Introduction
Forests cover about 30 % of the Earth's land surface, totaling almost 4 billion hectares (Food and Agriculture Organization of the United Nations [FAO], 2016). Between 1990 and 2015, 129 million hectares of forests were lost globally, which is equivalent to an approximate loss of 11 Pg (11 billion tons) of carbon stocks through deforestation or forest degradation (FAO, 2016). The loss and disturbance of temperate and tropical forests is the second largest source of direct CO2 emissions to the atmosphere (Intergovernmental Panel on Climate Change [IPCC], 2014).
In 2008, the Reducing Emissions from Deforestation and Forest Degradation Plus (REDD+) initiative was launched as one of the global strategies to mitigate climate change. This initiative aims to reduce greenhouse gas (GHG) emissions through the conservation and sustainable management of forests in developing countries (Food and Agriculture Organization of the United Nations, United Nations Development Programme, & United Nations Environment Programme [FAO, UNDP, & UNEP], 2015). REDD+ seeks to consolidate itself as a form of environmental governance for sustainable development that contributes to alleviating rural poverty and inequality, as well as guaranteeing the conservation of biodiversity (Corbera & Schroeder, 2011). In this way, each nation is responsible for directing its own program according to its particular conditions, but with the informed and active involvement of relevant social actors, including indigenous groups and rural communities dependent on forests (FAO, UNDP, & UNEP, 2015).
In developing countries, about 25 % of forests are under community ownership or management schemes (Bluffstone, Robinson, & Guthiga, 2013), so it is paramount to include local communities in the design and implementation of any public policy that affects their territories. Community participation in carbon measurement and monitoring makes it possible to physically measure forest degradation, biomass density and the annual increase in carbon stocks, variables that are difficult to measure reliably using remote sensing technologies (Skutsch & McCall, 2011). It also allows recording social and other kinds of environmental data simultaneously, observing changes in community territories in real time and explaining in detail the causes of carbon stock losses. In addition, the appropriation of measurement and data collection techniques by communities can improve their management capacity and strengthen the owners´ sense of territory (Butt, Epps, Overman, Iwamura, & Fragoso, 2015; Larrazábal, McCall, Mwampamba, & Skutsch, 2012; Sharma, Deml, Dangal, Rana, & Madigan, 2015). Communities can and should measure changes in their carbon stocks as a strategy for more effective, efficient and integrated forest management (Agrawal & Angelsen, 2009; Skutsch & McCall, 2011). In the case of forest carbon measurement and monitoring, global studies have reported that participatory methods yield valid, robust and reliable data (Hawthorne, Boissière, Felker, & Atmadja, 2016; Larrazábal et al., 2012).
It has been shown that communities that monitor their forests also manage them better (Gibson, Williams, & Ostrom, 2005). In Mexico, 55 % of forest lands are under communal ownership schemes (Madrid, Nuñez, Quiroz, & Rodríguez, 2009). Although the national REDD+ strategy (ENAREDD+) does not consider community participation as part of its measurement, reporting and verification (MRV) system, it does include the development of skills in communities to carry out better forest management practices among its objectives (Comisión Nacional Forestal [CONAFOR], 2014; Ellis, Rodriguez-Ward, Romero-Montero, & Hernández, 2015). Moreover, if communities are able to increase their carbon stocks instead of just reducing their losses, they would have the possibility to participate in voluntary carbon markets, external to government programs, and sell the credits for the certified increases (Balderas & Skutsch, 2012).
The objectives of this study were to estimate carbon stocks in communally owned forests through participatory mapping and measurement methods, and to identify land uses, priority landscape elements and forest conflicts that may influence the maintenance or enhancement of carbon stocks. The study was conducted in an international conservation priority area: the Monarch Butterfly Biosphere Reserve (United Nations Educational, Scientific and Cultural Organization [UNESCO], 2008), which is part of the upper Cutzamala River basin, and which is one of the priority REDD+ regions in Mexico (Ellis et al., 2015), given its importance in providing water to the country's most densely populated region.
