Serviços Personalizados
Journal
Artigo
Indicadores
-
Citado por SciELO
-
Acessos
Links relacionados
-
Similares em SciELO
Compartilhar
Revista mexicana de ciencias agrícolas
versão impressa ISSN 2007-0934
Rev. Mex. Cienc. Agríc vol.7 no.5 Texcoco Jun./Ago. 2016
Articles
Dynamics of rainwater in trees low deciduous forest
1Campo Experimental Valles Centrales -INIFAP. Melchor Ocampo No. 7, C. P. 68200, Santo Domingo Barrio Bajo, Etla, Oaxaca, México.
2Colegio de Postgraduados. Carretera México-Texcoco, km 36.5 C. P. 56230, Montecillo, Texcoco, México.
3Centro Nacional de Investigación Disciplinaria en Relación Agua Suelo Planta Atmósfera (CENID RASPA-INIFAP). km 6.5 Canal Sacramento, Las Huertas, C. P. 35140, Gómez Palacio, Durango, México.
The Low Deciduous Forest develops in semi-arid areas with water deficits and vegetative cover to the greatest extent at global and national level with 42% and 60% coverage, respectively. One of the areas that best represent this biosystem in Mexico is the Mixteca region. The tree cover of this forest, by the interception of raindrops canopy, impact runoff and water erosion. In order to know the effect of canopy interception of rain, cauline flow, flow sheet canopy and intercepted by foliage he was measured. These measurements were used to establish mathematical relationships using multiple linear regression, which allowed to calculate their magnitudes; through known values of sheet rain, event, and geometry of the canopy. Measurements were made during 2013 in 21 trees (9 species) predominate in Low Deciduous Forest, 350 rain events occurred in five representative sites in the Mixteca region. Trees on average recorded a flow of canopy 66.5%, 6.1% cauline flow and interception 27.5%. Field data identified the best models to evaluate the flow dynamics rainwater. Between observed was obtained and predicted values an R2 of 0.83 for the intercepted sheet flow of 0.96 to 0.67 for canopy and cauline flow. These relationships are useful to feed simulation models that estimate the hydrological balance of this ecosystem.
Keywords: canopy flow; canopy interception; flow cauline; Mixteca region
La Selva Baja Caducifolia se desarrolla en áreas semiáridas con déficit de agua y es la cubierta vegetal con la mayor extensión a nivel mundial y nacional con 42% y 60% de cobertura, respectivamente. Una de las zonas que mejor representan éste biosistema en México es la Región Mixteca. La cobertura arbórea de esta selva, por la intercepción de las gotas de lluvia del dosel, impacta el escurrimiento y la erosión hídrica. Con el propósito de conocer el efecto del dosel en la intercepción de la lluvia, se midió el flujo caulinar, el flujo de dosel y la lámina interceptada por el follaje. Estas mediciones se usaron para establecer relaciones matemáticas, utilizando regresión lineal múltiple, que permitieron calcular sus magnitudes; a través de valores conocidos de lámina de lluvia, por evento, y geometría del dosel. Las mediciones se realizaron durante 2013, en 21 árboles (9 especies) predominantes de la Selva Baja Caducifolia, para 350 eventos de lluvia ocurridos en cinco sitios representativos de la región Mixteca. Los árboles en promedio registraron un flujo de dosel 66.5%, flujo caulinar 6.1% e intercepción 27.5 %. La información de campo permitió identificar los mejores modelos para evaluar la dinámica del flujo de agua de lluvia. Entre valores observados y predichos se obtuvo un R2 de 0.83 para la lámina interceptada, de 0.96 para flujo de dosel y de 0.67 para flujo caulinar. Estas relaciones son útiles para alimentar modelos de simulación que estimen el balance hidrológico de este ecosistema.
