The Guatemalan fir (Abies guatemalensis) is an endangered species in Mesoamerica and is commonly utilized as a Christmas tree in Guatemala. Over a span of 45 years, the extraction of trees and branches from natural forests has resulted in a staggering 95% reduction in populations (CONAP, 2010). This species is listed in the IUCN Red List of Threatened Species and is categorized under Appendix I of CITES, thereby prohibiting international trade. To address the national demand for Christmas trees, Guatemalan fir plantations were established in the 1990s, which not only mitigated the impact on natural forests but also contributed to species conservation. The cultivation of Guatemalan fir has emerged as an effective conservation strategy rooted in sustainable utilization (Strandby et al., 2006; Strandby et al., 2008).
One of the primary challenges encountered in Guatemalan fir plantations revolves around pest management, including aphids (Mindarus sp.), sooty mold (Capnodium sp.) resulting from aphid excretions, and root rot caused by Phytophthora. Recently, a noteworthy pest has been observed, significantly impacting the plantations: the “green algae” of the Guatemalan fir (Strandby et al., 2008; INAB, 2018). This phenomenon is characterized by a powdery green coverage that manifests on trunks, leaves, and can even envelop entire branches. The biofilm formed by the algae diminishes light penetration and facilitates organic matter accumulation, thereby posing a risk of branch mortality. Furthermore, it compromises the aesthetic quality of the trees and diminishes their market value, consequently jeopardizing the profitability of Guatemalan fir cultivation. While the name implies an association with green algae, limited research exists regarding the identification of the algae and its potential correlation with other microorganisms, such as fungi (INAB, 2018; INAB, 2019).
Terrestrial algae have the ability to thrive on inert surfaces such as rocks and buildings, as well as on the surfaces of living organisms, including plants and animals (Novakova and Neustupa, 2015). This ecological phenomenon is referred to as epibiosis, where one organism, known as the epibiont, resides on the surface of another organism, the basibiont, potentially impacting it in various ways (Wahl, 2009). Algae frequently form symbiotic associations with other microorganisms, such as bacteria and fungi, resulting in the formation of biofilms (Freystein and Reisser, 2010).
Previous studies by multiple authors have documented instances of algae and fungi biofilms colonizing the leaves of Abies and other conifers in Europe and North America (Sochting, 1997; Poggio, 2002; Novakova and Neustupa, 2015). Nevertheless, this particular phenomenon has not been previously documented in A. guatemalensis. Consequently, the primary objective of this research endeavor was to identify the specific algae and fungi species that comprise the biofilm found on the leaves of the Guatemalan fir (Abies guatemalensis).
Materials and methods
Sample collection. During a one-year period from March 2019 to March 2020, three Guatemalan fir plantations situated in the departments of Chimaltenango (Valle Alto Estate), Guatemala (Billebén Estate), and San Marcos (Buena Vista Estate) were visited. The selection of these plantations was based on records provided by the National Institute of Forests, which documented the presence of the biofilm in 10 plantations and one natural forest (Figure 1). In each plantation, a targeted sampling strategy was implemented, whereby five Guatemalan fir trees displaying signs of “green algae” infestation, with a height exceeding two meters and a diameter greater than 10 cm at a height of 1.5 m, were carefully chosen. The georeferencing of the selected trees was conducted, followed by the selection of four infested branches from each tree, with one branch being sampled at each cardinal point. Detailed photographic documentation of the branches was obtained, after which the branches were excised, placed in plastic bags, and stored in a cooler for transportation to the Plant Protection Laboratory at the Universidad del Valle de Guatemala. Subsequently, the branches were examined under a stereomicroscope, and the leaf coverings were gently scraped to facilitate microscopic analysis for the identification of algae and fungi.

Figure 1 Records of pinabete (Abies guatemalensis) plantations where the biofilm formed by algae and fungi was present.
Algae identification. Two leaves per branch were selected for analysis, resulting in a total of eight leaves per tree and 40 leaves per sampling site. The leaf coverings were gently scraped, and each leaf was disinfested for 5 minutes using an antibiotic solution (Gentamicin 50 µg mL-1, Streptomycin 100 µg mL-1, and Carbendazim 64 µg mL-1). The scrapings were then vacuum-filtered and inoculated onto solid and liquid culture media following the methods outlined by Stanier et al. (1971), Andersen (2005), and Videv et al. (2017). Kuhl and Lorenzen’s (1964) culture medium was utilized, which consists of 10 mM KNO3, 4.5 mM NaH2PO4 * 1 H2O, 0.5 mM Na2HPO4 * 2 H2O, 1 mM MgSO4 * 7 H2O, 0.1 mM CaCl2 * 2 H2O, 0.025 mM FeSO4 * 7 H2O, 1 μM ZnSO4 * 7 H2O, 1 μM MnSO4 * 1 H2O, 1 μM H3BO3, 0.01 μM (NH4)6Mo7O24 * 4 H2O, and 0.01 μM CuSO4 * 5 H2O.