Materials and methods
Study area
The Monarch Butterfly Biosphere Reserve (MBBR) has plant associations of oak (Quercus sp.), pine (Pinus sp.), sacred fir (Abies religiosa [Kunth] Schltdl. & Cham.) and mixed-species forests (Giménez, Ramírez, & Pinto, 2003), associated with its elevational gradient of between 2,400 and 3,620 m. Sacred fir forests are the habitat where colonies of monarch butterflies (Danaus plexippus L.) overwinter during November-March in Mexico (UNESCO, 2008). For more than 10 centuries, these forests have been used by the local population, as settlements dependent on the use of natural resources have been established around them. Early evidence of this process is the pre-Hispanic ruin of Ziráhuato (650-1500 AD.; Guzmán, 2012) in the municipality of Zitácuaro, as well as the ruins of several agricultural, forestry and mining haciendas of the colonial era that can be seen in the surroundings of the reserve.
The MBBR covers 56 259 ha divided into three core zones (13 554 ha) and two buffer zones (42 705 ha). By law, only environmental research and education activities that do not alter the habitat can be carried out in the core zones. In the buffer zones, controlled sustainable production activities such as ecotourism, forest management, and agricultural production are permitted (Secretaría de Medio Ambiente, Recursos Naturales y Pesca [SEMARNAP], 2000). Throughout the 20th century, most of the territory that currently makes up the MBBR was granted to agrarian nuclei in the form of communal property (ejidos and indigenous communities). The reserve also includes some private properties, a federal property and a state one. In the two forms of communal land tenure, individuals who hold the title of ejidatario or communero make decisions about the management of common resources of the agrarian nuclei (Cámara de Diputados del H. Congreso de la Unión, 1992).
Four agrarian nuclei of the municipality of Zitácuaro participated in this work: the indigenous communities of Carpinteros, Donaciano Ojeda and Francisco Serrato, and the ejido San Juan Zitácuaro. All have forest lands partially within the MBBR and cover a total area of 6,563 ha (Figure 1). Each agrarian nucleus shows different levels of anthropic pressure on the forest (Table 1), which is reflected in the degree of conservation.
Table 1 Type of land tenure, anthropic pressure and land cover by agrarian nuclei in the Monarch Butterfly Biosphere Reserve (MBBR).
Characteristics | Carpinteros | Donaciano Ojeda | Francisco Serrato | San Juan Zitácuaro |
---|---|---|---|---|
1Type of land tenure | Indigenous community | Indigenous community | Indigenous community | Ejido |
1Total area (ha) | 857 | 2 597 | 1 841 | 1 268 |
1Number of ejidatarios/comuneros | 218 | 666 | 234 | 144 |
2Number of inhabitants | 860 | 2 264 | 2 561 | 1 922 |
3Forest area (ha) by ejidatario/comunero | 2.8 | 2.5 | 4.3 | 6.2 |
3Forest area (ha) per inhabitant | 0.7 | 0.7 | 0.4 | 0.5 |
4Period with forest harvesting permit | - | 1996-1999 | 1995-1997 | 1995-2016 |
3Loss of dense forest 1993-2015 (ha) | - | -45 | -142 | -27 |
3Forests 2015 (%) | 71 | 65 | 55 | 70 |
3Crops, pastures and secondary shrubs (%) | 9 | 22 | 42 | 7 |
3Fruit plantations (%) | 20 | 13 | 3 | 20 |
3Urban nuclei (%) | 0 | 0 | 0 | 3 |
Source: 1Padrón e Historial de Núcleos Agrarios (PHINA, 2017); for the San Juan Zitácuaro ejido, the data were estimated in the field, since it does not have official PROCEDE (Ejidal Rights Certification Program) certification. 22010 Population and Housing Census (Instituto Nacional de Estadística y Geografía [INEGI], 2017). 3Author-made calculations, from the 2015 update of Navarrete, Ramírez, and Pérez-Salicrup (2011). 4Data provided by the Michoacán Delegation of SEMARNAT (Secretariat of the Environment and Natural Resources).
Participatory mapping of forest condition
Participatory mapping workshops were held, one per community, with the participation of the relevant authorities and inhabitants interested in forest activities. Each workshop included talks on the project and the importance of the ecosystem carbon sequestration service. Foresters from Alternare A. C. and geographers, ecologists and environmentalists from UNAM-Campus Morelia collaborated in the workshops as well as in the other project tasks.
High-resolution satellite images obtained from Google Earth Pro (2014) were printed to create 120 x 70 cm photomaps (scale between 1:5000 and 1:10000, depending on the size of each agrarian nucleus), where the conditions of tree density and forest fragmentation could be observed. The boundary of the agrarian nucleus was added to each photomap.