Palabras clave: flujo de dosel; flujo caulinar; intercepción dosel; Región Mixteca
Introduction
The Low Deciduous Forest is a fragile and low resilience ecosystem characterized by its high level of endemism, seasonality and presence of deciduous trees of low height with extended cups; generally it located in mountainous formations with moderate to strong slopes. The vegetation of this biosystem covers 42% extension worldwide and in Mexico is the kind of tropical vegetation with the largest geographical distribution (60%), García et al. (2005). In Mexico it is distributed primarily on a strip on the side of the Pacific Ocean from the state of Sonora to Chiapas, and in small isolated areas throughout the national territory (Trejo, 1999; Castillo et al., 2007).
In semi-arid climatic environments, common in the area of tropical Low Deciduous Forest in Mexico, the effect of vegetation is important because it regulates various water processes including interception of precipitation (Fathizadeh et al., 2014), which is a subject little studied plant species for semiarid climate (Delphis et al., 2011; Méndez et al., 2014; Pérez et al., 2014; Zhi-Bin et al., 2014; Sadeghi et al., 2015). The analysis of this process, and generating parameters applicable to this type of vegetation, will assess and evaluate the flow behavior of precipitated water and determine the actual influence of plants on the gain (infiltration) and water loss (interception). The elements to evaluate the components of fractionation of rain, for plant species, are the flow of canopy and cauline flow (Van Dijk and Bruijnzeel, 2001; Clark et al., 2008; Muzylo et al., 2009; Bulcock and Jewitt, 2010; Bulcock and Jewitt, 2012; Dohnal et al., 2014). A determining factor for calculating the interception variable, the cauline flow and flow canopy, for each plant species, is the leaf area index (IAF) and the precipitated sheet (Luo et al., 2002; Boken and Chandra, 2012; Martin et al., 2013).
The interception of the rain begins to drop water on the vegetation, part of it through the foliage (flow canopy) and decreases the rate of fall of water precipitated, another small part runs off the trunk or main axis plants (cauline flow) and can reach the ground surface (Sun et al., 2013; Seyed et al., 2015). The measurement of this process for both arid and semiarid conditions has it done in huizache trees (Acacia farnesiana) in which is recorded on average 15-27%; mesquite (Prosopis laevigata), with values 17-27%; acacia (Acacia rigidula and Acacia berlandieri), 1.12 to 3.6% and Huamuchil (Pithecellobium ebano and Pithecellobium pallens), 1.5 to 10.3% (Návar et al., 2008; Mastachi et al. 2010). In coniferous forest interception variation is 11-51% for the same species (Xu et al., 2013).
As part of the experimental work already mentioned, they do not have specific studies quantifying water flow to the main native tree species that grow in Low Deciduous Forest, why this research developed at different sites was conducted study of the Mixteca region, with the aim of generating values of basic water parameters, depending on the geometry of the canopy, useful for hydrological simulation models. Quantification of water flows allow the water issue recommendations for assessment of plants.
Materials and methods
Study area
The Mixteca region is made up of 254 of the 194 municipalities which belong to the state of Oaxaca, Guerrero and 16 to 44 to Puebla. As the Mixteca region a very large area, for the state of Puebla two representative locations were selected (Tecomatlán and Tehuitzingo), one for Guerrero (Xochihuehuetlán and Huamuxtitlán), and one for Oaxaca (San Pedro Anane). These sites are located between 17° 31´ 30.1”- 18° 20´ 18.8” north latitude and 97° 22' 08.4”- 98° 34´ 54.3” west longitude.
The Mixteca is part of the morphotectonic province of the highlands mother of the south and highlands of Oaxaca, this presents a rugged terrain with altitudes ranging from 1 000 to 2 000 m and slopes greater than 8%.
In the study area, the predominant vegetation cover is Low Deciduous Forest mainly used for cattle pasture. This ecosystem, located from sea level to 1 700 msnm, the thermal and water limit the types of vegetation of the warmhumid areas. They occur in areas with above average annual temperatures 20 °C and average annual rainfall of about 800 mm; with a severe dry season which can last 7 or 8 months. Based on FAO (2014) classification the predominant soils in the region are leptosols, regosols and phaeozem.
