Introduction
International perspectives on California Brown Pelican conservation: A background
Endangered species and other listing processes in the United States and Mexico
In the United States (US) the Brown Pelican species (Pelecanus occidentalis) was officially classified “endangered” under the Endangered Species Act (ESA) of 1973 (Gress and Anderson 1983). Pollution-caused reproductive failures in the mid-1960s and early 1970s (Keith et al. 1971, Risebrough et al. 1971, Risebrough 1972, Jehl 1973, Anderson and Gress 1981, Gress and Anderson 1983, Gress 1995, and references therein) resulted in the federal and state of California endangered species status in 1970 and 1971 (Leach and Fisk 1972, California Fish and Game Commission 1981). This classification applied to all subpopulations within the range of the subspecies, the California Brown Pelican ( P. o. californicus) (hereafter simply referred to as “pelican”) (Table 1) . Listing was based entirely on the status of pelicans in the Southern California Bight (SCB) subpopulation (Fig. 1a), and it put less emphasis on the much larger breeding numbers in the Gulf of California and elsewhere in Mexico.
Table 1 A brief listing of milestones related to the conservation of the California Brown Pelican (CABRPE) in the United States and Mexico. USFWS = US Fish and Wildlife Service; CDFW = California Department of Fish and Wildlife; CONANP = National Commission for Protected Natural Areas, Mexico; BRPE = Brown Pelican.
Date(s) | Event(s) | Source |
1936 | Mexico joins the Migratory Bird Treaty Act with the United States and begins participation in protecting North American migratory birds. | Wikipedia.org |
1962 | Earliest documented evidence of DDE-related reproductive effects on CABRPE off southern California. | Anderson and Hickey (1972) |
1964 | The Mexican government declares Rasa Island as the first Gulf of California, Mexico, sanctuary, not for CABRPE, but this sets the stage for future actions in the Gulf of California, relating directly to all migratory seabirds. | Ezcurra et al. (2002), Ezcurra (2014) |
1969 | Scientists first publish data on a severe population decline of CABRPE and its pollution-related causes in the Southern California Bight, USA. | Schreiber and Risebrough (1972), Jehl (1973) |
1969-1971 | Endangered status is designated by the USFWS and CDFW. | See text |
1971 | Anacapa Island Research Natural Area is established by the US National Park Service, indirectly further protecting CABRPE in the United States. | Gress (2009) |
1972 | The Pacific Seabird Group formulates a policy statement supporting conservation of the Gulf of California, Mexico. | Ainley et al. (1972) |
1973 | The contamination problem in southern California is demonstrated to extend south along the Pacific coast into northwestern Baja California, the effects declining to the south and in the Gulf of California. | Keith et al. (1971), Jehl (1973) |
1971-1975 | Regulatory actions are taken by various agencies related to contamination abatement and management, resulting in the beginnings of a long-term CABRPE recovery off California. | Anderson et al. (1975) |
1976 | Resources (emphasizing the CABRPE and other seabirds) of the Gulf of California are recommended for conservation action. | Anderson et al. (1976) |
1978 | The Mexican government establishes Gulf of California islands as a Migratory Bird and Wildlife Nature Reserve and Refuge (Zona de Reserva y Refugio de Aves Migratorias y de la Fauna Silvestre). | Ezcurra et al. (2002) |
1979 | Anacapa Island Ecological Reserve is established by the CDFW and US National Park Service. | Gress (2009) |
1980 | The first scientifically-published statement to delist the CABRPE is proposed. | Schreiber (1980) |
1980 | The Channel Islands Marine Sanctuary is established by the National Oceanic and Atmospheric Administration, indirectly benefiting CABRPE. | Gress (2009) |
1982 | The Pacific Seabird Group establishes a CABRPE Advisory Committee. | LL Leschner (pers. comm.) |
1983 | The California Brown Pelican Recovery Plan is approved by the USFWS. | Gress and Anderson (1983) |
1986 | The Pacific Seabird Group recommends the down-listing of CABRPE and additionally concurs with the USFWS delisting of the Florida brown pelican, Pelecanus occidentalis carolinensis. | Leschner (1986) |
1990s | The USFWS and CDFW continue to review the conservation status of CABRPE; monitoring studies continue. | See text |
2000 | The Mexican government adjusts the category of Gulf of California islands (the “hub” of the subspecies’ range) as a Flora and Fauna Protection Area (Área de Protección de Flora y Fauna, Islas del Golfo de California), directly protecting a large proportion of the subspecies. | Ezcurra et al. (2002), Godínez-Reyes et al. (2006) |
2005 | Decree for the San Lorenzo Archipelago as a national park. | DOF (2005) |
2005 | The Pacific Seabird Group issues an updated, renewed support and additional recommendations for seabird conservation in the Gulf of California. | Anderson et al. (2005) |
2006 | The Endangered Species Recovery Council submits proposal to the USFWS and California Department of Fish and Game to delist the California subspecies; USFWS responds. | See text |
2006 | CONANP conservationists in Mexico assign the CABRPE as an indicator species for long-term population and ecological health monitoring. | Godínez-Reyes et al. (2006) |
2007 | Decree for the Bahía de los Ángeles Biosphere Reserve | DOF (2007) |
2006-2009 | The USFWS and CDFW complete extensive reviews on the statuses of the BRPE throughout its range. | See text |
2009 | BRPE is delisted in the United States and a post-delisting monitoring plan is proposed by USFWS, but not initiated. | See text |
2009-2015 | Monitoring for population status in the Southern California Bight (region of original endangerment) is completely lacking or minimal; monitoring in the Gulf of California continues on an annual basis. Possible signs of new population decline emerge in the Southern California Bight. | See text |
2010 | The Mexican government enlists the CABRPE for special protection status under NOM-059-SEMARNAT-2010. | DOF (2010) |
2014 | Gulf of California Midriff Island management plans and initial resource inventory published. | CONANP (2014a, 2014b) |
2014-2016 | The “Blob” (a Pacific-wide warming) depresses CABRPE reproduction range-wide. | This study |
2016-2020 | The USFWS funds post-delisting monitoring for Anacapa Island, California; monitoring begins. | R McMorran (pers. comm.) |

Figure 1 (a) Total California Brown Pelican subspecies (Pelecanus occidentalis californicus) breeding range with subpopulation designations (based on ecological and distributional differences) (from Anderson et al. 2013): SCB = Southern California Bight, SBP = Southern Baja-Pacific, GOC = Gulf of California, MME = Mexican Mainland Estuarine, and MMI = Mexican Mainland Island. The percentages following each designation represent proportions of the total subspecies breeding in that range in 2006. Red, dashed lines represent approximate boundaries of subpopulations. (b) The mid-region of the GOC showing northern (N) breeding colonies in our study area (CHL = Cholluda Island, SLU = San Luis Island, and PRF = Puerto Refugio); southern (S) breeding colonies (PIO = Piojo Island and SLA = San Lorenzo Archipelago); and 4 additional monitored breeding colonies south of our sampling zone (ALC = Alcatraz Island, SPM = San Pedro Mártir Island, SPN = San Pedro Nolasco Island, and CHA = Chaperona Island).
With cessation of oceanic input of the insecticide DDT (4,4’-dichloro-diphenyl-trichloro-ethane), originating from a sewage outfall and a manufacturing plant (Schmidt et al. 1971 and others), in about 1970/1971, and concomitant with reduction of DDT’s more recalcitrant metabolite, DDE (4,4’-dichloro-diphenyl-trichloro-ethylene) (Anderson et al. 1975, 1977; Gress 1995), the SCB subpopulation began to slowly recover. Breeding numbers (expressed as number of nests attempted [NA]) had apparently recovered significantly by the mid-1980s (Gress et al. 2003), peaking in 2004 with a record number of nearly 8,000 NA on Anacapa Island, California, alone (Fig. 1a) (Gress 2009). Pelecanus o. californicus, along with the remainder of the Brown Pelican total species, after extensive review and preceded by a long delay (Table 1) (see also recent reviews of the recovery by USFWS 2008 and Burkett et al. 2007), was finally removed from the federal and California endangered species lists in 2009 (Burkett et al. 2007, USFWS 2009a) (Table 1). Delisting was also based partly on a range-wide survey in 2006 by Anderson et al. (2013).
