Serviços Personalizados
Journal
Artigo
Indicadores
-
Citado por SciELO
-
Acessos
Links relacionados
-
Similares em SciELO
Compartilhar
Revista mexicana de ciencias forestales
versão impressa ISSN 2007-1132
Rev. mex. de cienc. forestales vol.7 no.36 México Jul./Ago. 2016
Articles
Growth of Tabebuia donnell-smithii Rose inoculated with arbuscular mycorrhizal fungi and rhizobacteriaa b
1 Facultad de Agronomía. UNACH. Huehuetán, Chiapas. México.
2 Colegio de Postgraduados. Campus Puebla. México.
Tabebuia donnell-smithii is a timber tree with economic and ecological importance in the tropical regions of Mexico, whose natural populations have declined, due, among other reasons, to its slow growth during its establishment. The effect on their growth and nutrition of the inoculation with Rhizophagus intraradices, Azospirillum brasilense and Pseudomonas fluorescens was investigated in Tabebuia plants. The fungus and bacteria were applied in the seeds in combinations of two or three of the following microorganisms: A. brasilense 9 x 106 UFC g of peat-1, R. intraradices with 40 spores g of sterile soil-1 and roots 95 % colonized and P. fluorescens with 9 x 106 UFC g of peat-1 plus the control. They were sown in pots with a non-sterile mixture of Molic andosol soil and washed river sand, 1: 1 (v / v). In total, five treatments were established, with five replications distributed in a completely randomized design. Five destructive samplings were carried out: the first one at 45 days after sowing and the next every 28 days. Morphological and physiological yield variables were recorded. Treatments increased plant growth, with the exception of the two bacteria together after 158 days. The corresponding to P. fluorescens - R. intraradices and that of the three microorganisms induced more biomass in the greater number of morphological and physiological components of yield. The highest content of N and P in plant tissue was obtained with the three microorganisms and with the combination R. intraradices - A. brasilense.
Key words: Azospirillum brasilense Tarrand; Krieg & Döbereiner; aerial biomass; root biomass; inoculation; Pseudomonas fluorescens Migula; Rhizophagus intraradices (Schenck & Sm.) Walker & Schuessler
Tabebuia donnell-smithii es un árbol maderable con importancia económica y ecológica en las regiones tropicales de México, cuyas poblaciones naturales han disminuido, debido, entre otras razones, a su lento crecimiento durante su establecimiento. Se investigó en plantas de Tabebuia el efecto en su crecimiento y nutrición de la inoculación con Rhizophagus intraradices, Azospirillum brasilense y Pseudomonas fluorescens. El hongo y las bacterias se aplicaron a las semillas en combinaciones de dos o tres de los siguientes microorganismos: A. brasilense 9 x 106 UFC g de turba-1, R. intraradices con 40 esporas g de suelo estéril-1 y raíces colonizadas al 95 % y P. fluorescens con 9 x 106 UFC g de turba-1 más el testigo. Se sembraron en macetas con una mezcla no estéril de suelo Andosol mólico y arena de río lavada, 1:1(v/v). En total se establecieron cinco tratamientos, con cinco repeticiones distribuidas en un diseño completamente al azar. Se realizaron cinco muestreos destructivos: el primero a los 45 días después de la siembra y los siguientes cada 28 días. Se registraron variables morfológicas y fisiológicas del rendimiento. Los tratamientos incrementaron el crecimiento de las plantas, con excepción de las dos bacterias juntas después de 158 días. El correspondiente a P. fluorescens - R. intraradices y el de los tres microorganismos indujeron más biomasa en el mayor número de componentes morfológicos y fisiológicos del rendimiento. El contenido más alto de N y P en el tejido vegetal se obtuvo con los tres microorganismos y con la combinación R. intraradices - A. brasilense.
Palabras clave: Azospirillum brasilense Tarrand; Krieg & Döbereiner; biomasa aérea; biomasa radical; inoculación; Pseudomonas fluorescens Migula; Rhizophagus intraradices (Schenck & Sm.) Walker & Schuessler
Introduction
The interaction between the elements of a microbe community in the agroforestry systems may or may not become apparent, in some morphological or physiological feature of the host plant of anthropocentric interest, particularly, in sustainable productivity systems or of low income, but its effective incidence depends on the microorganisms, of the environmental and soil conditions (Qiu et al., 2008). Some bacteria and fungi live in the section of the ground influenced by the roots, they stimulate growth of vegetals and induce positive effects in their development and survival (Artursson et al., 2006). For example, by promoting rhizobacteria of vegetal growth and the arbuscular mycorrhizal fungi (MA) that represent two of the most important groups of beneficiary microorganisms of the rhizosphere.