Selection of vegetation
The trees selected for analysis of the flow of rainwater, were chosen based on the following criteria: low water consumption, pivoting root system deep roots, extensive glass, low demand for nutrients, nitrogen fixers, and abundance in landscape (Table 1).
Table 1 Species studied trees of low deciduous forest, Mixteca region, Mexico.
Localidad | Nombre común | Nombre científico | Núm. de árbol |
San Pedro Añañe, Oaxaca | Enebro | Juniperus fláccida | 1 |
Enebro | Juniperus fláccida | 2 | |
Enebro | Juniperus fláccida | 3 | |
Rhus | Rhus standleyi | 4 | |
Rhus | Rhus standleyi | 5 | |
Tecomatlán, Puebla | Palo blanco | Acacia coulteri | 1 |
Casahuate blanco | Ipomoea arborescens | 2 | |
Casahuate blanco | Ipomoea arborescens | 3 | |
Tehuitzingo, Puebla | Palo camarón | Caesalpinia hintonii | 1 |
Palo camarón | Caesalpinia hintonii | 2 | |
Palo camarón | Caesalpinia hintonii | 3 | |
Espino | Acacia sp. | 4 | |
Espino | Acacia sp. | 5 | |
Xochihuehuetlán, Guerrero | Tehuixtle | Acacia bilimekii | 1 |
Tehuixtle | Acacia bilimekii | 2 | |
Copal hediondo | Bursera submoniliformis | 3 | |
Copal hediondo | Bursera submoniliformis | 4 | |
Copal hediondo | Bursera submoniliformis | 5 | |
Huamuxtitlán, Guerrero | Pochote de secas | Ceiba parvifolia | 1 |
Pochote de secas | Ceiba parvifolia | 2 | |
Pochote de secas | Ceiba parvifolia | 3 |
In these species the crown area (Ac) and leaf area index (IAF) through equations determined Dieguez et al. (2003). The IAF was calculated with the CSIRO Land and Water Forest Tools v1.0 software (Siggins, 2014) by taking, with three repetitions per tree, hemispherical photographs (Rajaei, 2014).
Water flows. Fractionation of rain, for selected plant species consisted of measuring rainfall during the cycle of the cauline flow (Fc), canopy flow (Fd) and precipitation (Pp). To measure Fc, they were placed, spirally shaped, plastic tubing 1.0 m long in the stem of the tree; the flow of the hoses are caught in a container placed at the foot of the tree (Figure 1). To determine the Fc, equation 1 was used:
Where: Fc= cauline flow (mm); Ac= crown area (m2); V= volume captured in the container by rain event (m3).
The precipitated water flowing through the canopy (Fd) was measured with rain gauges canopy, consisting of a triangular metal conduit and resizable, depending on the size of the foliage, the average length of the channel was 0.36 m. This rain gauge was placed under the canopy and with sufficient gap to concentrate water through a hose to a container for registration (Figure 1). The Fd is calculated with equation 2:
Where: Fd= canopy flow (mm); V= volume captured in the container by rain event (m3); Acc= catchment area of the chute (m2).
The precipitation was measured with a standard rain gauge records were performed in units of volume, then they became a film considering a factor of 35 milliliters per millimeter (Figure 1). Intercepted sheet (Li) was calculated using Equation 3.
Where: Li= intercepted sheet (mm); Pp= site precipitation (mm); Fd= canopy flow (mm); Fc= cauline flow (mm).
Analysis of data. In 2013, five sites were recorded 350 observations, with which the values of Fc, Fd and Li, for each plant species selected study sites were obtained. Intercepted sheet calculated (Lic) was determined in linear relationship with IAF. The flow equations canopy and cauline flow (Fdc and Fcc) were obtained through a mathematical analysis of multiple regression using SAS (SAS, 2010) software, depending on the leaf area index, precipitation and the intercepted sheet.
