Servicios Personalizados
Revista
Articulo
Indicadores
-
Citado por SciELO
-
Accesos
Links relacionados
-
Similares en SciELO
Compartir
Revista mexicana de ciencias agrícolas
versión impresa ISSN 2007-0934
Rev. Mex. Cienc. Agríc vol.7 no.7 Texcoco sep./nov. 2016
Articles
Expansión factors aboveground biomass for Pinus ayacahuite of the north of Oaxaca
1Instituto Tecnológico del Valle de Oaxaca (ITVO). Ex hacienda de Nazareno, Oaxaca, C. P. 71230. Tel: 01(951)5170444. (migue_1_magui@hotmail.com).
2División de Estudios de Posgrado e Investigación-ITVO. (grodriguez.itvo@yahoo.com; jenriquezdelvalle@yahoo.com; leonisa70@hotmail.com; casta_h50@hotmail.com).
3Universidad de la Sierra Juárez, Ixtlán, Oaxaca. (forest_wsg@hotmail.com).
The biomass is an important and difficult determining factor in forest species, biomass expansion factors (FEB) allow their inventory estimation using variables to infer the biomass and carbon stocks. The objective was to determine FEB variables for aboveground biomass of Pinus ayacahuite using easily measured variables in natural stands of Ixtlan, Oaxaca. In 2013 destructive sampling was held of 30 trees selected in proportion to the relative frequency of diameter class. The biomass was separated into leaves, branches and wood+bark; the volume and biomass were estimated by allometric fimctions. The volume linear model had the best coefficient of determination (0.99). The compartmental biomass was estimated with nonlinear models, presenting pseudo-R2 of 0.91, 0.91 and 0.97 for leaves, twigs and bole+bark, respectively; the total aboveground biomass was estimated normal diameter (DN) and total height (AT) (psedo-R2= 0.97). The partition of aboveground biomass is distributed 5, 14 and 81% in leaves, branches and bole+bark, respectively. The FEB presented an average value of470.9 kg for a tree with average dimensions of DN= 17.9 cm and AT= 16.2 m.
Keywords: Pinus ayacahuite; allometric function; biomass partition; destructive sampling
La biomasa es un factor importante y difícil de determinar en especies forestales, los factores de expansión de biomasa (FEB) permiten su estimación utilizando variables de inventario para inferir la biomasa y carbono almacenado. El objetivo fue determinar FEB variables para biomasa aérea de Pinus ayacahuite, utilizando variables de fácil medición en rodales naturales de Ixtlán, Oaxaca. En 2013 se realizó muestreo destructivo de 30 árboles seleccionados de forma proporcional a la frecuencia relativa de la clase diamétrica. La biomasa se separó en follaje, ramas y madera+corteza; el volumen y biomasa fueron estimados mediante funciones alométricas. El modelo lineal de volumen obtuvo el mejor coeficiente de determinación (0.99). La biomasa por compartimentos se estimó con modelos no lineales, presentando pseudo-R2 de 0.91, 0.91 y 0.97 para hojas, ramas y fuste+corteza, respectivamente; la biomasa total aérea se estimó con diámetro normal (DN) y altura total (AT) (psedo-R2= 0.97). La partición de biomasa aérea se distribuye 5, 14 y 81% en hojas, ramas y fuste+corteza, respectivamente. El FEB presentó un valor promedio de 470.9 kg para un árbol con dimensiones promedio de DN= 17.9 cm y AT= 16.2 m.
Palabras clave: Pinus ayacahuite; función alométrica; muestreo destructivo; partición de biomasa
Introduction
There is currently a worldwide concern about global warming and one of the main environmental challenges is climate change mitigation (Acosta-Mireles et al, 2002; Salinas and Hernández, 2008). The global warming has been the result of increased greenhouse gases, mainly carbon dioxide (CO2) which increases considerably by human activities (Acosta et al, 2001); for this, the United Nations has introduced the REDD+ mechanism to reduce emissions of pollutants (Manuri et al, 2014).
