Servicios Personalizados
Revista
Articulo
Indicadores
-
Citado por SciELO
-
Accesos
Links relacionados
-
Similares en SciELO
Compartir
Agrociencia
versión On-line ISSN 2521-9766versión impresa ISSN 1405-3195
Agrociencia vol.51 no.1 Texcoco ene./feb. 2017
Natural Renewable Resources
The influence of climate on Pinus hartwegii Lindl. recruitment at the alpine tree line ecotone in Monte Tlaloc, Mexico
1Instituto de Ciencias Agropecuarias y Rurales (ICAR), Universidad Autónoma del Estado de México (UAEMex). Unidad El Cerrillo Piedras Blancas, Estado de México. 50090. Toluca, Estado de México. México.
2Instituto Nacional de Investigaciones Forestales, Agrícolas y Pecuarias, Centro Nacional de Investigación Disciplinaria Relación Agua-Suelo-Planta-Atmósfera (INIFAP-CENID-RASPA). Margen Derecha del Canal Sacramento Km 6.5. 35140. Gómez Palacio, Durango. México.
3Centro Interamericano de Recursos del Agua (CIRA), Facultad de Ingeniería, Universidad Autónoma del Estado de México (UAEMex). Carretera Toluca-Atlacomulco Km. 14.5, Unidad San Cayetano. 50200. Toluca, Estado de México. México.
The upper timberline in Mexico is expected to be sensitive to climate change. Therefore, Pinus hartwegii recruitment at Monte Tlaloc’s tree line ecotone, in the Estado de Mexico, was analyzed, in order to detect a possible progress in the altitude interval of the species, as a result of higher temperatures. To explore the influence of climate in the recruitment pattern, we compared the age frequency of P. hartwegii with temperature and rainfall; additionally, we analyzed this impact on the basis of the year to year change in the thickness of their growth rings and its relationship with the El Niño-Southern Oscillation (ENSO). The age frequency was based on a 269 trees simple; their growth rings were dated a year after they were formed. P. hartwegii recruitment has increased since the late 1970s, which matches the rise in temperature, especially during El Niño events. This situation was made evident by a lower radial increase than the mean of trees found below the upper timberline. The wet periods detected in the years before the greater recruitment years could imply that optimal temperature conditions at greater altitude are not enough to promote a progress in the wider distribution of the species, but that the wetness level must be enough to optimize its development. The lack of synchrony between the recruitment and the radial growth of trees established at lower altitudes suggests an opposite climate impact in the species’ growth, which implies a possible long-term withdrawal of the altitude extent.
Keywords: Growth rings; climate change; Mexico’s central region; upper timberline; El Niño-Southern Oscillation (ENOS); Pinus hartwegii
El límite superior arbóreo de sitios elevados de México se espera que sea sensible al cambio climático. Por tal motivo se analizó el reclutamiento de Pinus hartwegii en el ecotono bosque-pastizal alpino de Monte Tláloc en el Estado de México, para detectar un posible avance del intervalo altitudinal de la especie a consecuencia del incremento en la temperatura. Para explorar la influencia del clima en el patrón de reclutamiento, se comparó la frecuencia de edades de P. hartwegii con relación a la temperatura y la precipitación; además, este impacto se analizó con la variación interanual en el grosor de anillos de crecimiento y su relación con los índices de El Niño-Oscilación del Sur (ENOS). La frecuencia de edades se fundamentó en un tamaño de muestra de 269 árboles, cuyos anillos de crecimiento se fecharon al año de su formación. El reclutamiento de P. hartwegii ha incrementado desde finales de la década de 1970, lo cual coincide con un aumento en la temperatura, en particular durante episodios El Niño. Esta situación se reflejó en un incremento radial inferior a la media en árboles ubicados por debajo del límite superior arbóreo. La detección de periodos húmedos en años previos a los de mayor reclutamiento podría implicar que no sólo bastan condiciones óptimas de temperatura a mayor altitud para promover un avance en la amplitud de la distribución de la especie, pero, además, el aporte de humedad debe ser suficiente para optimizar su desarrollo. La ausencia de sincronía entre el reclutamiento y el crecimiento radial de árboles establecidos a menor altitud, sugiere un efecto contrario del clima en el crecimiento de la especie, lo que implica un posible retraimiento de la amplitud altitudinal a largo plazo.
Palabras clave: Anillos de crecimiento; cambio climático; región centro de México; límite superior arbóreo; El Niño-Oscilación del Sur (ENOS); Pinus hartwegii
Introduction
Given the recent global climate changes (IPCC, 2007), a new behavior in the phenology, growth, and population dynamics of various tree species has been reported (Parmesan and Yohe, 2003; Parmesan, C. 2006). These ecological changes can be observed at their distribution limits, where the environmental conditions become extreme and where their consequences are likely to be more severe, as a result of a greater sensitivity of these species to global warming (Hampe and Petit, 2005; Kullman, 2005).
According to the tree areas affected by the increase of global temperature, an advance is expected in the upper limits of mountain areas and in the northern limit of their distribution, where low temperatures are no longer a limitation. Additionally, we can observe a recession in the lower mountain heights or in the southern limit of its distribution, where temperature conditions tend to be higher and droughts are more frequent and more intense (Matías, 2012).