Results and discussion
Fractionation of rain
In the Table 2 shows the observed averages reported by instrumented tree, leaf area and water bodies in precipitation to reach the canopy dissociates. The behavior of the components of fractionation rain corresponds to that described by Swaffer et al. (2014) suggests that greater cauline flow increased precipitation, canopy and intercepted flow sheet; the latter with a maximum limit set by the leaf area index of each plant species.
Table 2 Observed values fragmentation of rain, average per tree.
Localidad | Núm. de árbol | Eventos de lluvia | Af (m2) | IAF | Pp (mm) | Fd(mm) | Fc(mm) | Li(mm) |
San Pedro Añañe, Oaxaca | 1 | 19 | 3.44 | 1.72 | 14.1 | 10.1 | 0.6 | 3.42 |
2 | 16 | 3.29 | 1.05 | 13.6 | 11 | 0.6 | 2.08 | |
3 | 17 | 3.2 | 0.85 | 13.8 | 11.8 | 0.3 | 1.69 | |
4 | 19 | 11.31 | 1.5 | 13.3 | 10.4 | 0.4 | 2.5 | |
5 | 18 | 8.38 | 1.52 | 13.7 | 10.3 | 0.3 | 3 | |
Tecomatlán, Puebla | 1 | 10 | 104.1 | 1.41 | 19.5 | 14.1 | 0.8 | 4.55 |
2 | 7 | 19.85 | 0.78 | 15.3 | 9.7 | 2.4 | 3.23 | |
3 | 14 | 11.71 | 1.09 | 15.9 | 12.6 | 0.9 | 2.32 | |
Tehuitzingo, Puebla | 1 | 10 | 50.46 | 5.9 | 20.7 | 10.6 | 2.2 | 7.96 |
2 | 19 | 23.1 | 2.58 | 14.9 | 9.1 | 0.4 | 5.45 | |
3 | 13 | 34.53 | 2.6 | 16.1 | 9.4 | 1.1 | 5.64 | |
4 | 16 | 10.54 | 2.6 | 16.2 | 9.3 | 1.5 | 5.47 | |
5 | 13 | 8.66 | 2.65 | 13.9 | 7.9 | 0.5 | 5.58 | |
Xochihuehuetlán, Guerrero | 1 | 23 | 218.54 | 4.14 | 22.4 | 13.7 | 1.6 | 7.16 |
2 | 21 | 322.16 | 5.52 | 22.6 | 14.4 | 0.8 | 7.42 | |
3 | 18 | 103.1 | 2.4 | 23.3 | 14.4 | 3.5 | 5.41 | |
4 | 19 | 212.09 | 3.22 | 24.8 | 14.8 | 3.2 | 6.81 | |
5 | 12 | 145.5 | 3.39 | 24.2 | 13.8 | 3.2 | 7.16 | |
Huamuxtitlán, Guerrero | 1 | 22 | 63.63 | 1.8 | 8.7 | 5 | 0.1 | 3.62 |
2 | 24 | 36.23 | 1.44 | 8.3 | 5.3 | 0.1 | 2.86 | |
3 | 20 | 8.8 | 0.87 | 5.5 | 3.6 | 0.1 | 1.72 | |
Total | 21 | 350 | Promedio | 2.33 | 16.2 | 10.5 | 1.2 | 4.5 |
Máximo | 5.9 | 24.8 | 14.8 | 3.5 | 7.96 | |||
Mínimo | 0.78 | 5.5 | 3.6 | 0.1 | 1.7 |
* IAF= índice de área foliar; Af= área foliar; Pp= precipitación; Fd= flujo de dosel; Fc= flujo caulinar y Li= lámina interceptada.