Although the trees in terms of net photosynthesis are less than many annuals productivity, are considered as a carbon sink globally by the magnitude and longevity of individuals and area occupied forest ecosystems (Dixon et al., 1994). The forests globally, set and store large amounts of carbon during their lifetime and may control up to 90% of the annual flux of carbon between the atmosphere and the earth's surface (Apps et al, 1993; Ordoñez et al., 2011). Trees in particular store large amounts of carbon throughout their life, even though they could also represent a net source of CO2 (Dixon et al., 1994; Masera, 2002).
IEE (2006) and SEMARNAT (2001) report that land use, changes in land use and forestry (USCUSS) in Mexico during the period 1993 to 2002 showed 14% (89 854 Gg CO2 equivalent) of CO2 emissions, therefore their role as a carbon sink needs further investigation. The estimate of biomass in forests is an important issue in relation to the problem of global warming. In recent years they have increased interest in studying the role of forests in biogeochemical cycles, especially carbon, and its relationship to greenhouse gases. It is assumed that approximately 50% of the estimated biomass is carbon and therefore can be added to the atmosphere as carbon dioxide (CO2) when cut and burned (Morrissey and Justus, 1998).
The estimate of carbon stocks in an ecosystem can be determined with the amount of air and ground tree biomass, soil, compost, fermentation layer, herbs and shrubs. There are direct and indirect to estimate the biomass of a forest methods. The direct method is to cut the tree and weigh biomass directly. Another way is to calculate the biomass indirectly through equations and mathematical models, obtained by regression analysis between the variables collected in field and forest inventories (Brown, 1997).
Due to the high cost and time, they have been used simple methods, such as application of expansion factors biomass (FEB), which consist transform stem volume per tree or per unit surface density of C for tree or by the same unit area for each vegetation type. These facilitate the calculations of the density of C from the actual stock in a forest. The aim of this study was to determine allometric models of wood volume, biomass and FEB variables for air compartments Pinus ayacahuite var. veitchii Shaw natural stands under management in Ixtlan, Oaxaca.
Materials and methods
Study area
The study area is located in the northwestern part of the state of Oaxaca, in the Sierra Norte region. Pinus ayacahuite is between 96° 27'31.278" north latitude and 17° 24' 01.286" west longitude in the community of Ixtlan de Juarez, Oaxaca. Its distribution includes 1 137 ha in altitudes of 2 680-2 960 m and is associated with P. patula Schl. et Cham, P. pseudostrobus Lindl and Quercus sp.
In 2013 12 circular sites (400 m2) randomly established and directed a density stands of trees and different forestry methods throughout the study area. In the sample units woodland inventory was performed to obtain the diameter and height structure, which served to distribute the sample trees to break down in proportion to the relative frequency.
Biomass sample
The obtaining biomass of each air compartment it was carried out following the methodology proposed by Rodríguez-Ortiz et al. (2012). The destructive sample consisted of 30 trees, without deformities, representative of the categories in diameter and height; in each tree several variables inventory were measured (normal diameter (DN), total height (AT), length of clear bole (LFL), length of live crown (LCV), crown diameter (DC) and diameter of stump (DT ). The diameter was obtained with diameter tape (model 283D/20F, Jackson, MS, USA), lengths with longímetro® and height with electronic inclinometer (Haglóf EC II, Sweden). The trees were felled, sliced and separated by components (branches, foliage and wood with bark), using chainsaw (Husqvarna® 50 cm).
Estimating biomass of branches and leaves
The nine samples per tree were taken (three in each section of the cup), in which leaves and branches were separated by placing them in paper bags properly identified with progressive tree and branch numbers; was obtained green weight (PV, in g) of both components using a model Dhaus CS scale with capacity of 5 000 g.