In the upper timberline, specifically in transition areas like the Alpine tree line ecotone, evidence of a progress in the timberline as a result of the increase of temperature was found (Kullman, 1990; Körner and Paulsen, 2004; Körner, 2012), and one of the clearer answers is the recruitment of new seedlings and the increase of saplings (Juntunen and Neuvonen, 2006). Since recruitment is a key factor in the population dynamics control at the timberline (Rickebusch et al., 2007), the increase detected in the recruitment rates of some tree species, lengthways their altitude or latitude distribution limit, can indicate a progress in their distribution (Stöklin y Körner, 1999; Kullman, 2002; Harsch et al., 2009; Kullman, 2010).
The different climate scenarios models suggest increase of temperature and decrease in rainfall in the central region of México (Villers y Trejo, 2004; IPCC, 2007; Seager et al., 2009; Stahle et al., 2012; Stahle et al., 2016). These scenarios would impact the country’s high mountain forests, which are distributed above 3500 m, along the Transmexican volcanic belt, the dominion of Pinus hartwegii Lindl. (Arriaga and Gómez, 2004; Franco et al., 2006; Ricker et al., 2007; Endara et al., 2013).
Pinus hartwegii’s annual growth rings formation enables their use for climatic reconstructions and the analysis of drought or wet periods in Mexico’s central area, due to the relationship between radial growth and climate factors (Biondi, 2001; Biondi et al., 2009; Villanueva et al., 2015; Astudillo et al., 2016); therefore, this species is of particular importance for the study of climate change.
Extreme drought or rainfall events are related to superficial temperature anomalies in the tropical Pacific Ocean; which, in turn, are modulated by large-scale atmospheric and oceanic forces, such as El Niño-Southern Oscillation (ENSO). During its warm phase, ENSO causes extreme droughts in central and southern Mexico, while, during its cold phase, it has the opposite effect (Stahle et al., 1998; Méndez and Magaña, 2010; Stahle et al., 2012). Other studies of the same geographic area show that air temperature is likewise modified and that it increases during warm-phase ENOS events, and decreases during the cold phase (Enfield, 1996; Giannini et al., 2001; Karmalkar et al., 2011).
Since the high mountain forest’s upper timberline is temperature-sensitive, we must generate scientific data about the influence of this climate variable on the trees that they are made of (including evidence of its possible progress, as an answer to the increase of temperature). Therefore, the aim of this study was to assess the changes in P. hartwegii’s progress in the Alpine tree line ecotone, on the basis of the species’ recruitment pattern and it relationship with climate, through the use of meteorological records, growth rings series, and ENSO indexes. The hypothesis was that P. hartwegii’s increased recruitment beyond the timberline is linked with the recent increase of temperature in the region.
Materials and Methods
Field of study
The data for this study was obtained at Monte Tláloc, an elevation found in the Transmexican volcanic system; the mountain’s central part is located approximately at 19° 24’ 54” N and 98° 42’ 51” W, at an altitude of 4125 m. It is located in the State of Mexico and it borders the states of Puebla and Tlaxcala (Figure 1).

Figure 1 Geographic location of Monte Tlaloc in the Transmexican volcanic system. The image in the lower left corner indicates that the samples were taken above the timberline (4000 m).
Monte Tlaloc, where P. Hartwegii is the dominant element of the tree greenery, has a semi-cold weather with rainfalls during the summer (García, 2004). The climate records of the weather station closer to the site indicate an average temperature of 16.4 (maximum: 25.1; minimum: 7.7 °C), as well as an annual average rainfall of 621 mm (Figure 2).

Figure 2 Average monthly temperature and rainfall distribution registred at Chapingo’s weather station, Mexico (19.5° N, 98.9° W, 2250 m), 1955 to 2009 (CLICOM, 2015).
Andosol is the predominant type of soil in Monte Tlaloc, with appropriate quantity of humus for the development of temperate coniferous forests, currently composed of Abies religiosa (Kunth) Schltdl., and Cham., as well as P. hartwegii. However, at higher altitudes, P. hartwegii becomes the dominant species, forming pure stands above 3500 m (Endara, et al., 2012). The soil is shallow at this altitude and is classified as litosol; the herbaceous vegetation is made of bunchgrasses of the Calamagrostis and Festuca genera, which are typical of the Alpine grassland (Montero, 2002); whereas the bushy vegetation is made of scattered Juniperus monticola Mart individuals (Giménez and Escamilla, 1999; Villanueva et al., 2016).
Sampling and data compilation procedure
In order to obtain data from trees established in recent times and to determinate their age, we chose the Alpine tree line ecotone; this tree line is equivalent to the one defined by Körner and Paulsen (2004). In that mountain area, there was a recent invasion of P. Hartwegii, therefore, the sampling of young trees would allow us to analyze the climate influence on the species recruitment, on a yearly basis.
The sampling was carried out in October and November, 2013. The data was collected in the northern, eastern, southern and western hillsides. Two sampling lines were defined for each side, separated 100m from each other. Four 18-m diameter plots were established for each line above the timberline (4000 m) and they were distributed systematically in higher elevation sites; the centers of each plot were separated 200 m from each other (sampling points). The sampling points were taken from a previous design based on a satellite image of this area (Google Inc, 2013); these images were uploaded to a Global Positioning System (GPS) and they were located in the field; therefore, samples were collected from 32 plots, in a 3.2 ha area (Figure 1).