In percentage terms, for trees analyzed (Figure 2), average values were recorded for 66.5% canopy flow, flow cauline 6.1% and 27.5% intercepted sheet; extreme values for flow canopy were 79.2 to 56.8%, 13.8 to 1.6% of cauline flow and in case of interception ranged from 36.9 to 17.3%. The results Reed intercepted rainfall coincide with those reported in the literature, according to Maloney et al. (2002) may be of the order of 15 to 35%; while the flow canopy can reach 77% (Ufoegbune et al., 2010).

Figure 2 Composition of the sheet of rain (%) observed for trees of Low Deciduous Forest, Mixteca region.
By type of tree shown, in percentage terms, the maximum intercepted film was observed in the thorn (Acacia sp.) With 36.9%, while values lower interception were obtained for juniper (Juniperus flaccida) and casahuate white (Ipomoea arborescens); with 17.3 and 17.9% respectively, these minimum values correspond to those obtained by Flores et al. (2013).
In general terms shows (Figure 2) the inverse relationship between the intercepted flow sheet and canopy, which favors the cauline flow is maintained around 6.1% of the total rain sheet is observed; except hediondo copal (13.8%) and white casahuate (10.8%) that presented the highest values.
Rainfall interception. The IAF was considered as a standard parameter (Gómez et al., 2001; De Jong and Jetten, 2007; Vegas et al., 2012) to correlate the sheets intercepted by trees studied. This correlation between IAF and the measured values of Li, was performed using simple linear regression.
The correlation between observed and calculated values (Lic), a coefficient of determination was obtained (R2) of 0.8281 (Figure 3). The equation describing the intercepted sheet for the main plant species Low Deciduous Forest, is as follows:
Where: Lic= intercepted calculated sheet (mm); leaf area index (IAF).
Flow canopy. To determine the predictive flow model canopy (Fdc) was correlated through multiple linear regression, the flow of canopy observed (Fd) with measurements of leaf area index (IAF), precipitation (Pp) and intercepted sheet (Li). The correlation between observed values (Fd) and calculated (Fdc), a coefficient of determination was obtained (R2) of 0.9621 (Figure 4). The equation describing the flow of canopy for the main plant species growing in the Low Deciduous Forest ecosystem, is:
Where: Fdc= calculated flow canopy (mm); leaf area index (LAI); Pp= precipitation (mm); Li= intercepted sheet (mm).
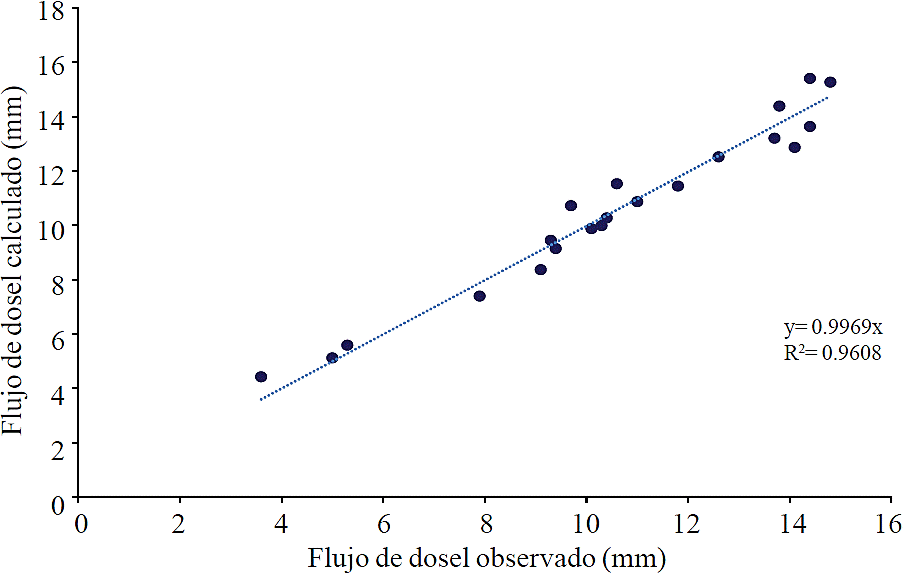
Figure 4 Flow canopy observed and calculated (equation 5), Species of Deciduous Forest, Mixteca region.