The samples of leaves and branches dried in an oven model MemmertBeschichung/Loading-Moll 100-800 at an average temperature of 75 °C until the constant weight. With DHAUS CS model scale with capacity of 5 000 g dry weight it was recorded (PS, in gr) corresponding constant of each sample of leaves and branches. In total 30 samples of each component they were obtained. The estimate of biomass of branches and leaves and tree branch level was obtained by adjusting nonlinear regression models using the Nlin Method= Dud procedure of SAS statistical package (SAS Institute Inc., 2004).
Estimation of biomass wood with bark
After removing branches and leaves of trees felled 30, each stem is sectioned into commercial logs of 2.57 and 1.25 m in length depending on the quality of each product. Before measuring each commercial trozathree slices of10 cm average thickness as a sample of each tree, which were identified and heavy instantly obtained for its green weight (PV, g). In each slice vernier measured with Scala mark to the nearest millimeter 222A®, thickness and diameter in different axes (cm) for fresh volume (VV, cm3). For their constant dry weight (PS, g) took to the drying oven at an average temperature of 105 °C. With these data the specific gravity (GE= PS/VV, g cm-3), whose product with the volume of each log was obtained, generated stem biomass of each log and individual.
Estimating biomass component tree wood+bark level was performed using nonlinear regression models, using the same procedure and statistical package using variables easy field measurement.
Getting volume tables and biomass expansion factor
To obtain the volume table with and without bark, were adjusted regression models that helped predict the different volumes of trees, depending on variables easy field measurement (normal diameter, DN) and overall height, AT). The models were fitted using linear regression techniques using the GML procedure of SAS statistical package (SAS Institute Inc., 2004); evaluated through their statistical values, coefficient of determination R2, amount of residual mean square error (CME) and dispersion of waste. The biomass expansion factors were obtained by the ratio of total aboveground biomass and total tree bark.
Results and discussion
Volume models with and without bark
The model volume with and without bark tree (VTcc and VTsc) for Pinus ayacahuite presented a good fit in their prediction parameters, with a coefficient of determination (R2) of 0.99 in both variabless, CME 0.034 in VTcc and 0.035 in VTsc and coefficient of variation of 13.7 and 15.9%, respectively. Both parameters of the equation showed significance of p≤ 0.0001. The relationship between volume and diameter of trees can be described by a quadratic linear function (Figure 1).
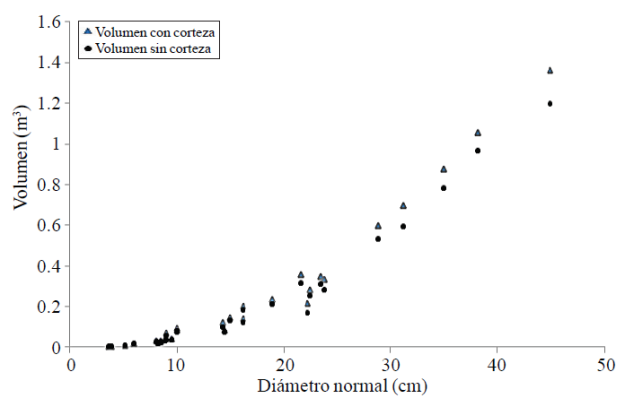
Figure 1 Relationship between normal diameter and volume with and without bark of Pinus ayacahuite of Ixtlan, Oaxaca.
VTCC= 0.0004670490 (DN2)+0.0000088023 (DN2xAT)
VTSC= 0.000376587 (DN2) + 0.0000092893 (DN2 x AT)
Where: VTCC, SC= total volume with bark and bark (m3), DN= normal diameter (cm), AT= total height (m).
The fit coefficients and other statistical models volume is substantially improved by involving, in addition to the DN, the AT generating highly reliable equations (R2= 0.99). The like P. ayacahuite, many species of cloud forest (Acosta et al, 2011), Pinus cooperi Blanco (Arroyo and Paredes, 2006) and P. chiapensis (Chávez-Pascual et al, 2013) have shown this improvement in their estimates.