Three teams were formed per community; each team included five to 10 people who knew the forest very well. On the photomaps, the participants of each community were in charge of indicating the forest conditions of their property, such as: dominant species, management activities and the conservation status of the forest (dense forests without disturbances in the last three decades or open forests resulting from extractions or other disturbances). Participants also identified any uses, events or resources related to their forestry and agricultural activities that they considered relevant. The maps of the three teams were integrated into a single map for each agrarian nucleus, to identify the common elements pointed out and thus reduce the inherent subjectivity of this mapping method.
Selection of sampling sites
Field sampling was done based on procedures described by Skutsch and Benet (2014). The technical team calculated the number of sites (n) to be sampled using the following formula:
where,
CV = coefficient of variation of the basal area (%)
Z = confidence level (90 %)
E = margin of error (10 %).
To calculate the CV, preliminary sampling was applied in different forest types and conditions in the study area. The number of resulting points (24) was distributed in equal parts among the four agrarian nuclei. The technical team proposed the distribution of the sites based on the results of the participatory mapping, the elevational gradients represented by the plant formations (Giménez et al., 2003) and the land cover as reported in Champó-Jiménez, Valderrama-Landeros, and España-Boquera (2012), López-García and Vega (2010) and Ramírez, Miranda, Zubieta, and Jiménez (2007).
The proposal was reviewed by community authorities, who made adjustments to ensure accessibility and sampling in sites of particular interest, such as areas at risk or affected by illegal logging, and areas with reforestation programs or where they have favored natural regeneration. In the end, 31 sites were agreed upon, seven or eight per agrarian nucleus, covering the plant formations and states of conservation present in each community.
Biomass measurement brigades
The community authorities assigned brigades of three or four ejido or community members to locate the sampling sites and conduct the tree parameter measurements. Individuals in the brigades were all members of the community surveillance committees or forest brigades and were knowledgeable about the forests and their territory. A second round of on-site workshops, one day per community, was conducted to instruct the brigade members on the delimitation, distribution and size of the sampling plots and sub-plots, as well as how to measure diameter at breast height (DBH) and tree height (Skutsch & Benet, 2014). Diameter and surveying tapes, compasses, clinometers and flagging tapes were provided for this purpose. Participants also learned the basic functions of GPS (Garmin GPSMAP® 60C) for the identification of the central coordinates and elevation of the sampling sites.
Field sampling
A cluster sampling method involving four circular plots per site was applied (CONAFOR, 2012) (Figure 2). Each plot was formed by two concentric circles with a 12.5 m and 2 m radius, equivalent to 491 m2 and 12 m2, respectively. In the first, trees with DBH > 7.5 cm were recorded and in the second, all regeneration individuals >25 cm in height and DBH ≤ 7.5 cm (Skutsch & Benet, 2014). First, the central tree was marked and the coordinates of the center of the plot were taken. From this tree the tape measure was stretched towards magnetic north along the 12.5 m radius; it was turned clockwise until it covered the entire plot. Each tree on this radius was marked and recorded in a format indicating the species and DBH measurement, as well as the distance from a point that allowed seeing the base and the crown, to obtain the inclination angles. This part of the work was carried out by the brigade members under the supervision of Alternare's technical team.

Figure 2 Conglomerate sampling design of four plots per site, for the measurement of tree-size parameters.
Using pre-prepared forms, the brigade members wrote down the common name of each tree and compared them with a MBBR vegetation guide (Cornejo-Tenorio & Ibarra-Manriquez, 2008). In cases of doubt, the trees were recorded with a consecutive identifier and a sample was collected for identification by UNAM Campus-Morelia specialists.
Sampling was done in five days per community, with a survey of between four and eight plots per day (one or two sampling sites), depending on the distance to the site, the number of trees per plot and the progress in the sampling area. In this process, the team of academics systematically recorded the performance of each brigade with respect to three criteria: interest and initiative, organization, and mastery of materials and measurements.
Tree carbon estimation
With the data recorded by the local brigade members, as supervised by the Alternare A. C. technicians, aboveground biomass and tree carbon were calculated by team members from the university (UNAM Morelia). In these calculations, non-linear allometric equations available for the identified species were applied (Table 2), giving preference to those that had been generated for species in nearby regions and with similar climatic conditions, in accordance with the recommendations of the IPCC (2003). Most of the equations available for Mexican species only require the DBH to make the estimate (Rojas-García, de Jong, Martínez-Zurimendí, & Paz-Pellat, 2015); for the calculation of tree carbon, it was assumed to account for 50 % of the biomass (IPCC, 2003). In the case of broadleaf trees for which no specific allometric equation was available, we used the regional model for Quercus sp. proposed by Návar (2009), instead of using alternative general equations for pantropical species (Gibbs, Brown, Niles, & Foley, 2007).