Inoculation with more than one microorganism to the seed or to the soil can make available to the host plant various mechanisms of action to supply nutrients and water. Several studies have shown that inoculation with fungi and bacteria produces a synergistic effect (Diouf et al., 2005; Lalitha et al., 2011), but in other cases it does not necessarily induce an additive or synergistic effect on the host plant (Trabelsi and Mhamdi, 2013).
In Mexico, increases in yield and their components in annual crops have been documented when inoculating fungi (MA) and nitrogen fixing bacteria under field conditions (Aguirre-Medina, 2006). In perennial crops such as Theobroma cacao L. in nursery, Aguirre-Medina et al. (2007) cite greater plant development when R. intraradices (Schenck & Sm.) Walker & Schuessler and A. brasilense Tarrand, Krieg & Döbereiner were incorporated together. In Coffea canephora (Pierre) ex Froehner, leaf number and stem biomass were superior when used separately (Aguirre-Medina et al., 2011).
Microorganisms induce increments in growth in shorter time in plants grown at the nursery, and, in consequence, diminish the necessary lapse before they are transplanted to the field. It is pretended to study this advantage on Tabebuia donnell-smithii Rose (Primavera), a deciduous tree from tropical and subtropical regions of Mexico (Miranda, 1998), that has a highly demanded timber, which has turned into a reduction of its natural abundance, from a greater harvesting and a rather unsuccessful regeneration.
The reforestation programs in Mexico show a survival under 50 %, partly from a poor quality of the plants grown at the nursery and the interrupting drought that occurs every year (Semarnat, 2010). In such conditions, it is possible that microorganisms help in plant survival when adverse environmental conditions prevail (Andrade et al., 2009).
Fungi (MA) increase nutrient absorption, phosphorous in particular (Richardson et al., 2009), and they induce tolerance to water stress (Sylvia, 2005). Azospirillum stimulates root development of the host plant, through hormones and nitrogen fixing (Bashan and De Bashan, 2010); and P. fluorescens Migula stimulates plant growth and makes minerals, soluble (Lucy et al., 2004).
In this context, the aim of the actual study was to determine the allocation of dry matter of Primavera interacting with the inoculation of Rhizophagus intraradices, Pseudomonas fluorescens and Azospirillum brasilense, and its effect over the nitrogen and phosphorous concentration in vegetal tissue.
Materials and Methods
Location and environment of the experimental site
The actual research took place (February to November 2013) at a nursery of the Experimental Station Rosario Izapa which belongs to the Instituto Nacional de Investigaciones Forestales, Agrícolas y Pecuarias (INIFAP), located at 18 km of the Tapachula-Cacaohatan highway in the municipality of Tuxtla Chico, Chiapas State between 14° 40’ N and 92° 10’ W at 435 masl. In this place the seeds of T. donnell-smithii seeds were collected which came from a dense crown, straight stem and without any signs of plagues or disease. The weather is humid warm in summer and with a monzoon influence. The average precipitation is 4 720 mm a year and the mean temperature, isotermal, of 25.4 °C.
Substrate physical and chemical analysis
The substrate was formed with a mixture of Molic andosol soil and washed river sand, 1: 1 (V / V), whose characteristics were sand-shallow texture(Bouyucos) (80.66 % sand, 16.36 % silt, 2.98 % clay), porosity (34 %), real density (2.09 g mL-1); bulk density (1.38 g mL-1); 3.1 % organic matter (Walkley-Black); 0.12 ds.m-1, electrical conductivity; pH 5.71; 0.19 % N (Kjelddhal); 5.0 ppm P (colorimetry); 18.5 ppm K++ (atomic spectrophotometry); 59 ppm Ca++ (atomic spectrophotometry); 8.1 ppm Mg++; and 5 Meq.100 g-1 cation exchange capacity. Black plastic bags (700 gauge) were filled with the non-sterile substrate, with a capacity of 5 kg of soil per bag, and were drilled in the lower part to favor drainage.
Microoganisms and inoculation
The microorganisms that were used were Rhizophagus intraradices produced in the Rosario Izapa Experimental Station of INIFAP. The inoculum contained 40 spores per gram of sterile soil and Brachiaria decumbens L. roots with 95 % of colonization. Azospirillum brasilense was purchased at the Benemérita Universidad Autónoma de Puebla with 9 x 106 bacteria g of peat-1. Pseudomonas fluorescens Migula, was provided by Celaya Experimental Station of INIFAP, with 9 x 106 bacteria g of peat-1. Seeds were germinated in trays with the same substrate and were transplanted to pots with two real leaves. At the time of transplantation, in the sewing hole were applied 2 g of inoculum mixed according to the treatment.