The equation 5 applies only when the different fractions of the flow are known, in the event that the intercepted sheet is not known, it is convenient to apply the following equation obtained by substituting Lic equation 4 in Li equation 5:
Where: Fdc= calculated flow canopy (mm); leaf area index (IAF); Pp= precipitation (mm).
Conclusions
This paper analyzed the water parameters in the rain is split, reaching the canopy, the main native plants growing in the Low Deciduous Forest ecosystem of the Mixteca region. The study of these components is one of the first studies to quantify the cauline flow, flow foliage canopy and interception for this type of vegetation.
In the species studied the sheet interception was related directly, regardless of the magnitude of precipitation, with leaf area index 83%. According to the models generated, the flow cauline canopy and flow can be estimated with precipitation data occurred, IAF and sheet interception. The models show an inverse relationship between the intercepted flow sheet and canopy, which is reflected in caulinees flows with minor variations, about 6.1% of the sheet of rain.
Since a greater leaf area index allowed the plants to intercept a larger sheet of precipitation, in this regard, to slow the process of water erosion, species like the stick shrimp (Caesalpinia hintonii) and tehuixtle (Acacia bilimekii) are recommended, that they have high rates of leaf area and values above 30% interception. On the other hand, trees with high flows caulinees favor natural groundwater recharge, such as stinky copal (Bursera submoniliformis) and white casahuate (Ipomoea arborescens). The tree that provides an ideal balance between flow canopy and the cauline flow, both to avoid surface runoff as to promote water infiltration into the soil, is the stick shrimp (Caesalpinia hintonii), which is also widely used in the area study as cattle feed.
This research is one of the first work aimed at quantifying the cauline flow, flow and canopy rainfall interception by the foliage of the main native plants growing in the low deciduous forest ecosystem of the Mixteca region. The generation of hydrological parameters for the fractionation of the rain that reaches the canopy of vegetation, hydrological models will feed simulation to assess the environmental water-vegetation such services.
Literatura citada
Boken, V. K and Chandra, S. 2012. Estimating leaf area index for an arid region using spectral data. Afr. Crop Sci. J. 20(4):215- 223. [ Links ]
Bulcock, H. H. and Jewitt, G. P. W. 2010. Spatial mapping of leaf area index using hyperspectral remote sensing for hydrological applications with a particular focus on canopy interception. Hydrol. Earth System Sci. 14:383-392. [ Links ]
Bulcock, H. H. and Jewitt, G. P. W. 2012. Modelling canopy and litter interception in commercial forest plantations in South Africa using the Variable Storage Gash model and idealised drying curves. Hydrol. Earth System Sci. 16:4693-4705. [ Links ]
Castillo, C. G.; Dávila, A. P. y Zavala, H. J. A. 2007. La selva baja caducifolia en una corriente de lava volcánica en el centro de Veracruz: lista florística de la flora vascular. Boletín de la Sociedad Botánica de México. 80:77-104. [ Links ]
Clark, D. B.; Olivas, P. C.; Oberbauer, S. F.; Clark, D. A. and Ryan, M. G. 2008. First direct landscape-scale measurement of tropical rain forest Leaf Area Index, a key driver of global primary productivity. Ecology Letters. 11:163-172. [ Links ]
