be for bioremediation. Isochrysis galbana and Dunaliella tertiolecta decreased their growth rate and final population density in direct relation with the increase in the concentration of produced water unlike Nannochloropsis sp, which had the lowest cell concentration in the treatment with the lowest concentration of produced water, which makes it a good candidate for further studies for use of this resource.
Literatura citada
Arriada, A. A and Abreu, P. C. 2014. Nannochloropsis oculata growth in produced water: an alternative for massive microalgae biomass production. J. Pet. Gas. 8(3):119-125. [ Links ]
Bacellar-Mendes, L. B.; Rodrigues-Cunha, P. C.; Montes D’Oca, M. G.; Abreu, P. C. and Primel, E.G. 2011. Method for removing pollutants from produced water. United States Patent No. US 7955505 B2. [ Links ]
Das, B.K.; Roy, A.; Koschorreck, M.; Mandal, S. M.; Wendt-Potthoff, K. and Bhattacharya, J. 2009. Occurrence and role of algae and fungi in acid mine drainage environment with special reference to metals and sulfate immobilization. Water Res. 43(4):883-894. [ Links ]
Debelius, B.; Forja, J. M.; DelValls, A. and Lubián, L. M. 2009. Toxicity and bioaccumulation of copper and lead in five marine microalgae. Ecotoxicol. Environ. Saf. 72(5):1503-1513. [ Links ]
Department of Energy (DOE). 2010. National Algal Biofuels Technology Roadmap. U.S. Department of Energy, Office of Energy Efficiency and Renewable Energy, Biomass Program. Fishman, D.; Majumdar, R.; Morello, J.; Pate, R. and Yang, J. (Eds). College Park, Maryland, U.S. 140 p. [ Links ]
Duong, V. T.; Li, Y.; Nowak, E. and Schenk, P. M. 2012. Microalgae isolation and selection for prospective biodiesel production. Energies. 5:1835-1849. [ Links ]
Dwivedi, S. 2012. Bioremediation of heavy metal by algae: current and future perspective. J. Adv. Lab. Res. Biol. 3(3):195-199. [ Links ]
Food and Agriculture Organization (FAO). 1996. Manual on the production and use of live food for aquaculture. In FAO Fisheries Technical Paper. Lavens, P. and Sorgeloos, P. (Eds). Rome, Italy. 361:295 p. [ Links ]
García, F.; Freile-Pelegrín, Y. and Robledo, D. 2007. Physiological characterization of Dunaliella sp. (Chlorophyta, Volvocales) from Yucatan, Mexico. Bio. Technol. 98(7):1359-1365. [ Links ]
Guillard, R. R. and Ryther, J. H. 1962. Studies of marine planktonic diatoms. Can. J. Microbiol. 8(2):229-239. [ Links ]
Hamawand, I.; Yusaf, T. and Hamawand, S. 2014. Growing algae using water from coal seam gas industry and harvesting using an innovative technique: A review and a potential. Fuel. 117:422-430. [ Links ]
Lewis, M. and Pryor, R. 2013. Toxicities of oils, dispersants and dispersed oils to algae and aquatic plants: review and database value to resource sustainability. Environ. Pollut. 180:345-367. [ Links ]
Li, S. X.; Hong, H. S.; Zheng, F. Y. and Deng, N. S. 2008. Effects of metal pollution and macronutrient enrichment on the photoproduction of hydroxyl radicals in seawater by the alga Dunaliella salina. Mar. Chem. 108:207-214. [ Links ]
Mahoney, B. M. and Haskin, H. H. 1980. The effects of petroleum hydrocarbons on the growth of phytoplankton recognized as food forms for the eastern oyster, Crassostrea virginica GMELIN. Environ. Pollut. 22:123-132. [ Links ]
Martel-Valles, J. F.; Benavides-Mendoza, A.; Valdez-Aguilar, L. A.; Juárez-Maldonado, A. and Ruíz-Torres, N. A. 2013. Effect of the application of produced water on the growth, the concentration of minerals and toxic compounds in tomato under greenhouse. J. Environ. Prot. 4(7):138-146. [ Links ]
Martínez-Roldán, A. J.; Perales-Vela, H. V.; Cañizares-Villanueva, R. O. and Torzillo, G. 2014. Physiological response of Nannochloropsis sp. to saline stress in laboratory batch cultures. J. Appl. Phycol. 26(1):115-121. [ Links ]
R Development Core Team. 2013. R: A language and environment for statistical computing. R Foundation for Statistical Computing, Vienna, Austria. URL http://www.R-project.org/. R Found. Stat. Comput. Vienna, Austria. [ Links ]
Roleda, M. Y.; Slocombe, S. P.; Leakey, R. J. G.; Day, J. G.; Bell, E. M. and Stanley, M. S. 2013. Effects of temperature and nutrient regimes on biomass and lipid production by six oleaginous microalgae in batch culture employing a two-phase cultivation strategy. Bio. Technol. 129:439-449. [ Links ]
Shi, J.; Podola, B. and Melkonian, M. 2007. Removal of nitrogen and phosphorus from wastewater using microalgae immobilized on twin layers: an experimental study. J. Appl. Phycol. 19:417-423. [ Links ]
Subramanian, S.; Barry, A. N.; Pieris, S. and Sayre, R. T. 2013. Comparative energetics and kinetics of autotrophic lipid and starch metabolism in chlorophytic microalgae: implications for biomass and biofuel production. Biotechnol. Biofuels. 6:150 p. [ Links ]
Sullivan, E. J. J.; Dean, C.; Laur, P. and Viszolay, A. 2011. Treatment and use of oil and gas produced water as a media substrate for algae cultivation for biofuels. In: 56th Annual New Mexico Water Conference. “New Water New Energy: A Conference Linking Desalinitation and Renewable Energy”. Dec. 13-14, 2011. Alamogordo, New Mexico, U.S. [ Links ]
Udhaya, R. L. B. B. and Sandhya, S. 2014. Evaluation of chemical flocculation-electro flocculation for harvesting of halotolerant microalgae. Int. J. Environ. Sci. 4:899-905. [ Links ]
Veil, J. A.; Elcock, M. G. P. D.; Robert, J. and Redweik, J. 2004. A white paper describing procedure water from production of crude oil, natural gas, and coal bed methane. U.S. Department of Energy National Energy Technology Laboratory. 87 p. [ Links ]
Yang, J.; Xu, M.; Zhang, X.; Yang, J.; Xu, M.; Zhang, X.; Hu, Q.; Sommerfeld, M. and Chen, Y. 2011. Life-cycle analysis on biodiesel production from microalgae: water footprint and nutrients balance. Bio. Technol. 102:159-165. [ Links ]
Received: September 2015; Accepted: December 2015