Table 2 Allometric equations used to estimate the aboveground biomass of species present in the Monarch Butterfly Biosphere Reserve.
Species | Equation | Source |
---|---|---|
Abies religiosa | B = 0.0754 * (DBH^2.513) | Avendaño, Acosta, Carrillo, and Etchevers (2009) |
Ageratina mairetiana | B = 0.0890 * (DBH^2.5226) | Návar (2009) |
Arbutus sp. | . | . |
Buddleja cordata | . | . |
Comarostaphylis longifolia | . | . |
Cornus disciflora | . | . |
Crataegus mexicana | . | . |
Ilex brandegeana | . | . |
Populus sp. | . | . |
Quercus sp. | . | . |
Styrax argenteus | . | . |
Other broadleaves | . | . |
Alnus sp. | B = 0.1649 * (DBH^2.2755) | Acosta, Carrillo, and Gómez (2011) |
Clethra mexicana | B = 0.4632 * (DBH^1.8168) | Acosta et al. (2011) |
Cupressus lindleyi | B = 0.5266 * (DBH^1.7712) | Rojas-García et al. (2015) |
Pinus sp. | B = 0.1229 * (DBH^2.3964) | Návar (2009) |
Ternstroemia sylvatica | B = 0.132193 * (DBH^2.49568) | Rodríguez, Jiménez, Aguirre, Treviño, and Razo (2009) |
The aboveground biomass and carbon content were estimated per individual, plot and site. For comparative purposes, the value of the latter was extrapolated to values per hectare. Average values were calculated by potential vegetation type (Giménez et al., 2003) and by actual vegetation type, determined by the dominance (>75 %) of a group of species (coniferous or broadleaf) (Jardel, 2015).
Recognition of forest uses
During the information and participatory mapping workshops, the field data collection and the review of results (which involved a third round of one-day workshops per community), semi-structured interviews were conducted with participants, brigade members and authorities. General information was obtained on land use, impacts and community achievements in forest management; agreements for access to forest resources, violations of such agreements and corresponding sanctions, and the conflicts and environmental history of the property.
Results and discussion
Participatory mapping workshops provided general information about the forests of each community and their conservation status. The information obtained through participatory methods, in addition to showing phenomena and physical spatial objects, can also expose priority relationships and events for people who live and perceive those spaces (McCall, Skutsch, & Chutz, 2016; Norris, 2014). This can result in communities becoming more involved in the management of the territory and its resources, as well as in actions to address climate change (Pokorny et al., 2008; Sheppard et al., 2011).
In the participating communities, regulated uses were identified, as well as clandestine logging, fires, pests and landslides that altered the structure and dynamics of the forests. Recovery areas, where reforestation and natural regeneration stood out, were also pointed out. Other relevant aspects represented in the maps were conflicts over land ownership boundaries, as well as some important components of the forest, such as springs, firewood collection areas, sites with fauna of interest and sites for collecting non-timber products (Figure 3). This creates complex mosaics of varying degrees of conservation, structure and tree composition that, as in other parts of the country, are reflected in the range of carbon stock by vegetation type. In Mexico, specialized literature on temperate forests reports values between 60 and 155 Mg C·ha-1 for oak forests and mixed pine-oak forests; between 40 and 200 Mg C·ha-1 in pine forests; and between 80 and 180 Mg C·ha-1 in sacred fir forests (Álvarez & Rubio, 2013; De Jong, 2001; Návar, 2009; Ordóñez et al., 2008; Razo, Gordillo, Rodríguez, Mayacotte & Acevedo, 2013; Rodríguez et al., 2009).