De Jong, S. M. and Jetten, V. G. 2007. Estimating spatial patterns of rainfall interception from remotely sensed vegetation indices and spectral mixture analysis. Inter. J. Geographical Inf. Sci. 21(5):529-545. [ Links ]
Delphis, F. L.; Darryl, C. M. and Tadashi, T. 2011. Forest hydrology and biogeochemistry. Springer. Netherlands. Ecol. Studies 216. 740 p. [ Links ]
Diéguez, A. U.; Barrio, A. M.; Castedo, D. F.; Ruíz, G. A. D.; Álvarez, T. M. F.; Álvarez, G. J. G. y Rojo, A. A. 2003. Dendrometría. Ediciones Mundi-Prensa. México. 327 p. [ Links ]
Dohnal, M.; Cerný, T.; Votrubová, J. and Tesar, M. 2014. Rainfall interception and spatial variability of throughfall in spruce stand. J. Hydrol. Hydromechanics. 62(4):277-284. [ Links ]
Fathizadeh, O.; Attarod, P.; Keim, R. F.; Stein, A.; Zahedi, G. A. and Asghar, A. D. 2014. Spatial heterogeneity and temporal stability of throughfall under individual Quercus brantii trees. Hydrol. Processes. 28:1124-1136. [ Links ]
Flores, A. E.; Becerra, L. F.; Buendía, R. E.; Carrillo, A. F.; Terrazas, G. G. H.; Pineda, O. T. y Acosta, M. M. 2013. Intercepción de lluvia por matorral inerme espinoso en Atotonilco el Grande, Hidalgo. Rev. Mex. Cienc. Agríc. 2(4):285-298. [ Links ]
FAO. 2014. World reference base for soil resources 2014. International soil classification system for naming soils and creating legends for soil maps. World Soil Reosurces Reports 106. Rome. 191 p. [ Links ]
García, R. A.; Mendoza, R. K. I. y Galicia, S. L. 2005. Valoración del paisaje de la selva baja caducifolia en la cuenca baja del río Papagayo (Guerrero), México. Investigaciones Geográficas, Boletín del Instituto de Geografía- Universidad Nacional Autónoma de México (UNAM). 56:77-100. [ Links ]
Gómez, J. A.; Giraldez, J. V. and Fereres, E. 2001. Rainfall interception by Olive trees in relation to leaf area. Agricultural Water Management. 49:65-76. [ Links ]
Luo, T.; Ronald, N. P.; Hanqin, T.; Vörösmarty, C. J.; Zhu, H. and Liu, S. 2002. A model for seasonality and distribution of leaf area index of forests and its application to China. J. Vegetation Sci. 13:817-830. [ Links ]
Maloney, D.; Bennett, S.; De Groot, A. and Banner, A. 2002. Canopy interception in a hypermaritime forest on the North Coast of British Columbia. Extensión Note # 49. Prince Rupert Forest Region. British Columbia. 6 p. [ Links ]
Martin, K. A.; Van Stan II, J. T.; Dickerson-Lange, S. E.; Lutz, J. A.; Berman, J. W.; Gersonde, R. and Lundquist, J. D. 2013. Development and testing of a snow interceptometer to quantify canopy water storage and interception processes in the rain/ snow transition zone of the North Cascades, Washington, USA. Water Res. Res. 49:3243-3256. [ Links ]
Mastachi, L. C. A.; González, E. S.; Becerril, R. P. y Braud, I. 2010. Pérdidas por intercepción en mezquite (Prosopis laevigata) y huizache (Acacia farnesiana) de la región semiárida del centro de México. Tecnología y Ciencias del Agua. 1(1):103-120. [ Links ]
Méndez, B. L. A.; Vivoni, E. R.; Robles, M. A.; Mascaro, G.; Yepez, E. A.; Rodriguez, J. C.; Watts, C. J.; Garatuza, P. J. and Saiz, H. J. A. 2014. A modeling approach reveals differences in evapotranspiration and its partitioning in two semiarid ecosystems in Northwest Mexico. Water Res. Res. 4(50):3229-3252. [ Links ]