Models to estimate biomass by air compartment
The regression model showed a good fit in different prediction parameters biomass branch level was the nonlinear model (1 and 2), with pseudo-R2= 0.81 in leaves and 0.74 in branches showing significance (p< 0.0001) in both models (Table 1).
Table 1 Nonlinear models for estimating biomass in Pinus ayacahuite branch level of Ixtlan, Oaxaca.

BH= biomasa de hoja (kg); BR= biomasa en rama (kg); ABR= área basal de la rama (n/4xDB2, cm2); DB= diámetro de base de la rama (cm); A= altura de inserción de la rama en el tallo (m); ß0,1,2= coeficientes de regresión.
The estimate tree biomass level according to their compartments, according to the regression models showed high p-R2 (0.91) in leaves and branches and 0.97 for biomass and aboveground stem (Table 2).
Table 2 Nonlinear models to estimate biomass components air a level tree Pinus chiapensis of Ixtlan, Oaxaca.

BT= biomasa total aérea (kg); BH= biomasa en hojas (kg); BR= biomasa en ramas (kg) y BF= biomasa en tallo con corteza (kg). DC= diámetro de copa (m); AT= altura total (m); DN= diámetro normal (cm), ß0,1,2= coeficientes de regresión.
The total biomass model that best fit the data was the considered the normal diameter and tree height as independent variables for this study. These match Figueroa-Navarro et al. (2009), who indicate that both variables using statistical estimators inventory equations are improved.
Compartmental distribution of biomass and biomass expansion factors (FEB)
The average distribution of biomass by Pinus ayacahuite compartment for stem was 81%, 14% to branches and leaves 5%. The results show that a greater diameter greater accumulation of biomass in any of the compartments in question; however, small trees tend to allocate more to the compartments of leaves and branches.
The amount of air that total biomass was estimated to Pinus ayacahuite is in a range between 26.95 and 147.57 t ha1, generated in the study area (1137 ha) between 30 644.49 and 167 788.36 tB. The largest proportion of biomass is on the stem (72.81 t ha-1) and lowest in the foliage (3.3 t ha-1) (Table 3).
Table 3 Estimated volume, biomass component and total air of Pinus chiapensis in Ixtlan, Oaxaca.

&Intervalos de confianza (95%) mediante la prueba t-Student, tα/2 (n-1).
The study shows that in the shaft is most (81%) of tree biomass, varied according to the size of the tree. Diaz-Franco et al. (2007) reported similar values (82% stem biomass) and Figueroa-Navarro et al. (2009), found n range from 36 to 85% for Pinus patula Schl. et Cham. Some biomass values reported in other species such as Pinus pseudostrobus Lindl. (Commercial plantations), are in a range of 65.7 to 82%, depending on the location and age of the stand (Méndez-González et al., 2011).
Jimenez (2010) found an average of 65.31% stem biomass in P. hartwegii Lindl. and Pacheco (2011) reports similar values (63.25%). However, this distribution is low compared to other studies, for example; Avendaño et al. (2007) found that religious Abies religiosa Kunt Schltdl. et Cham, the average biomass of 81.27% shaft is, as González (2008) found 87.6% stem biomass 6 tree 67.2 cm diameter in P. maximinoi H. E. Moore, these reported values are Similar to P. ayacahuite under study.
The biomass sheets P. ayacahuite (species of interest) accounted for 5% of the total aboveground biomass with a constant behavior with respect to shaft diameter (about the diameter class 15 onwards). This value is different reporting Rodríguez-Ortiz et al. (2012) of 2.4% because the study was conducted in young stands.
The foliar biomass in trees younger ages reported 18% in Pinus patula (Díaz-Franco et al., 2007). In Salto, Durango; Pacheco et al. (2007), found 25% of foliar biomass plantation P. greggii Engelm of 6 years old. González (2008) reports a distribution of biomass in branches of 8.49% for P. maximinoi and 11.20% for P. oocarpa Shiede, these values are close or equal to P. ayacahuite (species under study). Other studies found high results, such Jiménez (2010) found 23.79% in P. hartwegii and Pacheco (2011) found 23.49% for P. patula.Gayoso et al. (2002) note that the proportional distribution of biomass in the tree components, is associated with the characteristics of trees by species, the state of development and degree of silvicultural to exposed individuals interventions.