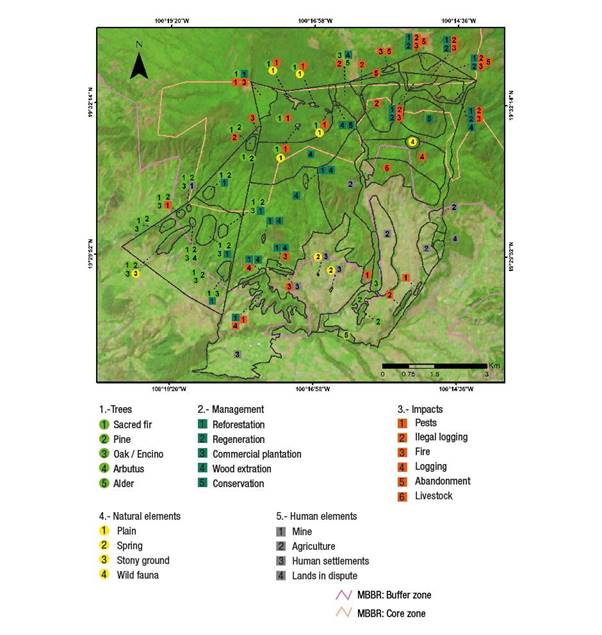
Figure 3 Example of the result of the integration of participatory mapping: indigenous communities of Carpinteros, Donaciano Ojeda and Francisco Serrato in the Monarch Butterfly Biosphere Reserve (MBBR).
Table 3 shows that, within the MBBR’s core zone, aboveground forest carbon estimates were highest at sampling sites dominated by conifers (A. religiosa and A. religiosa-Pinus sp.) with values between 51 and 210 Mg C·ha-1. In sites where broadleaf trees (Quercus sp. and Alnus sp. and other broadleaf species) predominate, between 40 and 154 Mg C·ha-1 were recorded, and in sites co-dominated by conifers and broadleaf trees, values between 15 and 157 Mg C·ha-1 were determined. These intervals are explained more by the degrees of conservation of the site than by the composition of the vegetation. Moreover, due to management differences, the dominant species in some sites do not correspond to the expected plant associations of the bioclimatic level in which they are found (Giménez et al., 2003).
Table 3 Tree carbon content by sampling site and vegetation type in the Monarch Butterfly Biosphere Reserve.
Site | Coordinates | Elevation (m) | Carbon (Mg·ha-1) | Dominant trees | Potential vegetation1 |
---|---|---|---|---|---|
Carpinteros indigenous community | |||||
La Cabaña | 19.530° N, 100.298° W | 3 121 | 51 | Conifers | Sacred fir |
Mesa Chica | 19.527° N, 100.301° W | 3 016 | 136 | Mixed | Sacred fir |
Debajo Mesa Chica | 19.523° N, 100.300° W | 2 898 | 136 | Mixed | Sacred fir-pine-broadleaf species |
Los Pozos | 19.515° N, 100.313° W | 2 615 | 109 | Mixed | Pine-oak-broadleaf species |
Cerca de Piedra | 19.503° N, 100.304° W | 2 492 | 92 | Mixed | Pine-oak-broadleaf species |
El Voladero | 19.505° N, 100.317° W | 2 480 | 78 | Mixed | Pine-oak-broadleaf species |
Magueyitos | 19.495° N, 100.306° W | 2 361 | 57 | Broadleaf | Pine-oak-broadleaf species |
El Espinal | 19.505° N, 100.327° W | 2 270 | 62 | Mixed | Pine-oak-broadleaf species |
Donaciano Ojeda indigenous community | |||||
El Cuartel | 19.539° N, 100.284° W | 3 001 | 210 | Conifers | Oyamel |
La Rosa | 19.534° N, 100.287° W | 2 979 | 174 | Conifers | Sacred fir-pine-broadleaf species |
Chivatí | 19.540° N, 100.297° W | 2 947 | 71 | Conifers | Sacred fir-pine-broadleaf species |
Piedra Encimada | 19.538° N, 100.271° W | 2 885 | 117 | Broadleaf | Sacred fir-pine-broadleaf species |
El Puerto | 19.511° N, 100.292° W | 2 647 | 125 | Broadleaf | Pine-oak-broadleaf species |
El Salto | 19.516° N, 100.273° W | 2 633 | 128 | Broadleaf | Pine-oak-broadleaf species |
Chisña | 19.499° N, 100.288° W | 2 384 | 98 | Broadleaf | Pine-oak-broadleaf species |
Francisco Serrato indigenous community | |||||
La Cebolleta | 19.543° N, 100.256° W | 3 064 | 78 | Mixed | Sacred fir |
Salistrillo | 19.541° N, 100.244° W | 3 048 | 26 | Mixed | Sacred fir |
Loma Caño Seco | 19.532° N, 100.264° W | 2 875 | 154 | Broadleaf | Sacred fir-pine-broadleaf species |
Loma del Gallinero | 19.535° N, 100.253° W | 2 874 | 141 | Broadleaf | Sacred fir-pine-broadleaf species |
Xoreje | 19.522° N, 100.254° W | 2 715 | 110 | Conifers | Sacred fir-pine-broadleaf species |
Madroños | 19.517° N, 100.258° W | 2 625 | 57 | Conifers | Pine-oak-broadleaf species |
Tercera Manzana | 19.487° N, 100.268° W | 2 387 | 32 | Mixed | Pine-oak-broadleaf species |
Parador ecoturístico | 19.481° N, 100.280° W | 2 232 | 103 | Mixed | Pine-oak-broadleaf species |
San Juan Zitácuaro ejido | |||||
Cima Cacique | 19.389° N, 100.316° W | 3 184 | 15 | Mixed | Sacred fir |
Intermedio | 19.381° N, 100.311° W | 2 840 | 79 | Broadleaf | Sacred fir-pine-broadleaf species |