The five treatments are described as follows: 1) Azospirillum brasilense + Pseudomonas fluorescens; 2) Rhizophagus intraradices + P. fluorescens; 3) R. intraradices + A. brasilense; 4) A. brasilense + R. intraradices + P. fluorescens; and 5) Control. They were used with five plants as replications in a completely at random design. The experimental unit was a pot with one plant. Watering was made with distilled water.
Variables in record
Morphological and physiological yield variables were recorded 28 days after sowing (dds); from the first sampling to 46 dds, and the following to 74, 102, 130 and 158 dds. Root colonization and N and P content in plant tissue were recorded at the end of the study.
The aerial and root dry matter of the plant was determined in a forced air oven for 72 h at 75-80 °C (Sheldon, VWR 1390FM, USA) and the performance components were weighed on a semianalitical scale (Ohaus, Adventurer Pro).
The leaf area (cm2) was obtained with a leaf area integrator (LI-COR, LI 3000A, USA).
By the staining and polishing technique of Phillips and Hayman (1970), 100 root segments with a length of 1.5-1.6 cm were prepared from each sample and were observed under optical microscope with immersion objective (100 X). The results were expressed as percentage of mycorrhizal colonization.
Finally, the contents of N (Micro-Kjeldahl) and P were quantified in a spectrophotometer (Thermo Fisher Scientific, model 400 ¼).
Statistical analysis
The effects between treatments were estimated with an analysis of variance for the morphological, physiological variables, the nitrogen and phosphorus content with the PROC ANOVA procedure. Subsequently, a comparison of means (Tukey, P ≤ 0.05) was done with the Statistical Analysis System, version 8.1 (SAS, 1999-2000).
Results and Discussion
Table 1 shows the results of the different treatments. The R. intraradices, A. brasilense and P. fluorescens combined inoculation significantly increased all the growth parameters.
Table 1 Height, foliar area and dry weight of root and stem of Tabebuia donnell-smithii Rose plants.
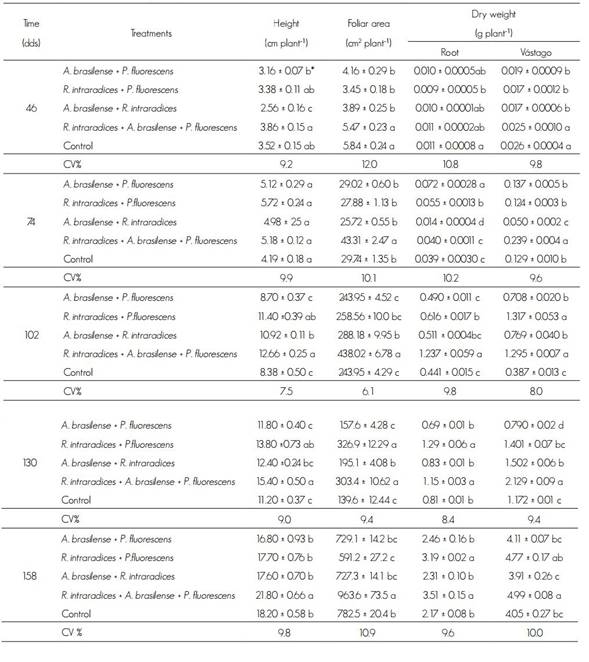
dds = Days after sowing; CV = Coefficient of variation (%).
* Values with different letter within each factor and column are statistically different (p ≤ 0.05).
Plants had slow growth the first 45 dds and, consequently, reached low height. At 28 days, an increase in all treatments was observed. This dynamic was repeated in the following samplings. The significant statistical differences (P ≤ 0.05) corresponded to the 46, 102, 130 and 158 dds. Between 46 and 74 dds, treatments with the microorganisms presented higher plant height compared to the control, and the average values varied from 1.32 cm, with inoculation of the three microorganisms, to 2.42 cm in the plants treated with R. intraradices and A. brasilense.
In all cases, the next evaluation period, at 102 dds, there was an increase in plant height: 4.0 at 7.0 cm; the highest height was obtained with the inoculation of the three microorganisms and the lowest with the two bacteria A. brasilense and P. fluorescens and the control. After the third sampling and until the end of the evaluation, differences in height were greater than 2 cm in treatments with microorganisms, compared to the control.
In other perennial species such as Theobroma cacao L., Aguirre-Medina et al. (2007) indicate that at 120 dds there are average differences in plant height of 9 cm in those inoculated with R. intraradices, in relation to the control. Karthikeyan et al. (2009) cite increase in plant height by inoculating the seeds of Catharanthus roseus (L.) G. Don. with A. brasilense and P. fluorescens; however, in the present study it was the combination with lower induction of plant height.