The P. o. californicus metapopulation (= subspecies) has, however, always been dominated by numbers breeding in the Gulf of California (hereafter referred to as “Gulf” or GOC), and it continues to be. In 2006, breeding numbers of pelicans from the GOC and MME (Mexican Mainland Estuarine) subpopulations (Fig. 1a) comprised 76.4% of the entire subspecies. Numbers nesting on various local islands in 5 island-clusters in the Gulf (Fig. 1b), in an area commonly termed the “Midriff Region”, alone comprised 67.4% of the Gulf subpopulation and 41.4% of the entire subspecies in 2006 (Anderson et al. 2013). The SCB subpopulation, at its highest ever recorded in 2006, still comprised only 16.6% of the metapopulation after recovery from declines in the SCB. In longer terms, this percentage was likely even less historically (more like ~5-10%). The California Brown Pelican Recovery Plan empirically considered that a 5-year mean productivity value (expressed as young fledged per nest attempt, YY • NA-1) of ~0.9, and ~3,000 NA • yr-1 (~4.2% of the metapopulation) would be adequate criteria for delisting in the US (Gress and Anderson 1983).
In Mexico, as early as 1964, indirectly relating to the pelican and other seabirds, a pioneering program for migratory and non-migratory marine bird conservation essentially set Gulf seabird conservation into its formal beginnings with the establishment of Rasa Island as a Nature Reserve and Refuge for Migratory Birds (Table 1; Velarde and Anderson 1994, Ezcurra et al. 2002, Ezcurra 2014 [these authors provide a detailed overview of these and other actions that have led to today’s ecological conservation in the Gulf]).
On the Baja California west coast and in the Gulf, where the subspecies’ range is and has been by far dominant based on breeding population size (Anderson and Gress 1981; Gress and Anderson 1983; Anderson et al. 2006, 2013), P. o. californicus was also given more formal protection by the Mexican government as early as 1978 (DOF 1978, Carabias-Lillo et al. 2000). The pelican has recently also been included in the Mexican Environmental Protection Official Norm (DOF 2010) and also designated as one of several sentinel species used to help monitor the Gulf (Godínez-Reyes et al. 2006) (http://www.islasgc.conanp.gob.mx/islas/) (Table 1, Fig. 1b). In the Gulf of California Islands Management Plan, long-term population and health/contaminant monitoring, along with island and seabird colony conservation (centered on marine ecosystems and islands rather than individual species) have been the primary goals under the philosophy of “ecosystem health” and “ecological conservation”, rather than individual species management and conservation (Godínez-Reyes et al. 2006, Ezcurra 2014). This specific plan recognizes the importance of the marine ecosystem around the Midriff Islands for all marine and insular biota, including marine birds. It includes the marine areas around Rasa Island, and the main islands plus islets around the San Lorenzo Archipelago, where the largest colony of P. o. californicus is found in the San Lorenzo Archipelago National Park (DOF 2005). In 2007 the Bahía de los Ángeles Biosphere Reserve was established (DOF 2007), including 387,986 ha in the Midriff Island area, and the original management plan was published in 2010. This all resulted in an impressive, Gulf island- and marine-conservation effort (Carabias-Lillo et al. 2000; CONANP 2014a, 2014b) and this protection system strongly applies to, and directly affects, the entire California Brown Pelican subspecies.
The long delisting process in the US
In the 4 decades ensuing since the first US ESA listing of pelicans in 1970, the entire California subspecies has undergone extensive study, review, and evaluation in both the US and Mexico (e.g., Anderson et al. 1976; Anderson and Gress 1981, 1983; Gress and Anderson 1983; Shields 2002; Gress et al. 2003; Capitolo et al. 2012). Census data from a range-wide survey in 2006 (Anderson et al. 2013), including the US and Mexico, indicated little change in numbers of breeding pelicans in most of the range over about 4 decades of nearly continuous study, except in the SCB breeding sub-population (Fig. 1a), which had shown breeding population recoveries until about 2010 (Gress 1995, 2009; Stahl et al. 2015). Major SCB pelican nesting colonies include those at Anacapa Island and Santa Barbara Island, California, plus the Coronados Islands off the northwestern coast of Baja California, Mexico (Anderson and Gress 1983). Also, several islands with more ephemeral or historical colonies remained likely recolonization sites: Scorpion Rock, Prince Island, and San Clemente Island in southern California, and Todos Santos Islands and San Martín Island in Baja California (Gress 1970). Recent colonizations of some of these islands by breeding pelicans (e.g., Aguirre-Muñoz et al. 2015, Stahl et al. 2015) had supported the notion of breeding population recovery in the SCB. For example, Palacios et al. (2003) reported in the early 2000s, the first known nesting of pelicans at Todos Santos Islands since 1920, and the first nesting at San Martín Island since 1978.
However, before 2009, US delisting had been temporarily postponed (Table 1) until there were some assurances that the much larger, continuouslyviable segment of the overall pelican metapopulation to the south also received viable conservation programs in Mexican waters (Gress and Anderson 1983). Indeed, conservation agencies and NGOs have made significant seabird conservation strides in western Mexico (Carabias-Lillo et al. 2000; Danemann and Ezcurra 2008; CONANP 2014a, 2014b; Ezcurra 2014; and others). A formal petition to reclassify or delist from the US perspective was finally put forward in 2006 by the US Fish and Wildlife Service (USFWS 2006). Total conservation of the pelican and other seabirds in both countries has always been only loosely connected in practice, however.
After the beginnings of this apparent local breeding population recovery in waters off southern California and north-western Baja California (historical review of population status and causes of endangerment by Gress 1970, 1995; Keith et al. 1971; Jehl 1973; Anderson et al. 1975; Anderson and Gress 1981; Gress and Anderson 1983; and others), various proposals for changing pelican status under the US ESA had been developing since the early 1980s (Table 1). An early, published proposal to delist the Brown Pelican species was based on a premature, but realistic and optimistic, review of the beginnings of a population recovery in the SCB (Schreiber 1980); the beginnings of that recovery and concomitant decline of pollution levels were first described by Anderson et al. (1975, 1977).
An early change in status was also proposed as a recommendation for down-listing to “threatened” status by the Pacific Seabird Group (PSG) (Table 1). That earlier petition in 1986 by the PSG (Leschner 1986) was not premature because it only suggested a “down-list” to “threatened” status-a more gradual administrative process. And follow-ing, the PSG continued to pursue this proposal through their Conservation Committee. Then, almost 20 years later in 2004, both California Department of Fish and Wildlife and USFWS alerted various experts and organizations that a 5-year status review, then almost certain reclassification (delisting), was imminent (announced officially by USFWS 2006). A petition by the Endangered Species Recovery Council to delist the subspecies (USFWS 2006) accelerated that process in the final stages.
Also, there had been a larger-scale recovery of Brown Pelicans throughout a major proportion of their North American range that also included other “calls for action” to reclassify the entire species (USFWS 2006, not reviewed here). Thus, the USFWS made a decision to include all sub-species in their review and recommendations, and finally to delist the remainder of the species’ populations that had not already been delisted earlier (USFWS 2008).
Post-delisting monitoring was, however, required by the ESA (provided funds were available) and a plan was formally proposed by the USFWS (2009b, 2009c). In Mexico, one federal pelican monitoring plan (Godínez-Reyes et al. 2006) continued through at least 2016, and it will likely continue into the future (Table 1). Of course, adequate funding support remains the key to success in any future environmental monitoring program (Ezcurra et al. 2002). Detailed monitoring in the SCB was delayed at least 5-6 years despite a viable plan (USFWS 2009c). Starting in 2016 and continuing at least through 2020, monitoring has recommenced at Anacapa Island and Santa Barbara Island in the SCB, and recent monitoring was also taken-up along the northwest coast of Baja California by Aguirre-Muñoz et al. (2015).
Incidental monitoring activity (http://www.nps.gov/chis/naturescience/restoring-anacapa-island-sea-bird-habitat.htm) indicated that SCB pelican breeding numbers as well as productivity (in that population segment that was originally endangered; Anderson and Gress 1981) had declined. Some recent estimates indicated low breeding numbers comparable to 1969-1970 (FG, unpublished field notes), when the sub-species had been originally declared endangered. A long, recent, multi-year (~7 yr) SCB breeding depression was ironically almost coincidental with pelicans being delisted. Pelican breeding surveys on Anacapa, Santa Barbara, and Coronados Islands (Fig. 1a) prior to 2003 (and including 2006, e.g., Anderson et al. 2013) included complete counts of successful and abandoned nests, chicks fledged, and chick mortality. However, with shrinking budgets, post-2002 surveys in the SCB were reduced, more limited in scope and detail, and missing in some years.