Muzylo, A.; Llorens, P.; Valente, F.; Keizer, J. J.; Domingo, F. and Gash, J. H. C. 2009. A review of rainfall interception modelling. J. Hydrol. 370:191-206. [ Links ]
Návar, C. J. de J.; Méndez, J. G. y González, H. R. 2008. Intercepción de la lluvia en especies de leguminosas del Nordeste de México. Terra Latinoam. 26(1):61-68. [ Links ]
Pérez, S. M.; Arredondo, M. J. T.; Huber, S. E. and Serna, P. A. 2014. Forest structure, species traits and rain characteristics influences on horizontal and vertical rainfall partitioning in a semiarid pine- oak forest from Central Mexico. Ecohydrology. 2(7):532-543. [ Links ]
Rajaei, N. M. 2014. Use of smartphone to derive the leaf area index. Thesis Master of Geoinformation Science. Center for Geoinformation. Wageningen University. Wageningen. The Netherlands. 68 p. [ Links ]
Sadeghi, S. M. M.; Attarod, P. and Pypker, T. G. 2015. Differences in rainfall interception during the growing and non-growing seasons in a Fraxinus rotundifolia Mill. Plantation located in a semiarid climate. J. Agric. Sci. Technol. 1(17):145-156. [ Links ]
SAS, 9.3. 2010. SAS Institute Inc. Cary NC. 27513, USA. [ Links ]
Seyed, M. M. S.; Attaroda, P.; Toland, J. V. S. II; Grant, T. P. and Dunkerley, D. 2015. Efficiency of the reformulated Gash’s interception model in semiarid afforestations. Agric. Forest Meteorol. 201:76-85. [ Links ]
Siggins, A. 2014. Commonwealth Scientific and Industrial Research Organisation (CSIRO) Land and Water Forest Tools v1.0. Australia. [ Links ]
Sun, X.; Wang, G.; Lin, Y.; Liu, L. and Gao, Y. 2013. Intercepted rainfall in Abies fabri forest with different-aged stands in southwestern China. Turk. J. Agric. Forestry. 37:495-504. [ Links ]
Swaffer, B. A.; Holland, K. L.; Doody, T. M. and Hutson, J. 2014. Rainfall partitioning, tree form and measurement scale: a comparison of two co-occurring, morphologically distinct tree species in a semi-arid environment. Ecohydrology. 5(7):1331-1344. [ Links ]
Trejo, V. I. 1999. El clima de la selva baja caducifolia en México. Investigaciones Geográficas (Mx). 39:40-52. [ Links ]
Ufoegbune, G.C.; Ogunyemi, O.; Eruola, A.O. and Awomeso, J. A. 2010. Variation of interception loss with different plant species at the University of Agriculture, Abeokuta, Nigeria. African J. Environ. Sci. Technol. 4:831-844. [ Links ]
Van Dijk, A. I. J. M. and Bruijnzeel, L. A. 2001. Modelling rainfall interception by vegetation of variable density using an adapted analytical model. Part 2. Model validation for a tropical upland mixed cropping system. J. Hydrol. 247:239-262. [ Links ]
Vegas, G. F.; Álvarez, C.; García, A. and Revilla, J. A. 2012. Estimated distributed rainfall interception using a simple conceptual model and Moderate Resolution Imaging Spectroradiometer (MODIS). J. Hydrol. 468-469:213-228. [ Links ]
Xu, Z., Feng, Z.; Zhao, C.; Zheng, J.; Yang, J.; Tian, F.; Peng, H.; Wang, C.; Peng, S. and Sher, H. 2013. The canopy rainfall interception in actual and potential distribution of Qinghai spruce (Picea crassifolia) forest. J. Hydrol. Hydromech. 61(1):64-72. [ Links ]
Zhi-Bin, H.; Jun-Jun, Y.; Jun, D.; Wen-Zhi, Z.; Hu, L. and Xue-Xiang, C. 2014. Spatial variability of canopy interception in a spruce forest of the semiarid mountain regions of China. Agric. Forest Meteorol. 188:58-63. [ Links ]
Received: April 2016; Accepted: July 2016