In wheat, Naiman et al. (2009) show different effects on the use of carbon sources when Azospirillum and Pseudomonas are inoculated and indicate their effect on growth. Zambrano and Díaz (2008) cite that A. brasilense promotes greater plant height in Gmelina arborea Roxb with Glomus manihotis Howeler, Sieverding & Schenk and Paraglomus occultum (Walker) Morton & Redecker. This background suggests the contrasting functionality of co-inoculations in interaction with plants (Jäderlund et al., 2008).
The leaf area evidenced growth values, statistically different (P ≤ 0.05) from the first sampling with the symbiosis of the three microorganisms. Only, at 130 dds of the A. brasilense + P. fluorescens bacteria it had a statistical difference (P ≤ 0.05). In other plants, such as cocoa and coffee with R. intraradices, there is also an increase in leaf area under nursery conditions (Aguirre-Medina et al., 2007; 2011). In this regard, Sylvia (2005) cites that plants after mycorrhizal colonization increase their photosynthetic activity, and what is expressed in more accumulation of biomass.
Dry root and stem weight
The highest biomass accumulated in the root system and the stem during the first sampling was recorded with the treatment of the three microorganisms and in the control were also statistically different (P ≤ 0.05) to the other treatments. The slow initial growth in plants inoculated with two microorganisms together is likely to be related to the increased demand for photosyntates by microorganisms during the establishment phase, even though with the three microorganisms the demand for carbon sources is greater and, consequently, initial growth is lower. It is possible that the combination of mechanisms of action between the three microorganisms depends on the functional compatibility in the physiology and biochemistry of the interaction between the microbial components (Vázquez et al., 2000).
At this stage, the availability of carbohydrates to the stem decreases, since most of the compounds are required by the root (Roveda and Polo, 2007). Wright et al. (2005) documented large concentrations of soluble carbohydrates in mycorrhizal roots of maize, adding that after the period of establishment of the symbiosis, normally initiates the mechanism of transport of nutrients to the plant and the concomitant increase in biomass, as happened in the following samplings with the inoculation of fungi and bacteria.
In the samples corresponding to 102, 130 and 158 dds, the largest growth in the root system occurred when inoculating the three microorganisms together. The combination of bacteria and fungi, P. fluorescens + R. intraradices improves root growth. P. fluorescens can stimulate root proliferation (Sánchez et al., 2005) through the production of indoleacetic acid (Patten and Glick, 2002), which is related to the promotion of plant growth (Gamalero et al., 2003). Co-inoculation with the two bacteria A. brasilense and P. fluorescens produced 30 % less radical biomass and 38 % in the control at the end of the study, compared to the treatment with the three microorganisms.
The dry weight of the stem was superior and statistically different (P ≤ 0.05) to the other treatments with the inoculation of the three microorganisms, throughout the evaluation. In two samples, at 102 and 158 dds, in the treatment with R. intraradices + P. fluorescens accumulation of biomass similar to that observed with the application of the three microorganisms was found, but without statistical difference between them (P ≤ 0.05). A similar behavior was observed during the first sampling (Table 1).
The co-inoculation with the two bacteria generated less biomass in root and stem in the samples at 74, 102 and 130 dds, compared to the treatments integrated by the bacteria in combination with R. intraradices. No colonization by the fungus (MA) at the beginning of the evaluation was obtained, but at 74, 102 and 130 dds, with an increase of around 20 %, at the end of the evaluation, the percentage was 26.
The mycorrhizal colonization might belong to the fungi in the substrate, whose presence is confirmed in addition, to the percentages of the control (without inoculation). The autoctonous fungi (MA) induced smaller vegetal development of T. donnelli-smithii and the greater vegetal response obtained from R. intraradices suggests a differential effect on the ability of fungi to stimulate plant growth, even when there is a possibility of having been colonized as well by autoctonous fungi (MA) (Figure 1).
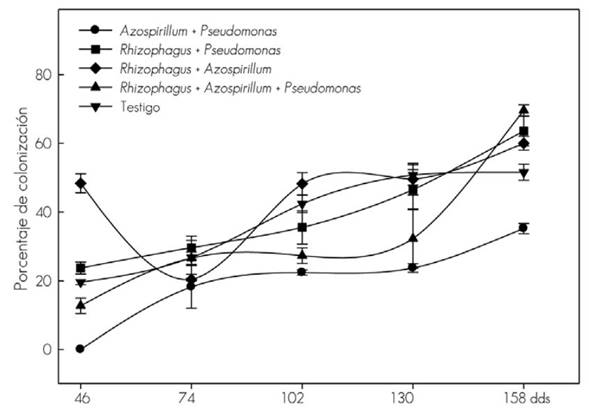
The vertical line indicates ± the standard error of five replications.