Agua de la Difunta | 19.384° N, 100.307° W | 2 800 | 84 | Mixed | Sacred fir-pine-broadleaf species |
Caseta 2 | 19.430° N, 100.259° W | 2 751 | 157 | Mixed | Sacred fir-pine-broadleaf species |
Reforestación Cacique | 19.378° N, 100.302° W | 2 750 | 40 | Broadleaf | Sacred fir-pine-broadleaf species |
Mesa del León | 19.376° N, 100.317° W | 2 668 | 40 | Broadleaf | Sacred fir-pine-broadleaf species |
Caseta 1 | 19.442° N, 100.261° W | 2 575 | 157 | Mixed | Pine-oak-broadleaf species |
La Charca | 19.439° N, 100.273° W | 2 465 | 97 | Mixed | Pine-oak-broadleaf species |
1Dominant trees of the corresponding bioclimatic level, according to Giménez et al. (2003).
The reliability of measurements obtained by participatory methods is well documented (Butt et al., 2015; Larrazábal et al., 2012; Skutsch & McCall, 2011), as is the accuracy of the application of allometric models. It is estimated that DBH alone is sufficient to estimate 95 % of carbon stocks in tropical forests (Gibbs et al., 2007), particularly if local specific allometric equations are used (Álvarez et al., 2011; Vieilledent et al., 2012).
Comparison of the carbon stocks measured and values reported in the specialized literature allows inferring that these communities have potential for carbon sequestration and storage. The brigade members perceived this situation when they visited the sites and transmitted this idea to the authorities for consideration in developing forest management plans. It is important to recognize that the potential for carbon sequestration is not only a consequence of the ecological conservation status of the forest, but also of the existence of local institutions that are able to organize themselves to carry out forest activities (Barsimantov & Navia, 2012; Gibson et al., 2005).
Table 4 indicates that the Donaciano Ojeda indigenous community had the highest carbon content (132 ± 46 Mg C·ha-1). There, community members make a very significant investment in effort and resources to monitor their forest in an organized manner and prevent illegal extraction of trees by outsiders. They also make frequent rounds to monitor the conditions of their forest and identify, with precision, the sites where wood has recently been extracted. For its forest monitoring and care work, the community received an Honorable Mention in the Recognition of Nature Conservation awards in 2013.
In the Carpinteros indigenous community, the average carbon level in the sampled sites reaches 90 ± 34 Mg C·ha-1 (Table 4), which denotes the dominance of young forests, resulting from disturbances two to three decades ago. Since the declaration of the MBBR, the community has worked in an organized manner in the recovery of its trees through reforestation, forest monitoring and control of the agricultural frontier; it also identifies a close relationship between the state of its forests and the availability of water for human consumption and productive activities. In 2012, the community received the National Forest Merit Award in the category of Forest Restoration. This area exhibits an extended process of intensified agricultural land use, as they have replaced most of their plots used for growing corn and other self-consumption crops with avocado plantations for export, which show significant growth in the eastern region of the state. During the results review workshop, participants mentioned that the data from the tree carbon measurements are useful for the development of the forest harvesting plan and that the members of the measurement brigades are part of the community Forestry Committee.