For each tree in the sampling plots, height and basal diameter were recorded. In order to determine their age, we carried out a subsampling of the dominant tree types; these subsamples were collected taking into consideration two (or more) growth cores (depending on whether or not we got the tree core). For trees with a 10cm+ basal diameter, the cores were collected as close as possible to the wood base, using a 5 mm Pressler drill (inner diameter); trees with <10cm diameter were cut with a manual saw, in order to obtain a transversal section, as close as possible to the soil surface.
The growth cores were mounted, sanded off and dated according to conventional dendrochronology techniques (Stokes and Smiley, 1968; Fritts, 1976). The samples were dated, using the exact year of their formation and the quality of the dating was verified using the COFECHA software (Holmes, 1983; Grissino-Mayer, 2001). In order to determine the date of their establishment, young samples that showed little change from year to year were used to carry out a ring-counting with a stereoscopic microscope. In order to improve our estimate of the year in which the trees were established, we carried out simple correlations, between the wood diameter versus its age, as well as its height versus age. Thus, 100 out of 269 sampled trees were dated (37 %) and, given that the relationship between diameter versus age was greater (r=0.80, p≤0.01) than the one obtained between height versus age (r=0.70, p≤0.01), the first correlation was used to generate a regression equation and estimate the age of non-dated trees. The age distribution of P. hartwegii considered all sampled trees in the four hillsides of Monte Tlaloc.
Climate influence on recruitment
Climate influence on recruitment was analyzed through the data climate records of Chapingo’s weather station (1955-2009). For that purpose, a mean, maximum, and minimum climate series temperature was generated, as well as a rainfall series. Additionally, P. hartwegii’s dendrochronology (1947-2012) was used; that data came from trees placed below the upper timberline in the area under study (Astudillo et al., 2016). The year-on-year multiannual changes in the growth index of the said chronology were used as reference to compare recruitment periods above the timberline.
Given that ENSO has a significant influence on the year-on-year and multiannual climate changes in Mexico (Magaña et al., 1999; Méndez and Magaña, 2010), we analyzed the Southern Oscillation Index (SOI) data which were obtained by NOAA, (2015) as an ENSO indicator. These indexes describe the difference between sea-level pressure between Tahiti and Darwin Island (Trenberth and Hoar, 1997). SOI negative (positive) value periods coincide with abnormally warm (or cold) waters along the western tropical Pacific Ocean, which is typical of El Niño and La Niña episodes (NOAA, 2014a, NOAA, 2014b).
Results and Discussion
Tree recruitment from 1947 to 1979 only included 7 % of the dated individuals. However, the greater recruitment happened from the late 1970s to 2012 (93 %), which implies a yearly 5% average recruitment rate for this period (Figure 3A).
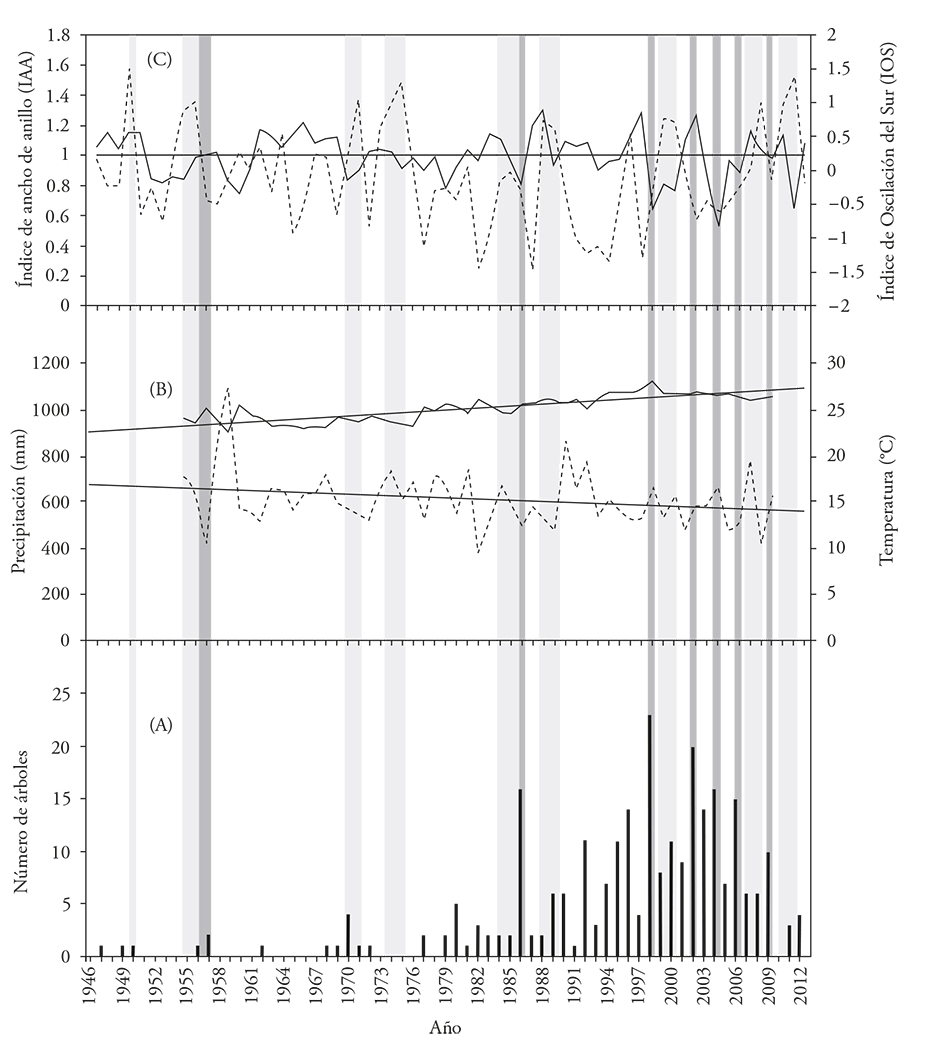
Figure 3 Comparison between tree recruitment, maximum annual temperature, overall ring series, and Southern Oscillation Index (SOI). (A) Annual P. hartwegii recruitment in the Alpine tree line ecotone. Dark grey bars show recruitment pulses; light grey lines indicate less recruitment. (B) Average rainfall (dotted line) and maximum temperature (continuous line) in Chapingo’s weather station (1995-2009). (C) Ring-width index (RWI) series for P. hartwegii (continuous line) and SOI (dotted line), for 1947-2012; horizontal grey lines show the average RWI; negative SOI values indicate El Niño episodes; positive values indicate La Niña episodes.