MacCall et al. (2016) reported that several species of marine wildlife had shown recent indications of food stress associated with a non-fishery-induced collapse in the bio-mass of a major SCB prey item off California (Anderson et al. 1980, 1982; Anderson and Gress 1984), the northern anchovy (Engraulis mordax). Sydeman et al. (2015) have also documented a quarter-decade overall decline in seabird abundance in the SCB, likely including the pelican. Despite very low northern anchovy abundance since about 2009 and continuing through 2015, this key-industry food-source was hypothesized by MacCall et al. (2016) also to have been concentrated inshore, appearing deceptively abundant. Biologists reported signs of food-stress in some species (but not all) of Pacific wildlife in the California Current System as early as 2013 (Leising et al. 2014) and likely even earlier in Pacific seabirds over a widespread area (Humphries et al. 2015). Regarding the recent status of the California Current, Leising et al. (2014) also stated: “...fall 2013, the California current system underwent a major change in phase... catches of sardine and anchovy were low throughout the CCS”. This all had a direct bearing on the pelican.
It has been our intent here to summarize (Table 1) only those events related to conservation of the California subspecies of Brown Pelican since passage of the Migratory Bird Treaty Act with Mexico in 1936, in order to add perspective on the baseline data following. An administrative decision, under recent recovery criteria, to delist the entire Brown Pelican species, would have been difficult to challenge. An apparent, significant population recovery in the SCB, along with long delays between management decisions and actions, begged some kind of management action. Yet, many conservationists remained concerned, partly because detailed pelican-specific, post-delisting monitoring had not been accomplished in the SCB, where the original endangerment of the pelican subspecies occurred and where significant long-term oceanographic changes had also begun to occur.
Objectives
Because the endangered status of P. o. californicus was originally based on severe, pollution-related declines in the SCB area in the 1970s, and because of recommendations in the original recovery plan ( Gress and Anderson 1983), we continued (since 1969, see Keith et al. 1971) to monitor the breeding populations of pelicans in the Midriff Region of the Gulf (Fig. 1b) through the listing and post-delisting period, nearly 50 yr. This was important because: (1) by far, the largest breeding numbers of the subspecies are and always have been located in the Gulf; (2) for various reasons, recovered numbers in the SCB were not monitored in detail during much of the immediate post-delisting period following 2009 (previous discussion); and (3) recent, developing conservation issues in the Gulf during the last decade or two now also have the potential to begin negatively affecting even these previously “secure” breeding colonies (Anderson et al. 2013; Velarde et al. 2013, 2014, 2015a).
In this paper, (1) we review the known, recent statuses and associated environmental conditions for breeding colonies from the Midriff Region of the Gulf (Fig. 1) from 2007 to 2016; (2) we compare these recent data to data from 1970 through 2006; and (3) we evaluate and briefly compare our recent data to recent information from other parts of the geographic range.
Materials and methods
Study sites and zonal separations
The normal, known range of P. o. californicus (e.g., Shields 2002, Anderson et al. 2013) extends from about 16ºN to about 48ºN latitude, or about 8,000 km of coastline distance. The Comisión Nacional de Áreas Naturales Protegidas (CONANP, National Commission for Protected Natural Areas, Mexico) pelican monitoring program began in 2006 when some of us participated in an international aerial survey of the entire range of the subspecies, or metapopulation (see appendices to Anderson et al. 2013 at http://www.marineornithology.org/cgi-bin/getpage.cgi?vol=41&no=2). CONANP thereafter selected a major portion of the Gulf subpopulation for future long-term population monitoring and to monitor individual health of pelicans and other sentinel species (Godínez- Reyes et al. 2006) (Fig. 1b), a representative sampling unit for long-term subspecies monitoring. This region represents the population center of California Brown Pelican total breeding range (concept after Mayr 1963; specific distribution described by Anderson et al. 2013). The GOC subpopulation, ecologically, represents a desert-island, open-ground nesting group, and nesting activities are therefore easily observed from an offshore boat and from strategic non-disturbing distances on shore (e.g., Anderson 1988).
Our colony and sub-colony definitions followed those provided by Anderson and King (2005) for American White Pelicans (Pelecanus erythrorhynchos): colony = a breeding aggregation associated with a distinct geographic feature, usually an island; a sub-colony = discreet grouping within a colony, but somewhat separated from it. We also explored the idea of “satellite colony” (Anderson 1983; satellite colony = small breeding aggregation on a separate island which behaves demographically the same as the larger aggregation on another nearby island). These examinations were intended first to develop regression equations to assist derivation of total numbers of nests in the San Luis Gonzaga region (northern Midriff, Fig. 1b), when total census was often not possible for safety reasons (usually severe wind and water conditions). A likely satellite colony, Cholluda Island (also named La Encantada) (30.0ºN, 114.5ºW), was located about 8 km from San Luis Island (also called Salvatierra Island or Encantada Grande Island), the north region’s major nesting colony. Secondly, we also examined the potential relationship between Piojo Island in the Bahía de los Ángeles Archipelago, a local but easily accessible island (Fig. 1b), to the nearest and largest breeding colony in the subspecies’ range (San Lorenzo Archipelago, ~65 km distant) for possible future use as an easily accessible representative sampling site.
Although oceanic boundaries are variable and dynamic and some biological connectivity is high in the entire Gulf (Turk-Boyer et al. 2014), San Luis and Cholluda Islands and other nearby pelican nesting islands (e.g., Puerto Refugio) are likely within a somewhat distinct ecosystem, variably defined as the “northern Gulf” (e.g., Hendrickson 1973; Maluf 1983; Santamaria-del-Angel et al. 1994; Álvarez-Borrego 2002, 2003; Hastings and Findley 2007, 2010; Lercari and Arreguín-Sánchez 2008; Cisneros-Mata 2010; see also reviews by Herrera-Cervantes et al. 2007 and Páez-Osuna et al. 2016). Northern Gulf uniqueness is likely related to high tidal influences and other more local features, remaining still productive offshore in the northern Gulf despite extreme changes farther up in the Colorado River delta area (Álvarez-Borrego 2002, 2003; Ramírez-León et al. 2015; Brusca et al. 2017).
Santamaria-del-Angel et al. (1994) have provided the most recent quantitative definitions of northern Gulf zonation based on Coastal Zone Color Scanner estimates of chlorophyll (a measure of primary productivity). Their ecogeographic zones II and IV are geographically closest to our northern Midriff pelican colonies. Brusca et al. (2017) separated the Gulf into 3 major ecological zones, north, central, and south, although further study may require more separation. Of course, another key factor in any geographical separation of nesting seabirds is simply the physical presence or absence of suitable, undisturbed islands near reproduction-sustaining food sources (Anderson et al. 1982, Anderson 1983). All these characteristics helped further justify our separations in describing baseline values for conservation planning, although specific biological and environmental boundaries likely vary in time and space.
El Niño/Southern Oscillation (ENSO) events also strongly influence breeding effort of pelicans and other seabirds (Ainley et al. 1988, Anderson et al. 2013, and many others), and ENSO cycles act as a major ecological driver in the Gulf (Baumgartner and Christensen 1985, Herrera-Cervantes et al. 2007, Páez-Osuna et al. 2016). Thus, we separated ENSO and non-ENSO periods (based on Oceanic Niño Indices [ONI], http://ggweather.com/enso/oni.htm, http://www.cpc.ncep.noaa.gov/), as well as north and south breeding zones (Fig. 1b), in both baseline and recent data because of their ecological differences. It has also been demonstrated that there is a northward progression of the ENSO signal toward the northern Gulf (Herrera-Cervantes et al. 2007), and we hypothesized that pelican colonies in the north might be less affected by the ENSO signal.
San Pedro Mártir (DWA, field notes), Alcatraz (LA and LM, field notes), San Pedro Nolasco (JT-V, field notes), and Chaperona (DWA and JT-V, field notes) Islands (Fig. 1b) were also included in some of our analyses because some limited recent data were available. These colonies also represent “central Gulf” as described by Brusca et al. (2017).
Breeding-season censuses
Annual censuses of new nests (expressed as peak number of NA) were conducted 3-5 times per breeding season, depending upon pattern and length of any particular breeding season. Abandoned nests of the year were included in NA estimates. Our recent monitoring period, 2007-2016, provided 10 yr of recent CONANP monitoring data. This encompassed the year of delisting, 2009 (a policy-related but not biologically-related event). Additional colonies outside our main study area were visited only 1-3 times per breeding season. Baseline data (1970-2006) were summarized from Anderson et al. (2006) and DWA (field notes).
Each season, early (January-March) reconnaissance was conducted by CONANP patrols and/or personal communications with resident fishermen to help determine the beginnings and extents of the pelican breeding effort and initial estimates of the season’s breeding numbers and phenology for later, same-season planning. More precise censuses later in the season were timed to obtain major quantitative data at or near each seasonal peak of nesting after most young were about 3+ weeks of age (partly to avoid the effects of disturbance, Anderson and Keith 1980), and to determine if the breeding season might have a bi- or multi-modality. In cases of multi-modality and longer breeding seasons, more visits were required. NA values also essentially represent peak numbers of breeding pairs, whether successful or not.