Aboveground biomass of the species of interest showed a range of 26.95 to 147.57 t ha-1. Similar results 29.4 to 122.1 and average (39.36 57 t ha-1) were reported for P. pseudostrobus Lindl. (Mendez-González et al, 2011). In turn, Gutiérrez and Lopera (2001) estimated 87.2 and 446 tB ha-1 in commercial plantations of P. patula of 6 and 29 years respectively. About Rodríguez-Laguna et al. (2007) found a ratio of546.90 kg biomass in trees normal diameter of 5 to 42 cm for P. pseudostrobus.
This variation between P. ayacahuite, P. patula and P. pseudostrobusoccurs under such values are of species, age, geographical location and different methodologies to those used for this study. This indicates that in commercial plantations of fast growing process biomass accumulation is faster than in natural forests. The increase in biomass depends mainly on soil and climatic conditions including the rate of return and depending on the responsiveness presenting the trees will grow and therefore their biomass in relation to carbon sequestration (Acosta et al., 2001).
With the resulting model biomass expansion factor (FEB) it is possible to know the total biomass accumulated air from a tree, knowing is a function of the height and normal diameter. In this study the FEB presented a value of 470.9 kg m-3 for an average normal diameter of 17.92 cm and a height of 16.2 m.
Where: FEB= biomass expansion factor (kg m-3); AT=total height (m); DN = normal diameter (cm).
The biomass expansion factor (obtained in inventory), provided an estimate biomass aerial total of 470.9 kg per tree average tree in (17.92 cm diameter and total height 16.2 m) in the species of interest. These values agree with Chávez-Pascual et al. (2013) that determined 444.14 kg/ tree average size of 27.7 cm average diameter and total height of
23.3 m in P. chiapensis (Martínez) Andresen. On the other hand, Silva-Arredondo and Návar-Cháidez (2010) obtained from 0.55 to 0.98 t per tree in Pinus sp.
Conclusions
The biomass expansion factor for Pinus ayacahuite corresponds to 470.9 kg m-3 for an average tree with normal diameter of 17.92 cm and a height of 16.2 m. So therefore the obtained models for predicting biomass branch level and tree species can be used in inventories for the estimation of aboveground biomass in natural stands, knowing normal diameter (DN), total height (AT) and crown diameter (DC).
Pinus ayacahuite has a partition aboveground biomass of 81, 14 and 5% in stem+bark, branches and leaves, so that in mature trees biomass concentration is much higher in the stem, having the greatest potential to store biomass and therefore carbon in its compartments.