The climate records of Chapingo’s weather station indicate a 2 °C increase in maximum temperature and a 62 mm decrease in rainfall since 1955 (Figure 3B). Out of these two variables, temperature would have a greater influence on P. hartwegii recruitment, particularly, maximum temperature, which underwent a 3 °C increase (from 23 to 26 °C), from the late 1970s to 2009, reaching 28°C in 1998. These years coincide with the greatest recruitment of P. hartwegii individuals (Table 1).
Table 1 Correlation between mean, maximum, and minimum temperatures and rainfall versus the number of established trees (1955-2009)

ENOS’ influence generated greater P. hartwegii recruitment during severe El Niño episodes (as in 1957, 1986, 2004, 2006, and 2009), when the trees’ radial growth at lower altitudes was below average (Figure 3C). During La Niña episodes (as in 1950, 1955-1956, 1964, 1970-1971, 1974-1975, 1984-1985, 1988-1989, 1999-2000, 2007-2008, and 2010-2011), the number of surviving trees underwent a consistent decrease, except in 1970, when in most cases the trees’ radial growth at lower altitudes was above average (Figure 3C).
According to our hypothesis, an increase of temperature contributed to greater recruitment of P. hartwegii. This evidence is consistent with studies about the change in the global upper timberline and the tree invasion at higher altitudes, as temperature increases (Körner, 1998; Harsch et al., 2009; Kullman, 2010). In Mexico, low temperatures have restricted the colonization and growth of P. hartwegii above 4000 m in the main volcanic elevations of the country’s central region, according to Beaman (1962) and Lauer (1978). Therefore, successful recruitment above this altitude limit in Monte Tlaloc will likely point to a change towards higher temperatures in this area.
Although rainfall did not have a significant influence in years of greater recruitment, the presence of humidity in previous years (La Niña) could be significant for the abundance of recruited trees the following year, once humidity had been stored in the soil and temperature were optimum (Kullman, 2002; Harsch, 2009). An exception to this reasoning was the years before 1998 and 2004, when no La Niña episodes were recorded. Nevertheless, during those years, besides high temperatures, there were also rains in Monte Tlaloc that exceeded the historical summer average.
Fires, particularly surface or low-intensity fires, are a favorable factor to the recruitment of P. hartwegii’s seedling, as they eliminate physical competition (pastures) and ash provides more nutrients to the soil (Rodríguez et al., 2004.); however this benefit would only be possible if additional humidity was available. In our study, we found out that the most important recruitment pulse took place in 1998, the year in which more forest fires were recorded in central Mexico (Rodríguez and Pyne, 1999; Duncan et al., 2003), even in extremely high mountains, such as Pico de Orizaba, where forest fires were documented in 1998, based on fire marks in P. hartwegii trees (Yocom and Fulé, 2012). It is likely that such fires happened in Monte Tlaloc during the spring (March-May) of 1998, a period that was simultaneous with ENSO’s heat episodes, but not during the summer of the same year, when the conditions were the opposite (La Niña) (NOAA, 2015). Therefore, abundant rain, after a fire in the zone under study, is likely to have favored the establishment of seedlings.
The same principle, that previous fires, which probably happened during the intense droughts recorded in the country’s central region in 1953 and 1982 (Florescano, 1980; Metcalfe, 1987; Duncan et al., 2003), were followed by wet years and subsequent recruitment, could be applied to the 1957 and 1986 pulses. Previous local fires are unlikely to be the cause of the 2002, 2004, 2006, and 2009 recruitment pulses, because there are no records of conditions that favored those events; if there had been fires above the timberline during those years, established seedlings would have succumbed to the said disaster, because they are more susceptible to fire during this development stage (Velázquez et al., 1986; Vélez, 2009).