In the Francisco Serrato indigenous community, the average tree carbon level of the sampled sites was 88 ± 48 Mg C·ha-1 (Table 4). Significant efforts have also been made there to monitor their forests; problems due to extraction by outsiders have decreased in recent years, although they have not completely disappeared. The community also faces internal conflicts where community agreements have not been respected by all, so there is forest deterioration caused even by the inhabitants themselves. During the trips to reach the sampling plots, evidence of tree extraction outside the community agreements was found and no sanctions were reported for the offenders.
In the San Juan Zitácuaro ejido, the average carbon level of the sampled sites was the lowest (84 ± 53 Mg C·ha-1; Table 4), although there are very contrasting conditions in the four fragments that constitute its property. In one, the recovery of about 350 ha of forest that was under agriculture for 45 years stands out (Figure 4). Based on a communal decision, the inhabitants opted to convert these areas to forest through a complex mixture of reforestation and natural regeneration, in order that they might later carry out authorized forest uses. This area is in the process of being certified by the Forest Stewardship Council (FSC). In 2011, the ejido won the National Forest Merit Award in the category of Forest Protection. Members of this community refer to the importance of caring for the forest to ensure the water supply to allow for agricultural intensification, and to change subsistence crops for avocado orchards of high commercial value. This, in turn, is seen as a source of funding for forest conservation activities. On the other hand, in the fragment furthest from the population nucleus, the forests with the lowest carbon content levels were found (Table 2); this area has no surveillance and is difficult to access, which prevents attending, in a timely manner, to natural disturbances (fires or pests) or illegal extractions.
Table 4 Tree carbon content by forest type and by study community in the Monarch Butterfly Biosphere Reserve.
Study classification | Tree carbon (Mg C·ha-1) |
---|---|
Dominant trees | |
Conifers | 112 ± 66 |
Broadleaf | 98 ± 42 |
Mixed | 91 ± 45 |
Potential vegetation | |
Sacred fir | 86 ± 75 |
Sacred fir-pine-broadleaf species | 109 ± 45 |
Pine-oak-broadleaf species | 92 ± 34 |
Community | |
Carpinteros indigenous community | 90 ± 34 |
Donaciano Ojeda indigenous community | 132 ± 46 |
Francisco Serrato indigenous community | 88 ± 48 |
San Juan Zitácuaro ejido | 84 ± 53 |
± Standard deviation of the mean.
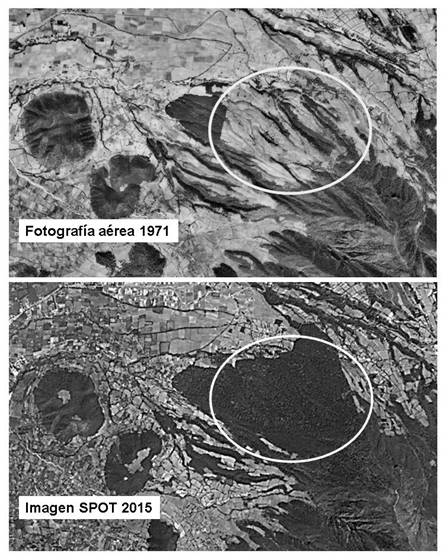
Figure 4 Example of forest reconversion in the San Juan Zitácuaro ejido, Michoacán, 1971-2015. Top image: fragment of orthophoto R3-20A-11(31) from INEGI, 1971. Bottom image: view of SPOT 5 585/311 panchromatic band image, taken in February 2015.
Brigade members from the four communities showed interest in learning tree measurement and monitoring skills, indicating the presence of social conditions receptive to the incorporation of new techniques for the efficient management of their resources. In the ENAREDD+, participatory measurement, reporting and verification (PMRV) does not appear as a form of recording carbon gains or as an activity financed with program resources (CONAFOR, 2014); however, the authorities and brigade members expressed motivation both in terms of obtaining data to improve forest use and thus achieve economic benefits for the community, and in terms of the possibility that at a certain point in time they may be able to access funds for carbon sequestration.
Conclusions
The data obtained through participatory mapping and measurement of tree carbon by community members were consistent with those generated by experts. Communities were able to directly identify areas with potential for carbon sequestration and may thus consider this in their management plans. The participatory methods used are adaptable to the skills, interests and needs of the community; they promote awareness, facilitate community discussion for the management of their resources, and assist in linking local processes with the objectives of external programs in which they can participate. In the four agrarian nuclei analyzed, it could be inferred that the content of carbon stocks depends more on the degree of conservation of the site than on the type of vegetation, and forest disturbance is more associated with internal and external conflicts than with forest management itself.