Breeding colony census methods were essentially the same as generally described by Anderson and Gress (1983) and Anderson et al. (2013), conducted from a boat or on the ground from non-disturbing distances. Nesting activity was located and mapped, and numbers of nests or breeding pairs were counted or estimated via units of 5, 10, 50, or 100 to determine the total approximate nesting effort in each sampled area, canyon, or other easily-identifiable geographic feature. Age-structure of young in weeks was sampled from clearly visible areas. When our field crews later entered nesting areas at a time with much less potential disturbance damage, pelican young were measured, weighed, aged, and sampled. Regurgitations were documented and final nest occupancy rates were estimated. The distribution of age-classes of young (in weeks) was used to describe phenological stages at each nesting colony each year. Much of this additional information will be published separately, however.
Nesting aggregations were enumerated as follows: (1) if the entire nesting aggregation in view could be seen, all nests and nest structures were counted; (2) if only parts of a nesting aggregation could be seen, due to geography, visible parts were enumerated and extrapolated to unseen areas. If activity could be seen on both sides of the not-seen areas, we assumed activity there, as well, and extrapolated numbers to the unseen proportions. If no activity could be seen on one side only of an obstruction, we extrapolated nesting half-way into the unseen portion, based on counts in the visible sample. In larger areas where we mapped the extent of nesting activity but did not census total nests, we extrapolated nest densities from the more carefully censused areas to the uncensused areas of known occupancy, entirely mapped for each island for each breeding season. In some areas where hundreds or thousands of nests were present, estimates were made by enumerating nests in readily-definable areas, then extrapolating these densities to the remainder of the nests not counted, but in known-occupied areas.
Longer-term breeding numbers (also expressed as NA, determined the same way) were summarized from Anderson (2013 and DWA [field notes]) and presented here as baseline, long-term NA expectations representing the same Midriff study areas. We selected the aerial survey year 2006 (Anderson et al. 2013) as our ending date for “baseline” and 2007 as the beginning date for “recent”. These separations were necessary to evaluate NA, comparing “baseline data” gathered by DWA and colleagues from 1970-2006 (n = 37 yr) vs 2007-2016 (n = 10 yr; Godínez-Reyes et al. 2006).
We further examined pelican breeding efforts for additional colonies south of our CONANP monitoring area, for 2 reasons: (1) it had already been demonstrated that ENSO effects are more pronounced to the south in the Gulf (Anderson et al. 2006), and (2) some colonies south of our study area are also closest to the highest industrial, trawling fishery activities, which potentially affect seabirds such as the pelican (Velarde et al. 2015a).
In this paper, NA (or initiating breeding pairs, based on total nests built) are reported, not total individuals, which for this subspecies has been systematically and completely enumerated only once (Anderson et al. 2013): individuals of P. o. californicus in 2006 = 195,900 ± 7,200, all age classes, preceded by normal reproductive output. Short-term variability in NA varies annually with (1) proportion of mature adults that breed each year ( Anderson and Gress 1983), and (2) other positive or negative contributing factors such as variable cohort survival and local effects on local reproductive success. Long-term trends of NA as a population index, despite year-to-year independence (see results), nonetheless, are useful tools in evaluating recent population status, providing that multiple-year trends and comparisons are made with natural variation in mind.
Measurements, data treatment, and statistics
Estimated recent nesting populations and seasonal breeding effort or output were represented as NA, productivity (PRDY) (as large or fledged young per NA [YY • NA-1]), and/or total number of near-fledged young produced (Production [PRDN] = NA • PRDY). To evaluate recent breeding population changes, each breeding colony’s PRDN value for our recent time-period was converted to a percentage of historical or baseline values for a given colony.
Baseline versus recent comparisons to test the hypothesis of recent nesting population changes for north and south Midriff zones were also conducted. Both parametric (analysis of variation, regression, and un-paired t-tests) and non-parametric statistical tests (Spearman’s rank correlations, r s) were performed with nearly identical results, but only non-parametric significance levels are reported here.
These and other summary statistics and tests were conducted with the programs Minitab 16 (http://www.minitab.com, see also Ryan and Joiner 1994) or Statistix 10 (http://www.statistix.com, see also Analytical Software 2008). F-tests were conducted as described in Sokal and Rholf (2011) with statistical tables from Rholf and Sokal (2012). In Tables 2 and 3, sample sizes and numbers given do not always sum exactly because of the various combinations from yearly samples used in the various categories combined, i.e., surveys were not conducted at every breeding colony every year because samples were taken as opportunity and funding allowed.
Table 2 Baseline, long-term means of California Brown Pelican nesting-effort (nesting attempt) estimates on islands in the Gulf of California study area (Fig. 1b), during 1970-2006, by non-ENSO and ENSO conditions, and by all years combined and latitudinal region (ENSO years were defined from http://www.cpc.ncep.noaa.gov/products/).1,2
Region1—Location | # years w/data3 | Mean (# nests)3 | Range (# nests)3 | SD | SE | CV % |
DURING NON-ENSO YEARS: | ||||||
North—San Luis Gonzaga Area4 | 22 | 6,940 | 1,190-17,550 | ±4,350 | ±930 | 63 |
North—Ángel de la Guarda Island | 18 | 1,675 | 240-6,575 | ±1620 | ±380 | 97 |
North colonies combined, non-ENSO | 16 | 9,525 | 3,210-18,290 | ±4,740 | ±1,185 | 50 |
South—Piojo Island | 26 | 505 | 45-1,430 | ±315 | ±60 | 62 |
South—San Lorenzo Archipelago5 | 25 | 11,640 | 5,905-20,100 | ±4,075 | ±815 | 35 |
South colonies combined, non-ENSO | 24 | 12,340 | 5,950-21,530 | ±4,140 | ±845 | 34 |
DURING ENSO YEARS: | ||||||
North—San Luis Gonzaga Area4 | 7 | 4,640 | 150-13,600 | ±5,180 | ±1,960 | 112 |
North—Ángel de la Guarda Island | 9 | 1,140 | 300-2,480 | ±665 | ±255 | 59 |
North colonies combined, ENSO | 7 | 5,785 | 450-14,600 | ±5,100 | ±1,930 | 88 |
South—Piojo Island | 8 | 150 | 0-300 | ±100 | ±35 | 66 |
South—San Lorenzo Archipelago5 | 7 | 3,300 | 0-10,000 | ±3,345 | ±1,265 | 101 |
South colonies combined, ENSO | 7 | 3,430 | 150-10,275 | ±3,405 | ±1,285 | 99 |
DURING ALL YEARS COMBINED: | ||||||
North—San Luis Gonzaga Area3 | 29 | 6,385 | 150-17,550 | ±4,580 | ±850 | 72 |
North—Ángel de la Guarda Island | 25 | 1,530 | 240-6,575 | ±1,420 | ±285 | 93 |
North colonies combined, all years | 23 | 8,390 | 450-18,290 | ±5,050 | ±1,055 | 60 |
South—Piojo Island | 34 | 420 | 0-1,430 | ±320 | ±55 | 75 |
South—San Lorenzo Archipelago5 | 32 | 9,815 | 0-20,100 | ±5,225 | ±925 | 53 |
South colonies combined, all years | 31 | 10,330 | 150-21,510 | ±5,460 | ±980 | 53 |
TOTAL MIDRIFF (North + South), All Years | 22 | 18,170 | 805-34,225 | ±9,045 | ±1930 | 53 |
1The census area (Midriff zone) was separated by north and south breeding colony regions (see text), then combined in various ways here.
2Mean (arithmetic), range, standard deviation (SD), and standard error (SE) were rounded to the nearest 5; coefficient of variation (CV) was rounded to the nearest whole number.
3Sample-sizes, means, and ranges do not sum exactly in the various categories because summed samples came from slightly different year combinations.
4This total included either actual censuses or projections (see text) for Cholluda Island, a satellite breeding colony of Salvatierra Island.
5This area included occasional brown pelican nesting at Partida Norte Island and Salsipuedes Island in addition to almost consistent nesting on Las Ánimas Island and San Lorenzo Island.
Table 3 California Brown Pelican breeding effort (expressed as nest attempts [NA]) in colonies outside the Midriff study area in the Gulf of California.