Results provide additional evidence about tree recruitment in the ecotone under study: they allowed us to determine that recruitment is more successful when higher temperature and soil humidity conditions are combined. Therefore, this finding suggests that optimum temperature conditions are not enough at higher elevations, but that enough humidity stored in the soil’s profile is crucial to promote tree growth, before the growth season starts.
Since an increase of temperature is directly linked with greater evapotranspiration, combining these stress factors results in low photosynthetic productivity (Marino C., 2002), which has a negative effect in trees’ growth and survival (Camarero and Gutiérrez, 2004; McDowell et al., 2010). Besides, poor and highly permeable soils, such as the ones that thrive in Monte Tlaloc’s Alpine tree line ecotone, tend to dry fast and this has a similar impact on younger trees (Holtmeier et al., 2003; Holtmeier and Broll, 2005). In this way, water stress is expected to be harsher and fire incidents to increase in the ecotone under study. Frequent fires in P. hartwegii’s upper timberline diminishes its population (Miranda and Hernández, 1985; Rodríguez et al., 2004.), therefore inhibiting their colonization above 4000 meters, either as a result of the absence of germplasm or the direct elimination of already established seedlings.
The lack of consistency between tree recruitment and the presence of rainfall implies that, although La Niña episodes favor increased rainfalls in Mexico’s central region (Magaña et al., 2003), extremely low temperatures were dominant, even until late spring (Karmalkar et al., 2011; NOAA, 2014b). Therefore, this would mean that a high likelihood of intense frost and snowfall in Monte Tlaloc during these episodes would be the cause of the seedlings’ mortality, as can be inferred from the scarce establishment of trees during those years.
Young trees’ mortality at high altitudes, likely influenced by intense frosts and snowfall until late spring, limits the growth period (Hofgaard et al., 2009), but it also has a negative repercussion on the plant’s development, as it harms its tissues (Holtmeier, 2009; Körner, 2012). Additionally, trees in their early development stages are more susceptible to fungal infections during these episodes (Stöklin y Körner, 1999), resulting in a limited population density.
The comparison between P. hartwegii’s recruitment and the ring width rate of the same species, established under the timberline, suggests that young trees in the Alpine tree line ecotone do not respond in the same way to an increase of temperature, compared with mature trees at a lower altitude. This lack of synchrony might point out that increases of temperature -which go along with diminished rainfall in Monte Tlaloc- has a negative impact in P. hartwegii’s radial growth at lower heights. This situation is consistent with data for other forest species in the upper timberline (Barber et al., 2000; Lloyd and Fastie, 2002; D’ Arrigo et al., 2004; Jolly et al., 2005; Sidor et al., 2015).
Diminished precipitation can lead to a progressive reduction of the radial growth (Bogino et al., 2009; Sánchez et al., 2012); additionally, several years of drought diminish the woodland’s recovery capacity after an extreme episode (Martínez V., 2012). This overall weakening of the individuals can result in a greater sensitivity and death of the woodlands, if they are subject to pests and fire, which are also increased by higher temperatures (Villers y Trejo, 1998; Bigler et al., 2006; PROBOSQUE. 2010.).
The increasingly frequent high temperatures and droughts in Monte Tlaloc can affect the establishment of new individuals under the timberline, since the seedling and juvenile phases are more sensitive to the lack of humidity in the trees’ ontogenetic cycle. Therefore, the mortality rate in these forest areas will likely increase and even reach a recruitment shortage during the less rainy summers (Castro et al., 2004; Mendoza et al., 2009), resulting in the presence of aged populations. On the long run, its population density may decrease and favor a contraction in the lower altitude interval for the species’ distribution.
Conclusions
P. hartwegii’s recruitment trend in Monte Tlaloc’s Alpine tree line ecotone was made by possible by the combination of an increased yearly maximum temperature and the presence of humidity, detected in the climate records of the past 66 years, and became clear since of the late 1970s to 2009. The greatest recruitment of P. hartwegii occurred during ENOS’ warm phase; additionally, good humidity conditions during La Niña years (or a year before that) contributed to its establishment.
There is a trend in the increase of temperature, which results in the forest reaching greater mountain heights. However, this process is not guaranteed, due to a trend of diminished rainfall, particularly, when new seedlings lack an extensive root system that allows them to cover a great soil volume and meet their water requirement.