Literatura citada
Acosta, M. M.; Carrillo, A. F. y Gómez, V. R. G. 2011. Estimación de biomasa y carbono en dos especies del bosque mesófilo de montaña. Re. Mex. Cienc. Agric. 2(4):529-543. [ Links ]
Acosta, M. M.; Quednow, K.; Etchevers, J. y Monreal, C. 2001. Un método para la medición del carbono almacenado en la parte aérea de sistemas con vegetación natural e inducida en terrenos de ladera en México. Colegio de Postgraduados. México. 1-11 pp. [ Links ]
Acosta-Mireles, M.; Vargas-Hernández, J.; Velázquez-Martínez, A. y Etchevers-Barra, J. D. 2002. Estimación de biomasa aérea mediante el uso de relaciones alométricas en seis especies arbóreas en Oaxaca, México. Agrociencia. 60:725-736. [ Links ]
Apps, M. J.; Kurz, W. A.; Luxmoore, R. J.; Nilsson, L. O.; Sedjo, R. A.; Schmidt, R.; Simpson, L. G. and Vinson, T. S. 1993. Boreal forest and tundra. In: Wisniewski, J. and Sampson, R. N. (Eds.) Terrestrial biosphere carbon fluxes: quantification and sources of CO2. Klwer Academic Pub. 39-53 p. [ Links ]
Arroyo, T. J. y Paredes, J. E. G. 2006. Estimación de la biomasa total y por componentes de hojas, ramas, copa y fuste en Pinus cooperi de la región de El Salto, Durango, México. Instituto Tecnológico del Salto, Durango, México. 37 p. [ Links ]
Avendaño, H. D.; Acosta, M. M.; Carrillo, A. F. y Etchevers, B. J. D. 2007. Estimación de la biomasa y carbono en árboles de Abies religiosa (H. B. K) Schl. et Cham., mediante ecuaciones alométricas. In: Memoria del VII Congreso Mexicano de Recursos Forestales, 28 al 31 de octubre. Morelia Michoacán, México. 1-9 pp. [ Links ]
Brown, S. 1997. Bosques y cambio climático y la función de los bosques como sumideros de carbono. Antalya, Turquía. 2 p. [ Links ]
Chávez-Pascual, E. Y.; Rodríguez-Ortiz, G.; Carrillo-Rodríguez, J. C.; Enríquez-del Valle, J. R.; Chávez-Servia, J. L. y Campos-Ángeles, G. V. 2013. Factores de expansión de biomasa aérea para Pinus chiapensis (Mart.)Andresen. Rev. Mex. Cienc.Agríc. 6:1273-1284. [ Links ]
Díaz-Franco, R.; Acosta-Mireles, M.; Castillo-Anzures, F.; Buendía-Rodríguez, E.; Flores-Ayala, E. y Etchevers-Barra, J. D. 2007. Determinación de ecuaciones alométricas para estimar biomasa en Pinus patula Schl. et Cham. Revista Madera y Bosques. 13(1):25-34. [ Links ]
Dixon, R. K.; Brown, S.; Houghton, R. A.; Solomon, A. M.; Trexler, M. C. and Wisniewski, J. 1994. Carbon pools and flux of global forest ecosystems. Science. 263:185-190. [ Links ]
Figueroa-Navarro, C. M.; Ángeles-Pérez, G.; Velázquez-Martínez, A. y De los Santos-Posadas, H. M. 2009. Estimación de la biomasa en bosque bajo manejo de Pinus patula Scht all. et. Cham. en Zacualtipán, Hidalgo. Rev. Mex. Cienc. Fores. 1(1):105-112. [ Links ]
Gayoso, J.; Alarcón, D. y Trincado, G. 2002. Biomasa total de los ecosistemas forestales y acumulación de carbono". Programa Bosques Pro-Carbono de la Universidad Austral de Chile (Sociedad Inversora Forestal). Valdivia Chile. 38 p. [ Links ]
González, Z. M. 2008. Estimación de la biomasa aérea y la captura de carbono en regeneración natural de Pinus maximinoi H. E. Moore. Pinus ocarpa var. ochoterenai Mtz, y Quercus spp. en el norte del estado de Chiapas, México. Tesis de maestría, CentroAgronómico Tropical de Investigación y Enseñanza. CATIE-Costa Rica. 81 p. [ Links ]