Location (years): Condition | N (years) | NA ± SE1 | ±SD1 | Range | CV1 |
San Pedro Mártir Island (1971-2006): ENSO | 3 | 100 ± 50 | 90 | 0-175 | 90 |
San Pedro Mártir Island (1971-2006): Non-ENSO | 9 | 3,490 ± 520 | 1,550 | 700-6,000 | 44 |
Alcatraz Island (2004-2013)2: Overall | 8 | 440 ± 150 | 420 | 75-1,370 | 96 |
San Pedro Nolasco Island (2006-2013): Overall | 8 | 700 ± 300 | 850 | 0-2,580 | 122 |
Chaperona Island (2005-2013)3: Overall | 9 | 290 ± 65 | 195 | 0-560 | 67 |
1See Figure 1b; NA, standard error (SE), and standard deviation (SD) rounded to the nearest 5, and coefficient of variation (CV) rounded to the nearest whole number.
2Data from 2004-2006 were reported by Galván (2007).
3California Brown Pelican nesting at Chaperona Island was first recorded in 2005, with about 75-100 NA (JT-V, field notes; LT Findley, pers. comm.).
For additional comparisons to breeding characteristics, the highest November, December, and January ONI scores were compared to nesting effort over the most recent CONANP monitoring period. And because of strong ENSO-like conditions in the Gulf in 2014 (Yuan and Yamagota 2014) (although not defined [see above ONI website and Su et al. 2014] as technically an ENSO year), we increased our category value for 2014 by one, but prior to any data analysis.
Piojo Island (Fig. 1b) was the breeding colony with the largest continuous annual samples, and also with well-documented human disturbance events (Anderson and Keith 1980, Anderson 1988, Anderson et al. 2006). PRDY (= YY • NA-1) from Piojo Island (29.0ºN) was related to Southern Oscillation Indices (SOI) here, rather than ONI values, in order to provide a direct quantitative comparison to a similar data analysis by Velarde and Ezcurra (2002) for Heermann’s Gulls (Larus heermanni) from nearby Rasa Island (28.8ºN).
Because of confounding variables, to test for the most recent warming on a north-south gradient of nesting effort and PRDY in the Gulf, non-parametric r s values (Ryan and Joiner 1994) were used, as well as conversions of measurements to quantitatively-comparable values: nesting effort (NA) and PRDY were converted to percentages of long-term baseline NA values and percentages of the long-term, base-line recruitment standard (see discussion). Our 2 measurements of yearly reproductive output in the most recent Gulf warming period were combined as the product of the 2 measurements (NA • YY-1 or PRDY, and %NA of baseline, %NAb) to derive a “breeding index” (PRDY • %NAb) for the most recent 3-year warming period, 2014-2016, for each of our sampled colony-areas. These values were correlated with straight-line kilometer distances of each colony site from the center of the “mouth” of the Gulf (approx. 22.4ºN, 108.2ºW). A curve of this relationship was fitted with quadradic regression analysis, but non- parametric correlations (r s) were used to statistically evaluate a south-north warming effect on pelican reproduction because of unknown possible confounding environmental variables that remained unquantifiable, such as unknown local factors. Breeding index values of ~90 to 110 should approximate baseline, long-term expectations (without disturbances, see Fig. 2), i.e., 100% baseline NA * 0.9-1.1 YY • NA-1 = 90-110. We used breeding index as a simple but useful index to reproductive output averaged for the most recent 3-year warming period to further test for a north-south effect in the Gulf.

Figure 2 Relationship between baseline (1970-2006) pelican productivity values (young [YY] fledged per nest attempt [NA]) and mean winter Southern Oscillation Index (SOI) values at Piojo Island, Gulf of California, showing non-ENSO, ENSO, and disturbance conditions (only the mean productivity value is shown for disturbance) (data modified from Anderson et al. 2006). A simple linear relationship is significant at P < 0.001, and 95% confidence intervals are indicated by short-dashed lines.
Results and discussion
Satellite breeding colonies and inter-colony relationships in baseline data
We found a close relationship (P < 0.001) between the breeding effort of pelicans at San Luis Island (SLU) and nearby Cholluda Island (CHL) (Fig. 3a), as well as Piojo Island (PIO) and San Lorenzo Archipelago (SLA) (P < 0.01) (Fig. 3b). The best fit for SLU vs CHL was a polynomial regression (R 2 = 90%, P < 0.01, CHL = 0.016 SLU + 0.000017 [SLU2]), but a simple linear fit was also sufficient (R 2 = 86%, P < 0.01, CHL = 0.252 SLU - 634). Applying parsimony, we used the simpler relationship (considering CHL as a satellite colony, or sub-colony of SLU) for our total estimates of breeding numbers in the northern segment of the Midriff zone (Fig. 1b), applied when census data were not available for CHL. PIO also bore a statistically significant relationship (P < 0.02, R 2 = 80%) in recent (2006-2014) breeding populations compared to those in the SLA. The reductions of NA by ENSO events were also essentially the same comparing PIO and SLA (χ2-test = not significant). Combined, the mean reduction in breeding effort (NA) associated with ENSO events in the south zone was -69% (P < 0.001, d.f. = 18).
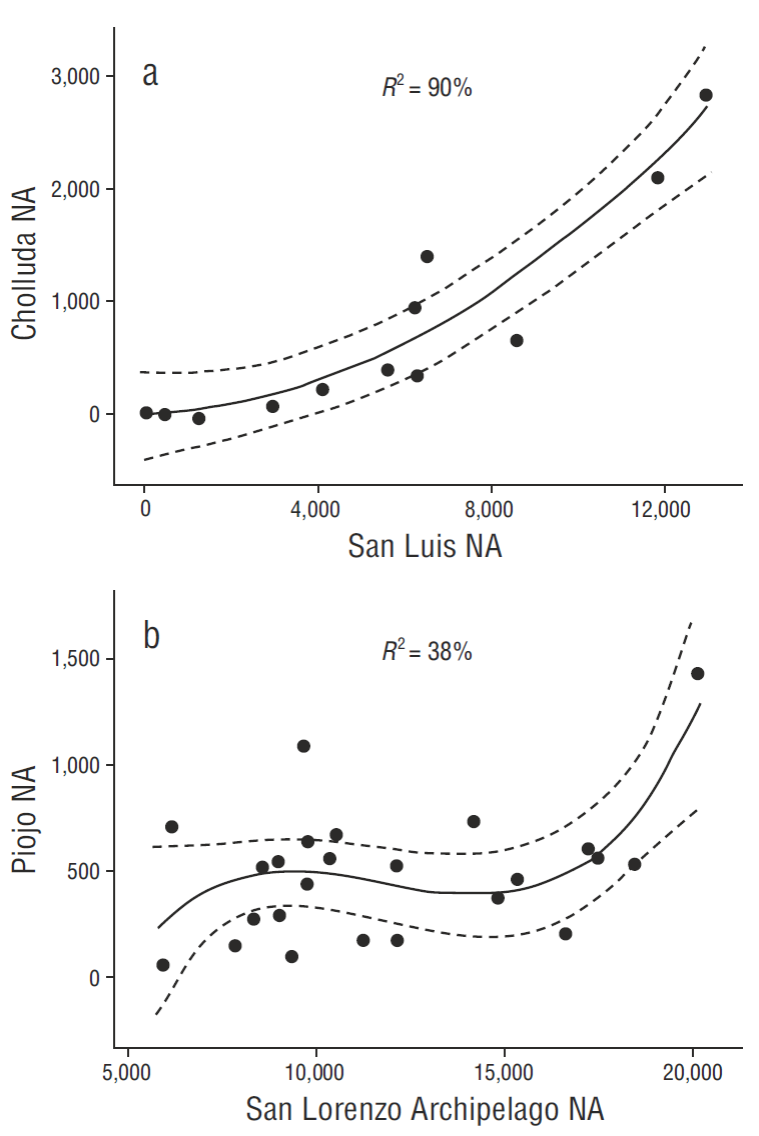
Figure 3 Relationships between large and small Brown Pelican breeding colonies in the north (a) and south (b) parts of the monitoring area in the Gulf of California. NA = nesting attempt.
Previous human disturbance events may have confounded the relationship between PIO and SLA, as indicated from PIO productivity (PRDY) data (Fig. 2, modified from Anderson et al. 2006). PRDY is closely related to NA and both are potentially reduced by human disturbances (Anderson and Keith 1980) in addition to changing oceanographic conditions such as warm water. But, this strong PIO/ SLA connectivity (in the absence of disturbance) was therefore also further justification for inclusion of PIO plus SLA as a separate category (south Midriff) for baseline values (Table 2) and recent data summaries.
Baseline, long-term (1970-2006) breeding populations in and near the monitoring zone
Year N vs Year N + 1 comparisons
In our baseline data (Table 2), nesting populations (NA) were not serially correlated in either north or south population segments (P = 0.81 and P = 0.45, respectively), indicating independence from one year to the next in breeding effort. Nor was there any serial correlation in the most recent decade (2006-2015) in our north and south categories (P = 0.42 and P = 0.99, respectively).