If these climate conditions continue, the populations of this species will likely contract in the forest’s lower heights, as we were able to verify in the root growths under observation
Literatura Citada
Arriaga, L., y L. Gómez. 2004. Posibles efectos del cambio climático en algunos componentes de la biodiversidad de México. In: Martínez, J., y A. Fernández-Bremauntz. (comps). Cambio Climático: Una Visión desde México. INE/SEMARNAT. México, pp: 255-265. [ Links ]
Astudillo S. et al. 2016. Climatic variability at the treeline of Monte Tlaloc, Mexico: a dendrochronological approach. Trees. DOI 10.1007/s00468-016-1460-z. [ Links ]
Barber, V., G. P. Juday, and B. Finney. 2000. Reduced growth of Alaska white spruce in the twentieth century from temperature-induced drought stress. Nature 405: 668-672. [ Links ]
Beaman, J. H. 1962. The timberlines of Iztaccihuatl and Popocatepetl, Mexico. Ecol. 43: 377-385. [ Links ]
Bigler, C. et al. 2006. Drought as an inciting mortality factor in scots pine stands of the Valais, Switzerland. Ecosystems 9: 330-343. [ Links ]
Biondi, F. 2001. A 400-year tree-ring chronology from the tropical treeline of North America. Ambio 30: 162-166. [ Links ]
Biondi, F., P. Hartsough, and I. G. Galindo E. 2009. Recent warming at the tropical treeline of North America. Front. Ecol. Environ. 7: 463-464. [ Links ]
Bogino, S., M. J. Fernández-Nieto , and F. Bravo. 2009. Climate effect on radial growth of Pinus sylvestris at its southern and western distribution limits. Silva Fenn. 43: 609-623. [ Links ]
Camarero, J. J., and M. Gutiérrez. 2004. Pace and pattern of recent treeline dynamics: response of ecotones to climatic variability in the Spanish Pyrenees. Climate Change 63: 181-200. [ Links ]
Castro, J., R. Zamora, J. A. Hódar, and J. M. Gómez. 2004. Seedling establishment of a boreal tree species (Pinus sylvestris) at its southernmost distribution limit: consequences of being in a marginal Mediterranean habitat. J. Ecol. 92: 266-277. [ Links ]
CLICOM (Clima Computarizado). 2015. Base de datos climáticos diarios. http://clicom-mex.cicese.mx . (Consulta: Octubre 2015). [ Links ]
D’Arrigo, R. D. et al. 2004. Thresholds for warming induced growth decline at elevational tree line in the Yukon Territory Canada. Glob. Biogeochem. Cycle 18: GB3021. [ Links ]
Duncan, B. N. et al. 2003. Interannual and seasonal variability of biomass burning emissions constrained by satellite observations. J. Geophys. Res. 2: 1-13. [ Links ]
Endara A. et al. 2012. Por los senderos de Tláloc: el bosque y la montaña. In: Gobierno del Estado de México y Universidad Autónoma del Estado de México. Monte Tláloc II, la casa del Dios del Agua. Dirección de Difusión y Promoción de la Investigación y los Estudios Avanzados (SIEA), UAEM. México. pp: 89-108. [ Links ]
Endara A. et al. 2013. Analysis of fragmentation processes in high mountain forest of the centre of Mexico. Am. J. Plant Sci. 4: 697-704. [ Links ]
Enfield, D. B. 1996. Relationships of inter-American rainfall to tropical Atlantic and Pacific SST variability. Geophys. Res Lett .. 23: 3305-3308. [ Links ]
Florescano, E. (ed). 1980. Análisis Histórico de las Sequías en México. Secretaría de Agricultura y Recursos Hidráulicos, Comisión del Plan Nacional Hidráulico. México, D.F. 158 p. [ Links ]
Franco, S., H. H. Regil, y J. A. Ordoñez. 2006. Dinámica de perturbación-recuperación de las zonas forestales en el Parque Nacional Nevado de Toluca. Madera y Bosques 12: 17-28. [ Links ]
Fritts, H. C. 1976. Tree Rings and Climate. London Academic Press. New York, NY, USA. 565 p. [ Links ]
García, E. 2004. Modificaciones al sistema de clasificación climática de Köppen, para adaptarlo a las condiciones de la República Mexicana. 5a. ed. Instituto de Geografía, Universidad Nacional Autónoma de México. México. 90 p. [ Links ]
Giannini A., Y. Kushnir, and M. A. Cane. 2001. Seasonality in the impact of ENSO and the North Atlantic High on Caribbean rainfall. Phys. Chem. Earth Part B 26:143-147. [ Links ]
Giménez de Azcárate, J., y M. Escamilla. 1999. Las comunidades edafoxerófilas (enebrales y zacatonales) en las montañas del centro de México. Phytocoenologia 29: 449-468. [ Links ]
Grissino-Mayer, H. D. 2001. Evaluating crossdating, accuracy: a manual and tutorial for the computer program COFECHA. Tree-Ring Res. 57: 205-221. [ Links ]
Hampe, A., R. J. Petit. 2005. Conserving biodiversity under climatic change: the rear edge matters. Ecol Lett. . 8: 461-467. [ Links ]
Harsch, M. A. et al. 2009. Are treelines advancing? A global meta-analysis of treeline response to climate warming. Ecol Lett. . 12: 1040-1049. [ Links ]
Hofgaard, A., L. Dalen, and H. Hytteborn. 2009. Tree recruitment above the tree line and potential for climate-driven tree line change. J. Veg. Sci. 20: 1133-1144. [ Links ]