Figure 1 Mycorrhizal colonization in the root of Tabebuia donnell-smithii Rose plants.
The co-inoculation of R. intraradices + P. fluorescens led to a greater increase in dry biomass of root and stem, compared to the R. intraradices + A. brasilense combination. The effect was more evident from 74 and 158 dds, although mycorrhizal colonization was higher with the R. intraradices + A. brasilense combination during the whole evaluation period, when compared to the R. intraradices + P. fluorescens combination, in which there was little root colonization of the fungus (MA) at the beginning, but increased by about 60 % at the end of sampling.
The co-inoculation of A. brasilense and P. fluorescens bacteria responded differently with the same fungus (MA). This suggests some specificity between the bacteria and R. intraradices. Thus, coinoculation of the fungus with the specific bacterium may induce synergism in growth (Trabelsi and Mhamdi, 2013). With A. brazilense the initial colonization was the highest and with P. fluorescens, it was at the end of the study, and the same behavior was observed with the inoculation of the two bacteria together with the fungus (MA). In wheat the root growth is increased with Azospirillum and radical colonization is not affected when inoculated with G. mosseae (Nicolson & Gerd.) Gerd. & Trappe and G. macrocarpum Tul & Tul (Russo et al., 2005).
In treatments with R. intraradices, the increase in the development of the host plant may be due to the greater absorption of nutrients, through the fungus hypha. It is possible from the increase in the absorption capacity of mycorrhized plants (Leigh et al., 2009), due to a stimulatory effect on mycelial development, as stated by Barea et al. (1998) with Pseudomonas sp. F113 strain and G. mosseae; in addition to nitrogen fixation by bacteria (Hungria et al., 2004). Results confirm the ability of R. intraradices to colonize, and their effect on inducing higher host plant growth, in annual crops, as well as on different maize varieties in Africa (Wright et al., 2005); with Creole maize, varieties and hybrids in Mexico (Aguirre-Medina, 2006); and perennial crops like Coffea arabica L. (Aguirre-Medina et al., 2011), Coffea canephora Pierre ex A. Froehner (Ibarra-Puón et al., 2014) and Cedrela odorata L. (Aguirre-Medina et al., 2014).
Mycorrhizal colonization associated with bacteria does not seem to be related to the induction of plant development of Tabebuia donnell-smithii, since it varies according to the combination of the fungus with the bacterium. However, it is expected that mycorrhizal symbiosis presents changes in colonization levels (Sylvia, 2005) due to the interaction of various environmental and management factors (Andrade et al., 2009).
There is evidence that mycorrhiza interacts with a wide variety of organisms in the rhizosphere, such as diazotrophs; although the demand for carbohydrates increases with the co-inoculation of more than one microorganism (Lugtenberg et al., 1999), and it has been estimated that about 20 % of the total assimilated carbon by the plant is transferred to the fungus (Sylvia, 2005). In the study that is documented, the increase in the accumulation of biomass in the treatment with the three microorganisms together suggests the functional compatibility of them with the host plant, and that it supplied enough carbon to the microorganisms.
N and P content
In regard to nutrients, Figure 2 shows the contents in T. donnellsmithii at 140 dds.
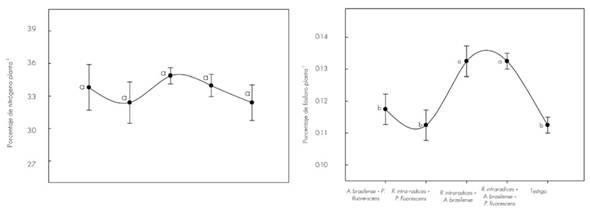
The vertical line indicates ± the standard error of five replicates. Different letters are statistically significant (P≤0.05). Coefficient of variation in nitrogen 6.4 % and in phosphorus 6.0.
Figure 2 Variation of nitrogen and phosphorus content in the plant tissue of Tabebuia donnell-smithii Rose by treatment at 140 days.
The nitrogen content in the stem of the plants inoculated with A. brasilense + P. fluorescens, R. intraradices + P. fluorescens; and the three microorganisms together were higher compared to the rest of the treatments, but with no statistical difference. This result can be related to competition (Neuhauser and Fargione, 2004) or incompatibility (Oyekanmi et al., 2007) among microorganisms, since its functional incidence has a primary effect on plant nutrition and, consequently, on the growth of the plant (Cano, 2011). With Glomus fasciculatum (Thaxt.) Gerd. And Trappe did not present increase of the nitrogen content in the plant tissue of Astronium graveolens Jacq., Tectona grandis L.f., Terminalia amazonia (Gmel.) Exell and Gmelina arborea Roxb., but it did in other nutrients (Hernández and Salas, 2009). In other investigations, inoculation of R. intraradices + A. brasilense increased the N content in the plant tissue of C. arabica (Aguirre-Medina et al., 2011).