Baseline breeding numbers
Linear trend models with linear regression for north zone nest attempts (NA t = 6,700 + 84t, where t is the year number starting with 1970 = 1) and south (NA t = 10,800 - 23t) indicated little (north) or no (south) change over the baseline period, 1970-2006 (Fig. 4, n = 37 yr) . Northern trend analysis changes were slightly outside the 95% confidence level (CL) of mean baseline values (Table 2), indicating a small northern increase in NA (3,110 by model; 2,110 by CL) (further evaluated in following paragraphs) . Southern trend analysis changes were well within the 95% CL of mean baseline values (Table 2) (850 by model; 1,960 by CL). We therefore determined it justifiable to use data from 1970 to 2006 as “baseline”, and to conclude there had been no major population changes over that period.

Figure 4 Nesting attempts (NA) from 1970 to 2006 in both the northern (a) and southern (b) portions of the Gulf of California study area, showing stability of nesting effort over that baseline period.
High temporal variability in addition to smaller geographical variability characterize baseline long-term nesting effort in Gulf pelicans (Tables 2, 3). Breeding effort in our Midriff study area varied from ~800 to ~34,200 over the baseline period (overall mean = ~18,200 ± 3,860, 95% CL; CV = 54%; N = 22 yr of complete Midriff data [when data were available for all breeding sites in a given year]). Some smaller colonies occasionally declined to zero NA in ENSO years (Table 2). Additional baseline data are also summarized here from outside and south of our study area (Fig. 1b) (“Central” Gulf of Brusca et al. 2017) for San Pedro Mártir, Alcatraz, San Pedro Nolasco, and Chaperona Islands. Smaller sample-sizes from 4 colonies to the south of the Midriff zone (Table 3) also suggested high levels of variability, from 0 to ~6,000 NA (CV = 44-122%). All pelican and other seabird breeding colonies need to be evaluated with this variability in mind.
ENSO influence on breeding effort
Average reduction of pelican breeding effort in ENSO years, even disregarding the various intensities of ENSO events reported, over the baseline period has been significant (Table 2). Long-term mean reductions in breeding population sizes (1970-2006), non-ENSO vs ENSO years (t-tests comparing years with data, and assuming unequal variances, Table 2), were as follows (baseline data: Anderson 2013), from north to south (Fig. 1b): San Luis Island area = -39.3%, P = 0.163, d.f. = 15; Angel de la Guarda North Island = -43.8%, P = 0.104, d.f. = 15; Piojo Island = -70.7%, P < 0.0001, d.f. = 34; San Lorenzo Archipelago = -68.0%, P < 0.0001, d.f. = 14; total Midriff area, all colonies combined = -56.7%, P = 0.003, d.f. = 12. ENSO influence on breeding effort has been weaker in northern Gulf breeding pelicans. Long-term effects of ENSO apparent in the baseline data (Table 2, above summary) were also evident as a continuing dominant factor in the most recent decade (separately testing for relationships between NA and winter ONI values from 2006 to 2015; Fig. 5 a-c).

Figure 5 Recent relationships between winter Oceanic Niño Index values (ONI, see text) and California Brown Pelican nest attempts (NA) in the north breeding colonies (a), the south breeding colonies (b), and the combined total (Midriff) (c). All regressions were significant at the P < 0.05 or higher significance levels. NDJ = mean for November, December, and January.
An F-test, comparing north ENSO vs non-ENSO variances in baseline data, was also significant (F = 2.89; d.f. = 6,15; P < 0.05), as was the same test, comparing south ENSO/non-ENSO variances (F = 2.59; d.f. = 6,23; P < 0.05), and that variation was also uniformly slightly higher in the ENSO years; coefficients of variation of NA were also higher in the northern breeding colonies than in the south -Midriff (final column Table 2). Yet, in the lower mid-Gulf, outside and farther south of our study area, coefficients of variation were highest, although we could only calculate this for recent data in 3 of 4 cases (Table 3) because of lack of earlier data.
Recent (2007-2016) breeding
Spearman rank correlations were all significant (P < 0.05) when percent baseline values of NA and NA • YY-1 were independently related to distance from the open Pacific (r s = 0.857, P = 0.014 or r s = 0.829, P = 0.021; respectively) or as a combined breeding index (r s = 0.857, P = 0.014) (Fig. 6). In the most recent, extensive warming period then, our data independently supported the hypothesis that the northern Gulf continued to be somewhat less affected by warming events (although still significantly reduced in the most productive areas when north and south warming-period data are compared to baseline). Over the long-term and even recently, ENSO events (or longer-term warm-water conditions such as in the most recent period, which earlier failed to develop into a defined ENSO event due to unusual oceanographic conditions in the Pacific [Su et al. 2014]) dominated variability of breeding numbers as first suggested by Baumgartner and Christensen (1985) for that ecosystem. Connectivity to environmental conditions is likely via pelican (and other seabird) food availability (see Anderson et al. 1982 specifically for pelicans, but a universal paradigm in seabird population biology).

Figure 6 Relationship between distance from the mid-open-ocean entrance of the Gulf of California to the 3-year mean “breeding indices” (see text) of 7 pelican breeding colonies over the same 3-year period of general ocean warming (2014-2016).
Recent warming (subscript “w”) period (“Blob”) NAw and breeding success (PRDYw and PRDNw) compared to expected long-term baseline (subscript “b”) values NAb (Table 2), as well as calculated/observed ratios of PRDYb and PRDNb (Table 4), illustrate an extreme, unprecedented breeding failure in 2014, continuing through 2015 and 2016 (declining in spring) (http://www.cpc.ncep.noaa.gov). This effect was certainly more extreme than expected in even baseline ENSO conditions. Compared to baseline, all measurements of breeding effort and production of young in 2014-2016 were extremely low (north area change in breeding effort, 3 years consecutively = -83.6%, YY • NA-1 = 0.33; south area = -96.4%, YY • NA-1 = 0.14) (Table 4).
Table 4 California Brown Pelican breeding variables for 2014-2016 (recent warm-water period, subscript “w”), compared to historical values (baseline = 1970-2006, subscript “b”) and separated by north and south census zones. PRDN = total production; PRDY = productivity rate; NA = number of nest attempts; YY = young-of-the-year.
Variable | North1 | South1 |
Baseline—NAb (1970-2006, all)2 | 8,390 ± 1,055 | 10,330 ± 980 |
Baseline—NAb (1970-2006, ENSO)2 | 4,705 ± 1,700 | 3,845 ± 1,190 |
Recent warming—NAw (mean 2014-2016)2 | 1,380 ± 970 | 370 ± 200 |
Recruitment standard3 | ~0.9-1.1(1.05) | ~0.9-1.1(1.05) |
Recent warming—PRDYw (mean 2014-2016) | 0.333 | 0.137 |
Baseline—PRDNb (expected)4 | 8,810 | 10,850 |
Baseline—PRDNb (expected, ENSO)4 | 4,940 | 4,040 |
Recent warming—PRDNw (mean 2014-2016)4 | 460 | 50 |
NAw/NAb (all) | 0.164 | 0.036 |
NAw/NAb (ENSO only) | 0.293 | 0.096 |
PRDYw/PRDYb | 0.333 | 0.137 |
PRDNw/PRDNb (all) | 0.052 | 0.005 |
PRDNw/PRDNb (ENSO only) | 0.093 | 0.012 |
1North and south zones are shown and defined in Figure 1b.
2Means are reported with ± 1 standard error.
3Recruitment standard (RS) is a long-term value or a long-term mean NA • YY-1 required to maintain a stable population, long-term (where ʎ = 1, r = 0; or for Brown Pelicans, RS = ~1.05) (Gress and Anderson 1983).
4Approximate needed mean annual PRDNb = RS • NAb.
Pelican breeding farther outside the Midriff and mid-Gulf zones
At Ballena Island, in the far south of the Gulf, near La Paz, Baja California Sur (~24.5ºN, 110.4ºW), EL Palacios (pers. comm.) reported no pelican nesting for the entire 3-year warming period, when ~1,000 NA would be the expected normal breeding population size (see Anderson et al. 2013, appendix 3). In the far south of pelican range on Mexico’s west coast, at Los Arcos Islands, near Puerto Vallarta, Nayarit (20.5ºN), there were only ~40 NA in 2015 (Robert and Helen Martínez pers. comm., who photographed the entire nesting colony). The 2015 NA were reduced by 77% from a colony size at Los Arcos Islands more typical of ~175 nests during non-ENSO conditions (Anderson et al. 2013). Sarmiento (1994) reported year-to-year variation in numbers of NA at Pájaros Island, Sinaloa (23.2ºN), also in the far southern portion of pelican range (Fig. 1a, Mexican Mainland Island [MMI] subpopulation): during the ENSO of 1991-1992, that colony contained 69 NA vs 334 NA in the non-ENSO years 1992-1993, a 77% reduction. Our 2015 estimates at Los Arcos likely represented a more “typical” pelican response to ENSO conditions.