Holmes, R. L. 1983. Computer-assisted quality control in tree-ring dating and measurement. Tree-Ring Bull. 43:69-78. [ Links ]
Holtmeier, F. K. 2009. Mountain Timberlines. Ecology, Patchiness and Dynamics. Advances in Global Change Research 36. 2nd. ed. Springer. USA. 448 p. [ Links ]
Holtmeier, F. K., G. Broll. 2005. Sensitivity and response of northern hemisphere altitudinal and polar treelines to environmental change at landscape and local scales. Global Ecol . Biogeogr. 14: 395-410. [ Links ]
Holtmeier, F. K ., G. Broll, K. Anschlag, A. Müterthies. 2003. Regeneration of trees in the treeline ecotone: Northern Finnish Lapland. Fennia 1981: 103-128. [ Links ]
IPCC (Intergovernmental Panel on Climate Change). 2007. Climate Change, in 2007. The Physical Science Basis. Contribution of Working Group I to the Fourth Assessment Report of the Intergovernmental Panel on Climate Change. Cambridge University Press, Cambridge, United Kingdom and New York, NY, USA. 996 p. [ Links ]
Jolly, W. M., M. Dobbertin, N. E. Zimmermann, M. Reichstein. 2005. Divergent vegetation growth response to the 2003 heat wave in the Swiss Alps. Geophys. Res Lett.. 32: L18409. [ Links ]
Juntunen, V., S. Neuvonen. 2006. Natural regeneration of Scots pine and Norway spruce close to the timberline in northern Finland. Silva Fenn . 40: 443-458. [ Links ]
Karmalkar, A.V., R. S. Bradley, H. F. Diaz. 2011. Climate change in Central America and Mexico: Regional climate model validation and climate change projections. Clim. Dynam. 37: 605-629. [ Links ]
Körner, C. 1998. A re-assessment of high elevation treeline positions and their explanation. Oecologia 115: 445-459. [ Links ]
Körner, C. 2012. Alpine Treelines: Functional Ecology of the Global High Elevation Tree Limits. Springer, Basel. Heidelberg New York Dordrecht London. 220 p. [ Links ]
Körner, C., J. Paulsen. 2004. A world-wide study of high altitude treeline temperatures. J. Biogeogr. 31: 713-732. [ Links ]
Kullman, L. 1990. Dynamics of altitudinal tree-limits in Sweden: a review. Norsk Geogr. Tidsskrift 44: 103-116. [ Links ]
Kullman, L. 2002. Rapid recent-margin rise of tree and shrub species in the Swedish Scandes. J. Ecol . 90: 68-77. [ Links ]
Kullman, L. 2005. Pine (Pinus sylvestris) treeline dynamics during the past millennium- a population study in west-central Sweden. Ann. Botanici Fennici 42: 95-106. [ Links ]
Kullman, L. 2010. A richer, greener and smaller Alpine World: Review and projection of warming-induced plant cover change in the Swedish Scandes. Ambio 39: 159-169. [ Links ]
Lauer, W. 1978. Timberline studies in Central Mexico. Arct. Alp. Res. 10: 383-396. [ Links ]
Lloyd, A. H., C. L. Fastie. 2002. Spatial and temporal variability in the growth and climate response of treeline trees in Alaska. Climatic Change 52: 481-509. [ Links ]
Magaña, V. O., J. L. Vázquez, J. L. Pérez, J. B. Pérez. 2003. Impact of El Niño on precipitation in Mexico. Geofísica Int. 42: 313-330. [ Links ]
Magaña, V. et al. 1999. El Niño y el clima. In: Magaña, V. (ed). Los Impactos del Niño en México. SEP-CONACYT. México, pp: 23-68. [ Links ]
Marino C., H. 2002. Respuestas ecofisiológicas de plantas en ecosistemas de zonas con clima mediterráneo y ambientes de altamontaña. Rev. Chil. Hist. Nat. 75: 625-637. [ Links ]
Martínez V., J. et al. 2012. Stand and tree-level determinants of the drought response of Scots pine radial growth. Oecologia 168: 877-888. [ Links ]
Matías, L. 2012. Cambios en los límites de distribución de especies arbóreas como consecuencia de las variaciones climáticas. Ecosistemas 21: 91-96. [ Links ]
McDowell, N. G., C. D. Allen, L. Marshall. 2010. Growth, carbon-isotope discrimination, and drought-associated mortality across a Pinus ponderosa elevational transect. Glob. Change Biol. 16: 399-415. [ Links ]
Méndez, M., V. Magaña. 2010. Regional aspects of prolonged meteorological droughts over Mexico and Central America. J. Climate 23: 1175-1188. [ Links ]
Mendoza, I. et al. 2009. Recruitment limitation of forest communities in a degraded Mediterranean landscape. J. Veg. Sci . 20: 367-376. [ Links ]
Metcalfe, S.E. 1987. Historical data and climatic change in Mexico-a review. Geogr. J. 2: 211-222. [ Links ]
Miranda, F., E. Hernández-Xolocotzi. 1985. Los tipos de vegetación en México y su clasificación. En: Xolocotzia Tomo I. Reproducido del boletín de la Soc. Botánica de México No. 28. Revista de Geografía Agrícola. Universidad Autónoma Chapingo. pp. 41-162 [ Links ]
Montero, I. 2002. Atlas Arqueológico de la Alta Montaña Mexicana. SEMARNAT-CONAFOR. México. 180 p. [ Links ]
NOAA (National Oceanic and Atmospheric Administration), 2015. Southern Oscillation Index (SOI). http://www.cpc.ncep.noaa.gov/data/indices/soi . (Consulta: Octubre 2015). [ Links ]