The highest phosphorus content in plant tissue was recorded when R. intraradices was co-inoculated with A. brasilense and with the three microorganisms together. The concentrations were statistically different (P ≤ 0.05). Many studies have shown that mycorrhizal plants absorb P from soil more efficiently than non-colonized plants (Andrade et al., 2009). In Eleusine coracana Gaertn inoculated with Glomus fasciculatum and A. brasilense, greater uptake of P is recorded compared to control plants (Geeta et al., 2013). It has been reported that fungal hyphae have a higher affinity for phosphate ion when their concentration is low in the solution (Tajini and Drevon, 2012), and in low input production systems, mycorrhizal activity is more effective (Grant et al., 2005), with emphasis on low fertility soils (Mudge, 2003).
Co-inoculation of P. fluorescens with R. intraradices did not lead to higher P content in plant tissue. It is likely that this effect is related to the functionality of the two microorganisms and their effect on the T. donnell-smithii plant.
Conclusions
The inoculation of the three microorganisms together increased the dry weight of the root and the stem of T. donnellsmithii along the whole assessment and at the end of it the co-inoculation of R. intraradices + P. fluorescens expressed itself in a similar biomass accumulation. The effect of the combinations of microorganisms in the asignation of dry matter became apparent after 74 dds, a time at which the root colonization of the arbuscular mycorrhizal fungi increased.
The coinoculation of the three microorganisms and the coinoculation R. intraradices and A. brasilense promoted a greater content of N and P in the vegetal tissue.
REFERENCES
Aguirre-Medina, J. F. 2006. Biofertilizantes microbianos: Experiencias agronómicas del programa nacional del INIFAP en México. Instituto Nacional de Investigaciones Forestales, Agrícolas y Pecuarias. Centro de Investigaciones Regionales Pacífico Sur-Campo Experimental Rosario Izapa. Rosario-Izapa, Chis., México. 201p. [ Links ]
Aguirre-Medina, J. F. , A. Mendoza-López, J. Cadena-Iñiguez y C. H. Avendaño Arrazate. 2007. Efecto de la biofertilización en vivero del cacao (Theobroma cacao) con Azospirillum brasilense Tarrand, Krieg & Döbereiner y Glomus intraradices Schenk & Smith. Interciencia 32(8): 541-546. [ Links ]
Aguirre-Medina, J. F. , D. M. Moroyoqui-Ovilla, A. Mendoza-López , J. Cadena Iñiguez, J., C. H. Avendaño-Arrazate y J. F. Aguirre-Cadena. 2011. Aplicación de A. brasilense y G. intraradices a Coffea arabica en vivero. Agronomía Mesoamericana 22 (1):71-80. [ Links ]
Aguirre-Medina, J. F., F. O. Mina-Briones, J. Cadena-Iñiguez, J. D. Dardón-Zunun, y D. A. Hernández-Sedas. 2014. Crecimiento de Cedrela odorata L. Biofertilizada con Rhizophagus intraradices y Azospirillum brasilense en vivero. Revista Chapingo Serie Ciencias Forestales y del Ambiente 20 (3):177-186. [ Links ]
Andrade, S. A. L., P. Mazzafera, M. A. Schiavinato and A. P. D. Silveira. 2009. Arbuscular mycorrhizal association in coffee. Journal of Agricultural Science 147(2): 105-115. [ Links ]
Artursson, V., R. D. Finlay and J. K. Jansson. 2006. Interactions between arbuscular mycorrhizal fungi and bacteria and their potential for stimulating plant growth. Environmental Microbiology 8(1):1-10. [ Links ]
Barea, J. M., G. Andrade, V. Bianciotto, D. Dowling, S. Lohrke, P. Bonfante, F. O’gara, F. and C. Azcon-Aguilar. 1998. Impact on arbuscular mycorrhiza formation of Pseudomonas strains used as inoculants for biocontrol of soil-borne fungal plant pathogens. Applied Environmental Microbiology 64(6):2304-2307. [ Links ]
Bashan, Y. and L. E. De-Bashan. 2010. How the Plant Growth-Promoting Bacterium Azospirillum Promotes Plant Growth-A Critical Assessment. Advances in Agronomy 108:77-136. [ Links ]
Cano, A. M. 2011. Interacción de microorganismos benéficos en plantas: micorrizas, Trichoderma spp. y Pseudomonas spp. Una revisión. Revista UDCA Actualidad & Divulgación Científica 14(2):15-31. [ Links ]