In the range peripheries, where NA and reproductive success were somewhat higher than in the Midriff (Fig. 1a), pelicans still performed sub-optimally. For example, in 2014-2015, the reported reproductive performance in the SCB (about 34.0ºN) was ~76% below a long-term mean of about 0.90-1.05 YY • NA-1 (see below). Unfortunately, breeding effort and breeding success in the SCB began declining in about 2010 after 2009 delisting, an ENSO year. However, the breeding numbers after 2004 at Anacapa Island, California, gradually declined to about 1,200 in both2009 and 2010 with even fewer in 2011 (FG, field notes); the 2011 breeding effort failure was nearly complete as well, with only one chick fledged. Ironically, this was reminiscent of 1970 when only one chick fledged from a colony consisting of 550 nests at Anacapa Island during the height of the DDT era. From 2012 to 2015, breeding effort and productivity somewhat improved, but was still far below the long-term “recruitment standard” (0.9-1.1 YY • NA-1) estimated required for population maintenance (Schreiber 1979, Gress and Anderson 1983). Another example from SCB in 2012 on Santa Barbara Island, California, 16 chicks fledged from about 400 nests (data for Anacapa Island were unavailable for 2012, and there were no systematic surveys in 2013). In 2014, about 1,300 chicks (a projected mean of 2 estimates) fledged from about 5,300 NA on Anacapa Island (Harvey and Mazurkiewicz 2015), and in 2015, there were ~2,000 NA producing ~1,300 fledged young (D Mazurkiewicz, pers. comm.), approaching normal productivity (PRDY = 0.64), the highest-producing colony known in the range of the subspecies in 2015. Perhaps these breeding pelicans were utilizing local concentrations of inshore anchovies (MacCall et al. 2016), although SCB pelican food habits were not documented. In early 2016, DWA and FG estimated ~1,900 NA at Anacapa (final PRDN estimates were not available at the writing of this report), still far below the ~5,000 NA expected (Anderson et al. 2013, appendix 1). In 2014, Howard et al. (2015) reported ~78 YY fledged in ~500 NA (or ~0.16 YY • NA-1) at Santa Barbara Island (33.5ºN). Reproduction and NA were also far below “normal” in both 2014 and 2015 in pelican breeding colonies at 7 islands or island groups along the west coast of the Baja California peninsula (~27.1º-32.4ºN) (Aguirre-Muñoz et al. 2015).
Current status: recent (2007-2016) versus baseline (1970-2006) pelican breeding efforts in the study area
Variances were compared by F-test and found to be equal in both north and south categories, P > 0.10 and P > 0.50, respectively. The t-tests comparing baseline nesting (NAb) vs recent breeding population indices (NAr) in the 2 breeding zones indicated no changes in the north zone (P = 0.81, mean = 8,390 ± 1,055 NAb [± SE] vs 7,485 ± 2,080 NAr), but statistically significant recent changes in the south zone (P = 0.03, NAb = 10,330 ± 980 vs NAr = 5,865 ± 1,950). This was a recent decade reduction in the south, 2007-2016, of -43.2%.
A decline in breeding numbers at San Pedro Mártir Island appears to have been more severe and starting earlier than we observed for the south-Midriff. Breeding effort at San Pedro Mártir Island, outside of our CONANP monitoring area (Fig. 1b), over the baseline period (Table 2 and Anderson 2013), and combined with our recent NA estimates, indicated a significant, long-term decline there (Fig. 7), and therefore likely breeding population declines larger than seen in the south-Midriff, notable sometime after the 1990s at San Pedro Mártir but more recently at other colonies in the south-Midriff zone (Piojo Island + San Lorenzo Archipelago).
Synthesis and conclusions
The 2014-2016 period represented an unprecedented, continuous breeding failure or under-performance of pelicans and other seabirds (in nearly half a century of study by DWA and since studies in the Gulf began in 1969, Keith et al. 1971), largely range-wide (see also news article perspective by Kintish 2015 and Velarde et al. 2015b). Typical views comparing this contrast between optimal (baseline) and sub-optimal (warm-period) conditions are clearly illustrated in Figure 8.

Figure 8 (Upper) A subcolony of Brown Pelicans on San Luis Island in May 2011 showing “normal” reproduction at the time of expected fledging. In 2011, this subcolony contained ~330 nest attempts (NA) and produced ~345 young-of-the-year (YY) (= 1.05 YY • NA-1). (Lower) The same subcolony in May 2014, the beginning year of a 3-year warming. In 2014, this subcolony contained 2 YY from ~55 NA (= 0.04 YY • NA-1). Photos: DW Anderson.
Although nesting effort was better in colonies on the range peripheries, it was still sub-optimal. This breeding failure in pelicans was similar to that observed in many other Pacific seabirds (e.g., Anchundia et al. 2014, Humphries et al. 2015, MacCall et al. 2016), but apparently not universal in all Pacific seabirds (Sydeman et al. 2015).
Most of the recent, widespread pelican breeding failures have been characterized (1) by failures of breeders to arrive at nesting colonies in expected numbers, (2) by abandonment and large-scale, earlier- than-expected migrations of those birds that did arrive, and (3) sub-optimal breeding in those few birds attempting to breed. The range-wide, breeding failure was most extreme in 2014-2016 (but improved in 2016). It continued as ENSO extended to late-spring 2016 (http://www.cpc.ncep.noaa.gov/products/analysis_monitoring/enso_advisory/index.shtml).
Piojo Island and the town of Bahía de los Ángeles are in the region nearest the largest nesting populations for the entire subspecies. Piojo Island is close to the mainland (12 km) and accessible (Fig. 1a). If strictly protected from human disturbances (Anderson and Keith 1980, Anderson and Palacios 2008; Fig. 2), the Bahía de los Ángeles area would be a viable monitoring location for both breeding and dispersing Gulf pelicans. San Luis Island to the north (about 18 km from San Luis Gonzaga Bay) has similar characteristics for regular and convenient monitoring. Nonetheless, we expect that more protection of nesting seabirds will be necessary in both localities because of a new highway and new developments that are beginning to attract many more people than previously (2016 and beyond).
Overall, pelican colonies in our Midriff study area were reduced varying degrees by ENSO throughout both baseline and recent study periods, but the strength of the effect over the entire study period (1970 to present) was apparently weaker and/or less consistent in the northern Gulf. This implies that the ENSO effect has varying degrees of “penetration” into the northernmost breeding colonies of the Gulf, perhaps slightly less influential in the northern ecosystems due to additional ecological effects from strong northern tidal influence on food sources, unique bottom substrate, very high primary productivity (Brusca et al. 2017), and more local effects of a northern gyre as described by Álvarez-Borrego (2002), creating unique local ecological conditions for breeding pelicans.
Contrasting baseline non-ENSO + ENSO expectations, it is apparent that, when comparing unprecedented, low 2014-2016 pelican breeding parameters, breeding effort and success were much more severely reduced than demonstrated by all previous data (spanning nearly 4 decades of baseline data), even when compared only to ENSO expectations (Table 4).
Because shifting breeding effort and highly variable breeding effort and success is an adaptive, ecological characteristic of seabirds like the pelican, long-term population status and trends are difficult to determine with certainty, whether pelican numbers have experienced recent declines, or whether the observed behaviors have been an early sign of impending problems, or if the very-adaptable pelican is experiencing a rare or cyclic low point in a natural cyclic phenomenon. Outside of areas of highest likely interactions with a declining commercial fishery (Velarde et al. 2015a, Velarde and Ezcurra 2016, previous discussion), breeding numbers seem to be stationary at least through 2016 in the northern portions of our study area, but not in colonies to the south.
Uncertainty (typical in seabird demography and its associated environmental conditions, Sydeman et al. 2015), however, in our various approaches to characterize population statuses may also be confounded by the phenomenon of range-shifting within and outside the Gulf. But this was not substantiated in the north-south comparisons for pelicans, discussed above. There have been no concomitant increases in the north to account for the decreases observed in the south of the Gulf. Still, another species of Gulf seabird, the Elegant Tern ( Thalasseus elegans), has made a partial range-shift during this same time period (~10% of expected Rasa Island Elegant Tern populations shifted to the SCB) (Fig. 1a) (Velarde et al. 2015b).