Gutiérrez, V. V. H. y Lopera, A. G. J. 2001. Metodología para la cuantificación de existencias y flujo de carbono en plantaciones forestales. In: Simposium Internacional de medición y monitoreo de la captura de carbono en ecosistemas forestales. Universidad Nacional de Colombia. Valdivia-Chile. Resumen N° 10. 1-18 pp. [ Links ]
IEE. 2006. Inventario nacional de emisiones de gases de efecto invernadero. (1990-2002). 554 p. [ Links ]
Jiménez, C. C. 2010. Usos de ecuaciones alométricas para estimar biomasa y carbono en la parte aérea de Pinus hartweii Lindl. Tesis de licenciatura. Universidad Autónoma Chapingo (UACH). México. 55 p. [ Links ]
Manuri, S.; Brack, C.; Nugroho, N. P.; Hergoualc'h, K.; Novita, N.; Dotzauer, H.; Verchot, L.; Septiadi, P. C.A. and Winyasari, E. 2014. Tree biomass equations for tropical peat swamp forest ecosystems in Indonesia. Forest Ecol. Management. 334: 241-253. [ Links ]
Masera, O. 2002. Bosques y cambio climático en América Latina. Análisis y perspectiva. In: la transición hacia el desarrollo sustentable. Perspectivas de América Latina y el Caribe. Le, E.; Ezcurra, I.; Pisanty, E. y Romero, L. P. (Eds). SEMARNAT- Instituto de Ecología- Universidad Autónoma Metropolitana (UAM). Organización de las Naciones Unidas. Programa de las Naciones Unidas para el Medio Ambiente. 211-235 pp. [ Links ]
Méndez-González, J. M.; Luckie-Navarrete, S. L.; Capó-Arteaga, M.A. y Nájera-Luna, J. A. 2011. Ecuaciones alométricas y estimación de incrementos en biomasa aérea y carbono en una plantación mixta de Pinus devoniana Lindl. y P. pseudostrobus Lindl., en Guanajuato, México. Agrociencia. 45:479-491. [ Links ]
Morrissey, A. y Justus, J. 1998. Global climate change. Committee for The National Institute for the Environment, Washington D.C. 32 p. [ Links ]
Ordoñez, J.A. 2011. Captura de carbono en bosque templado: en el caso de San Juan Nuevo, Michoacán, México. Agrociencia. 31:73-81. [ Links ]
Pacheco, A. G. 2011. Ecuaciones alométricas para estimar biomasa aérea por compartimentos en reforestaciones de Pinus patula Schl. et Cham., en Xiacuí, Ixtlán, Oaxaca. Universidad de la Sierra Juárez. Oaxaca, México. 58 p. [ Links ]
Pacheco, E. F. C.; Alderete, A.; Gómez G. A.; Fierros, G., A. M.; Cetina, A. V. M. y Vaquera, H. H. 2007. Almacenamiento de carbono en la biomasa aérea de una plantación joven de Pinus gregii Engelm. Rev. Fitotec. Mex. 30 (3): 251-254. [ Links ]
Rodríguez-Laguna, R.; Jiménez-Pérez, J.;Aguirre-Calderón, O. y Jurado-Ibarra, E. 2007. Ecuaciones alométricas para estimar biomasa aérea en especies de encino y pino en Iturbide, N. L. Rev. Cien. Forest. 32(101):39-56. [ Links ]
Rodríguez-Ortiz, G.; de los Santos-Posadas, H. M.; González-Hernández, V.; Aldrete, A. V.A.; Gómez-Guerrero, A. y Fierros-González, A. M. 2012. Modelos de biomasa aérea y foliar en una plantación de pino de rápido crecimiento en Oaxaca. Revista Madera y Bosques. 18(1):25-41. [ Links ]
Salinas, Z. y Hernández, P. 2008. Guía para el diseño de proyectos MDL forestales y de biotecnología. CATIE, Turrialba, C.R. 171 p. [ Links ]
SAS Institute Inc. 2004. SAS/STAT 9.1 User's guide. SAS Institute, Cary, N.C. USA. 4979 p. [ Links ]
Secretaría del Medio Ambiente y Recursos Naturales (SEMARNAT). 2001. Estadística de deforestación en México: 1993-2000. México, D.F. 236 p. [ Links ]
Silva-Arredondo, F. M y Návar-Cháidez, J. J. 2010. Factores de expansión de biomasa en comunidades forestales templadas del norte de Durango, México. Rev. Mex. Cienc. Forest. 1(1):55-62. [ Links ]
Received: January 2016; Accepted: April 2016