Diouf, D., R. Duponnois, A. T. Ba, M. Neyra and D. Lesueur. 2005. Symbiosis of Acacia auriculiformis and Acacia mangium with mycorrhizal fungi and Bradyrhizobium spp. improves salt tolerance in greenhouse conditions. Functional Plant Biology 32 (12):1143-1152. [ Links ]
Gamalero, E., L. Fracchia, M. Cavaletto, J. Garbaye, P. Frey-Klett, G. C. Varese and M. G. Martinotti. 2003. Characterization of functional traits of two fluorescent pseudomonads isolated from basidiomes of ectomycorrhizal fungi. Soil Biology Biochemistry 35 (1):55-65. [ Links ]
Geeta, B. Patil, H. C. Lakshman, Romana M. Mirdhe and B. S. Agadi. 2013. Effect of co-inoculation of AM fungi and two beneficial microorganisms on growth and nutrient uptake of Eleusine coracana Gaertn. (Finger millet). Asian Journal of Plant Science and Research 3(1):26-30. [ Links ]
Grant, C., S. Bittman, M., Montreal, C. Plenchette and C. Morel. 2005. Soil and fertilizer phosphorus: Effects on plant P supply and mycorrhizal development. Canadian Journal of Plant Science 85(1):3-14. [ Links ]
Hernández, W. y E. Salas. 2009. La Inoculación con Glomus fasciculatum en el crecimiento de cuatro especies forestales en vivero y campo. Agronomía Costarricense 33(1):17-30. [ Links ]
Hungria, M., R. J. Campo, E. M. Souza y F. O. Pedrosa. 2004. Inoculation with selected strains of Azospirillum brasilense and A. lipoferum improves yields of maize and wheat in Brazil. Plant Soil 331(1-2):413-425. [ Links ]
Ibarra-Puón, J. C., J. F. Aguirre-Medina, A. Ley-De Coss, J. Cadena-Iñiguez y A. Zavala-Mata. 2014. Inoculación de Coffea canephora (Pierre) ex Froehner con Rhizophagus intraradices (Schenck et Sm.) Walker et Schuessler y Azospirillum brasilense Tarrand, Krieg et Döbereiner en vivero. Revista Chapingo Serie Horticultura 20(2): 201-213. [ Links ]
Jäderlund, L., V. Arthurson, U. Granhall and J. K. Jansson . 2008. Specific interactions between arbuscular mycorrhizal fungi and plant growth-promoting bacteria: as revealed by different combinations. FEMS Microbiology Letters 287(2):174-180. [ Links ]
Karthikeyan, B., A. J. Cheruth and M. M. Azooz. 2009. Individual and Combined Effects of Azospirillum brasilense and Pseudomonas fluorescens on Biomass Yield and Ajmalicine Production in Catharanthus roseus. Academic Journal of Plant Science 2 (2):69-73. [ Links ]
Lalitha, S., K. Rajeshwaran, P. Senthil Kumar and S. Deepa. 2011. Role of AM fungi and rhizobial inoculation for reclamation of phosphorus deficient soil. Asian Journal of Plant Science 10(3): 227-232. [ Links ]
Leigh, J., A. Hodge and A. H. Fitter. 2009. Arbuscular mycorrhizal fungi can transfer substantial amounts of nitrogen to their host plant from organic material. New Phytologist 181(1):199-207. [ Links ]
Lucy, M., E. Reed and B. R. Glick. 2004. Application of free living plant growth promoting rhizobacteria. Antonie Van Leeuwenhoek 86(1):1-25. [ Links ]
Lugtenberg, B. J. J., L. V. Kravchenko and M. Simons. 1999 Tomato seed and root exudate sugars: composition, utilization by Pseudomonas biocontrol strains and role in rhizosphere colonization. Environmental Microbiology 1:439-446. [ Links ]
Miranda, F. 1998. La Vegetación de Chiapas. Edición Gobierno del Estado de Chiapas. Tuxta Gutiérrez, Chis., México. 600 p. [ Links ]
Mudge, S. R., F. W. Smith and A. E. Richardson. 2003. Root-specific and phosphate-regulated expression of phytase under the control of a phosphate transporter promoter enables Arabidopsis to grow on phytate as a sole P source. Plant Science 165(4):871-878. [ Links ]
Naiman, A. D., A. Latronico and I. E. Garcia de Salamone. 2009. Inoculation of wheat with Azospirillum brasilense and Pseudomonas fluorescens: impact on the production and culturable rhizosphere microflora European Journal of Soil Biology 45(1):44-51. [ Links ]
Neuhauser, C. and J. E. Fargione, 2004. A mutualism-parasitism continuum model and its application to plant-mycorrhizae interactions. Ecological Modelling 177: 337-352. [ Links ]