Several questions need further clarification: (1) Can Brown Pelican populations adapt to long-term warming effect? (2) Can the well- known Brown Pelican adult longevity help recover quickly from such demographic challenges, and if so, in how long? (3) Is this unprecedented warming effect exacerbated by the known heavy/excessive fishing activities on major prey items of pelicans and other marine wildlife, and will we observe adaptive food-habit changes (e.g., Ainley et al. 2015 and associated papers)? (4) With warming, can we expect or predict increasing numbers of breeding pelicans in the California Current Region? Proximate and ultimate cause(s) remain unclear but seem consistently related to warming events in the baseline period, now likely exacerbated by increasing frequency and intensity of warming events (Cai et al. 2014, Yuan and Yamagata 2014), and changes in available food sources (e.g., MacCall et al. 2016). Perplexing recent changes seem related to unusual events that are importantly associated with what some marine biologists have defined as a regime shift in the Pacific (e.g., Leising et al. 2014, MacCall et al. 2016) and elsewhere (e.g., Wernberg et al. 2016), likely related to complex developing effects of climate change (Sydeman et al. 2015).
Local subpopulations of pelicans and other seabirds respond first and most strongly to perturbations and decimating factors that are close to their breeding sites during the season of breeding when they are more restricted in their movements to more local feeding areas (Anderson et al. 1982). It follows that seabirds would also be especially vulnerable to commercial fishing activity as reported by Velarde et al. (2015a) for sardine fishing in the Gulf. Possible interference from a commercial fishery (i.e., Furness 2007) in the Gulf is highly likely (exploiting the key foods of pelicans as well as countless other predatory species in the Gulf; e.g., Anderson et al. 1980, 1982; Velarde et al. 1994). A severe decline in sardine catch in the Gulf began before 2014 (and likely much earlier), declining from ~500,000 mt in 2008/2009 to ~3,500 mt at least through 2013/2014 (Cisneros et al. 1995, Velarde et al. 2014). Velarde et al. (2015b) suggested this fishery collapse likely caused changes in number and distribution of Elegant Terns in both population decline and range-shift (see also Fuller et al. 2015 for further discussion on climate change and fisheries).
Regarding the pelican, a similar-scale recruitment of breeders to new areas from distant sources has not yet been observed, with one exception. A past but unrelated situation involved a small proportion of outside recruitment by Gulf-derived pelicans into SCB colonies (perhaps no more than several hundred individuals, Anderson and Gress 1983) during a period when SCB breeders were severely depleted by the effects of oceanic pollution.
Farther-south subpopulations of pelicans (MME and MMI, Fig. 1a) have recently shown indications of impending or current breeding population declines (Castillo-Guerrero et al. 2014). These breeding colonies certainly also warrant systematic monitoring and protection, perhaps justifying such activities by utilizing the pelican as one of the key indicator species of ecological health in the Gulf region (e.g., Anderson et al. 1980, Anderson and Gress 1981).
Recent and unprecedented warm-water conditions with-out an officially declared ENSO event occurred in the Gulf (2009-2010 and 2013-2014). If long-term oceanographic conditions associated with climate change occur as widely expected, various adaptive or negative responses of marine organisms are also likely.
Pelican conservation in the world view and future threats
Many seabird populations, worldwide, have been reported to be in a deteriorated and changing state for various reasons (Paleczny et al. 2015). Potential future impacts of climate change on fisheries (Barange et al. 2014, Pershing et al. 2015) are also likely to impact populations of marine wildlife and overall oceanic biodiversity, because of the close association between mid-water fisheries activities and seabird population statuses (reviewed in the Gulf by Velarde et al. 2004, 2005, 2014). Additional threatening factors also include the likelihood of increasing frequency and intensity of future ENSO events (Cai et al. 2014), plus changes in number and intensity of tropical cyclones (Webster et al. 2005). Sydeman et al. (2015) review the likely variable effects of climate change on marine vertebrates, citing phenological, distributional, and demographic aspects as likely parameters related to these potential effects, both positive and negative. But, if we are really serious about seabird and oceanic biodiversity conservation, it is important that even apparently “healthy” numbers, or those that seem in the beginning phases of potential declines, be carefully and extensively monitored in coming years, and appropriate actions taken to preserve marine ecosystems as intact as possible (e.g., Cury et al. 2011).
The California Brown Pelican is no exception. The sub-species is iconic and a sentinel or indicator species (e.g., Andelman and Fagan 2000) of Pacific seabirds in general. All marine wildlife benefits greatly from conservation efforts often for one or just a few species (for pelicans, see Anderson and Gress 1981). In policy change, actions such as the delisting of the Brown Pelican species have been cited as examples of ESA success (Neel et al. 2012). Although a formal declaration of change in the status of subspecies like P. o. californicus does not instantly change any kind of biological reality, it is what it is: a policy action that will hopefully be positively perceived by the public to help justify the successes and worth of the ESA and other protective measures on both sides of the US/Mexico border. But this should encourage the development of more “modern” approaches, and most importantly for pelicans, to continue active interest in seabird conservation per se. In 1973 terms, the ESA was successful regarding the Brown Pelican species; in 2016 terms, conservationists are calling for more encompassing approaches, including conservation of habitats, ecosystems, and ecological processes, as in the Gulf approach through a network of islands and marine protected areas.
The conservation of individual breeding colonies, peripheral breeding colonies (Lesica and Allendorf 1995), and even historical or suitable colony sites found unoccupied in single surveys, will, however, still be important in achieving ecological and genetic conservation goals for Brown Pelicans, as with all colonial waterbirds. The individual breeding colony or annual roost still represents the most accessible, practical, and easily-definable species management units for Brown Pelicans, as it is for American White Pelicans (Anderson and King 2005).
Future considerations in affecting population status and distribution of pelicans (Páez-Osuna et al. 2016 review current conservation threats in the Gulf in general, and these apply here) must involve these aspects of marine and coastal ecology: (1) effects of climate change (how adaptable will pelicans and other seabird species be, e.g., Wolf et al. 2009); (2) effects of continued change and degradation of coastal habitats (e.g., Naylor et al. 1998, Cruz-Torres 2000, DeWalt et al. 2002, Primerva 2005, Duke et al. 2007); (3) effects of potential direct disturbances and interferences (Anderson and Keith 1980) which have increased (Tershey et al. 1999); (4) effects of emerging diseases (Friend 2002, Rocke et al. 2005, Godínez-Reyes et al. 2006); (5) effects of present and emerging pollutants (e.g., polybrominated diphenyl ethers or PBDEs) (Vander Pol et al. 2012); (6) need for protection and enhancement of current and alternate-breeding sites (Anderson et al. 2013); (7) mitigating the almost certain negative interaction with pelagic mid-water industrial fishing activities that compete for many primary food species of marine wildlife (Velarde et al. 1994, 2014, 2015a; Lindegren et al. 2013; Velarde and Ezcurra 2016); and (8) need for the protection of non-breeding-site roosting areas, also important to a multitude of coastal marine wildlife species (Jaques 1994).
In reviewing the many efforts to continue to study and monitor pelicans as recommended in the “post-delisting monitoring plan” (USFWS 2009b, 2009c), we anticipated that considerable monitoring and study of the species would continue after US delisting. Since the pelican has also been an important icon of marine, coastal conservation since being placed on the endangered species list (Anderson and Gress 1981), a multitude of other non-listed species of seabirds and other marine wildlife have benefited from conservation measures related to the pelican under the ESA as well as to related actions in Mexico. The now well-known tendency of Brown Pelicans to shift frequently amongst colony sites (reported in Panama by Angehr and Kushlan 2007), and for colonies within the metapopulation to “blink-in and blink-out” (a metapopulation phenomenon reviewed in McCullough 1996), dictates that even those unoccupied colony sites as seen in single-year or other short-term surveys have relevance in conservation strategies.
Pelecanus occidentalis californicus subunits have yet to be examined closely for genetic isolation and distinctiveness; yet, genetic isolation is but one criterion characterizing biodiversity’s uniqueness. And given the conservation value of any groups of alike-individuals from different ecologies, behaviors, and geographic ranges (all the way down to local breeding groups or colonies), very local and specific conservation measures are still the most effective conservation measures, but necessary over many locations. Yet, it is certainly most applicable to also understand a seabird’s ecological dynamics and conservation within interacting systems as the ultimate goal of conservation and management (such as we suggest here for pelicans in the northern and southern Midriff area).
We console ourselves with the comfortable fallacy that a single museum piece will do, ignoring the clear dictum of history that a species must be saved in many places if it is to be saved at all.