NOAA (National Oceanic and Atmospheric Administration). 2014a. Climate Variability: Southern Oscillation Index. http://www.climate.gov/news-features/understanding-climate/climate-variability-southern-oscillation-index . (Consulta: Julio 2014). [ Links ]
NOAA (National Oceanic and Atmospheric Administration). 2014b. El Niño/Southern Oscillation (ENSO). Episodios históricos ELNINO/LA NINA (1950 al presente). Changes in the Oceanic Niño Index (ONI). In: Center for weather and climate prediction. http://www.cpc.ncep.noaa.gov/products/analysis_monitoring/ensostuff/ensoyears.shtml . (Consulta: Julio 2014). [ Links ]
Parmesan, C. 2006. Ecological and evolutionary responses to recent climate change. Annu. Rev. Ecol. Evol. S. 37: 637-669. [ Links ]
Parmesan, C., G. Yohe. 2003. A globally coherent fingerprint of climate change impacts across natural systems. Nature 421: 37-42. [ Links ]
PROBOSQUE (Protectora de Bosques). 2010. Inventario Forestal del Estado de México. México. 222 p. [ Links ]
Rickebusch, S. et al. 2007. Understanding the low-temperature limitations to forest growth through calibration of a forest dynamics model with tree-ring data. Forest Ecol. Manag. 246: 251-263. [ Links ]
Ricker, M., G. Gutiérrez G., D. C. Daly. 2007. Modeling long-term tree growth curves in response to warming climate: test cases from a subtropical mountain forest and a tropical rainforest in Mexico. Can. J. Forest Res. 37: 977-989. [ Links ]
Rodríguez T., D. A., H. C.Martínez H., V. Ortega B. 2004. Ecología del fuego en bosques de Pinus hartwegii. In: Villers-Ruiz, L., y J. López-Blanco (eds). Incendios forestales en México. Métodos de Evaluación. Centro de Ciencias de la Atmósfera, Universidad Nacional Autónoma de México. México. pp: 103-120. [ Links ]
Rodríguez T., D. A ., and S. J. Pyne. 1999. Mexican fires of 1998. Int. For. Fires News 20: 61-63. [ Links ]
Sánchez S. et al. 2012. Is drought the main decline factor at the rear edge of europe? The case of southern Iberian pine plantations. Forest Ecol. Manag. 271: 158-169. [ Links ]
Seager, R. et al. 2009. Mexican drought: an observational modeling and tree ring study of variability and climate change. Atmosfera 22: 1-31. [ Links ]
Sidor, C. G., I. Popa, R. Vlad, P. Cherubini. 2015. Different tree-ring responses of Norway spruce to air temperature across an altitudinal gradient in the Eastern Carpathians (Romania). Trees 29: 985-997. [ Links ]
Stahle, D. W. et al. 2016. The Mexican Drought Atlas: Tree-ring reconstructions of the soil moisture balance during the late pre-Hispanic, colonial, and modern eras. Quat. Sci. Rev. 149: 34-60. [ Links ]
Stahle, D. W. et al. 2012. Pacific and Atlantic influences in Mesoamerican over the past millennium. Clim. Dynam . 39: 1431-1446. [ Links ]
Stahle, D. W. et al. 1998. Experimental dendroclimatic reconstruction of the Southern Oscillation. Bull. Am. Meteorol. Soc. 79: 2137-2152. [ Links ]
Stokes, M. A., T. L. Smiley, 1968. An Introduction to Tree-Ring Dating. University of Chicago Press. Chicago, USA. 73 p. [ Links ]
Stöklin, J., C. Körner. 1999. Recruitment and mortality of Pinus sylvestris near the arctic tree line: the role of climatic change and herbivory. Ecol. Bull. 47: 168-177. [ Links ]
Trenberth, K. E., T. J. Hoar.1997 El Niño and climate change. Geophys. Res. Lett. 24: 3057-3060. [ Links ]
Velázquez M. A., Musálem M. A., Keyes M. R., L. G. Zárate. 1986. Influencia del tratamiento en el suelo y la condición de apertura del dosel en el establecimiento inicial de la regeneración natural de Pinus hartwegii Lindl. Agrociencia 64: 147-170. [ Links ]
Vélez, R. 2009. La Defensa Contra los Incendios Forestales, Fundamentos y Experiencias. 2a. ed. McGraw Hill. España. 840 p. [ Links ]
Villanueva D., J. et al. 2016. Potencial dendrocronológico de Juniperus monticola Martínez en el Monte Tláloc, México. Rev. Fitotec. Mex. 39: 175-185. [ Links ]
Villanueva D., J. et al. 2015. Red dendrocronológica del pino de altura (Pinus hartwegii Lindl.) para estudios dendroclimáticos en el noreste y centro de México. Invest. Geogr. Bull. Inst. Geogr. UNAM 86: 5-14. [ Links ]
Villers R., L., I. Trejo V. 1998. Climate change on Mexican forest and natural protected areas. Glob. Environ. Change 8: 141-157. [ Links ]
Villers R., L., I. Trejo V. 2004. Evaluación de la vulnerabilidad en los ecosistemas forestales. In: Martínez, J, A. Fernández-Bremauntz. (comps). Cambio Climático: Una Visión desde México. INE/SEMARNAT. México, D.F. pp: 239-254. [ Links ]
Yocom, L. L. and P. Z. Fulé. 2012. Human and climate influences on frequent fire in a high-elevation tropical forest. J. Appl. Ecol . 49: 1356-1364. [ Links ]
Received: April 2016; Accepted: November 2016