Oyekanmi, E. O., D. L. Coyne, O. E. Fagade and O. Osonubi. 2007. Improving root-knot nematode management on two soybean genotypes through the application of Bradyrhizobium japonicum, Trichoderma pseudokoningii and Glomus mosseae in full factorial combinations. Crop Protection 26:1006-1012. [ Links ]
Patten, C. L. andB. R. Glick. 2002. Role of Pseudomonas putida indoleacetic acid in development of the host plant root system. Applied Environmental Microbiology 68(8):3795-3801. [ Links ]
Phillips, J. M. and D. S. Hayman. 1970. Improved procedures for clearing and staining parasitic and vesicular-arbuscular mycorrhizal fungi for rapid assessment of infection. The British Mycology Society 55(1): 58-161. [ Links ]
Qiu, M. Q, H. Zhang, G. X. Wang and Z. Q. Liu. 2008. Effects of nitrogen on plant-microorganism interaction. EurAsian Journal of BioScience 2:34-42. [ Links ]
Richardson, A. E., J. M. Barea, A. M. Mc Neill and C. Prigent-Combaret. 2009. Acquisition of phosphorus and nitrogen in the rhizosphere and plant growth promotion by microorganisms. Plant Soil 321: 305-339. [ Links ]
Roveda, R. y C. Polo. 2007. Mecanismos de adaptación de maíz asociado a Rhizophagus spp. en suelos con bajo fósforo disponible. Agronomía Colombiana 25(2): 349-356. [ Links ]
Russo, A., M. Götz, C. Felici, C. Collados, Y. Moënne-Loccoz, J. Vanderleyden, A. Toffanin, J. M. Barea , K. Smalla and M. Nuti. 2005. Effect of Azospirillum inoculants on arbuscular mycorrhiza establishment in wheat and maize plants. Biology Fertility Soils 41(5):301-309. [ Links ]
Sánchez, L., S. Weidmann, C. Arnould, A. R. Bernard, S. Gianinazzi and V. Gianinazzi-Pearson. 2005. Pseudomonas fluorescens and Glomus mosseae Trigger DMI3-Dependent Activation of Genes Related to a Signal Transduction Pathway in Roots of Medicago truncatula. Plant Physiology 139(2):1065-1077. [ Links ]
Secretaria de medio ambiente y recursos naturales (Semarnat). 2010., Informe de evaluación externa de los apoyos de reforestación, ejercicio fiscal 2009. Comisión Forestal. México, D.F., México. 140p. [ Links ]
Statistical Analysis System (SAS). 1999-2000. SAS/STAT User´s Guide. Ver 8.1 SAS Institute Inc. Cary, NC, USA. n/p. [ Links ]
Sylvia, M. D. Mycorrhizal symbioses. 2005. In: Sylvia, M. D. , J. J. Fuhrmann, G. P. Harte and A. D. Zuberer. (ed.). Principles and Applications of Soil Microbiology. Second Edition. Upper Saddle River, NJ, USA. Pearson Prentice Hall. pp. 263-282. [ Links ]
Tajini, F. and J. J. Drevon. 2012. Phosphorus use efficiency in common bean (Phaseolus vulgaris L.) as related to compatibility of association among arbuscular mycorrhizal fungi and rhizobia. African Journal of Biotechnology 11(58):12173-12182. [ Links ]
Trabelsi, D. and R. Mhamdi. 2013. Microbial Inoculants and Their Impact on Soil Microbial Communities: A Review. BioMed Research International. doi:10.1155/2013/863240. [ Links ]
Vázquez, M. M., S. César, R. Azcón andJ. M. Barea . 2000. Interactions between arbuscular mycorrhizal fungi and other microbial inoculants (Azospirillum, Pseudomonas, Trichoderma) and their effects on microbial population and enzyme activities in the rhizosphere of maize plants. Applied Soil Ecology 15:261-272. [ Links ]
Wright, D. P., J. D. Scholes, D. J. Read and S. A. Rolfe. 2005. European and African maize cultivars differ in their physiological and molecular responses to mycorrhizal infection. New Phytolist 167(3):881-896. [ Links ]
Zambrano, J. A. y L. A. Díaz. 2008. Efecto de la inoculación de Azospirillum brasilense y Glomus sp. en Gmelina arborea durante su germinación y manejo en vivero. Universitas Scientiarum 13(2):162-170. [ Links ]
b Contribution by autor: Juan Francisco Aguirre Medina: research planning and desing, data sampling, sample handling for vegetal analysis, root colonization, structuring and review of the manuscript; Juan Francisco Aguirre Cadena: experiment control and susbstrate preparation, bag filling, watering and sampling; Miguel Antonio Ramón Castro: seed collection, and establishment of the experiment.