The liquid cultures were maintained with constant aeration and CO2 pumping at a rate of 60 bubbles per minute, at a temperature of 28 ºC, and under a 12-hour photoperiod using fluorescent lamps. Daily monitoring of the cultures was conducted to identify instances of bacterial and fungal growth. Subsequently, the cultures were filtered and inoculated onto potato dextrose agar (PDA) medium to facilitate the identification of contaminating microorganisms. Following one week of growth, the algae were examined under a light microscope for genus-level identification. Taxonomic keys for terrestrial algae (Graham and Wilcox, 2009; Freystein and Reisser, 2010; John and Rindi, 2015; Archibald et al., 2017), as well as the current classification based on the AlgaeBase and Index Nominum Algarum databases (AlgaeBase, 2023), were consulted as references.
Identification of fungi. The fungi were isolated from the collected leaves using an equivalent number of samples as in the algae identification process. Segments measuring 2 cm in length were carefully excised and subjected to disinfection with a 2% sodium hypochlorite solution and distilled water. Subsequently, the plant material was inoculated onto potato dextrose agar (PDA) culture medium. Four leaves from each branch were placed in individual Petri dishes and incubated at a temperature of 28 ºC for a period of 5 days. Fungal growth that appeared on the leaves was cultivated, and the resulting cultures were purified using the hyphal tipping technique (Soto et al., 2017). In order to achieve genus-level identification, reproductive structures and spores were mounted on microscope slides and examined under a light microscope. Taxonomic keys by Farr et al. (1989) and Phillips and Burdekin (1992) were utilized as references during the identification process.
DNA extraction and molecular analysis of fungi. Five fungi were identified at the species level through genetic analysis. DNA extraction followed the CTAB protocol by Murray and Thompson (1980). A 1.5 mL Eppendorf tube was loaded with 300 mg of mycelium, and 600 μL of preheated CTAB extraction buffer (2% CTAB, 1% polyvinylpyrrolidone [PVP], 100 mM Tris-HCl pH 8.0, 1.4 M NaCl, 20 mM EDTA, and 0.2% 2-mercaptoethanol) was added at 65 °C. The mycelium was homogenized using an automated homogenizer, followed by the addition of 500 μL of chloroform:isoamyl alcohol (24:1). After centrifugation at 13,000 rpm for 10 minutes, the supernatant was transferred to a new tube. Subsequently, 500 μL of cold isopropanol and 50 μL of 7.5 M ammonium acetate were added, and the sample was incubated at -20 °C for one hour. Further centrifugation at 13,000 rpm for 10 minutes resulted in the decantation of the supernatant, which was followed by the addition of 150 μL of cold 70% ethanol. After centrifugation at 13,000 rpm for five minutes, the supernatant was decanted, and the precipitate was air-dried at 30 °C for 20 minutes. Finally, the precipitate was resuspended in 50 μL of preheated TE buffer (10 mM Tris-HCl, 1 mM EDTA) at 65 °C and stored at 4 °C.
DNA was quantified, and the ITS region was analyzed using Polymerase Chain Reaction (PCR) with specific primers: ITS1 (5’TCCGTAGGTGAACCTG-CGG3’) and ITS4 (5’TCCTCCGCTTATTGATATGC3’) (White et al., 1990). The target PCR product size was expected to be 650 bp. The reaction mixture had a volume of 25 μL, comprising 17.7 μL of sterile distilled water, 2.5 μL of 10X buffer containing 25 mM MgCl2, 1 μL of the dNTP mixture (10 mM), 1.3 μL of each primer (10 pmol μL-1), and 0.2 μL of Taq DNA polymerase (5U μL-1) (Invitrogen®). The amplification was performed in a thermocycler following this protocol: initial denaturation at 95 °C for 15 min, 35 cycles of denaturation at 94 °C for 30 s, annealing at 52 °C for 90 s, extension at 72 °C for 1 min, and a final extension step at 60 °C for 30 min.
The amplified fragments were visualized using 1% agarose gel electrophoresis and sent to Macrogen, Korea for sequencing. The obtained sequences were analyzed and compared with those stored in the GenBank database of NCBI (National Center for Biotechnology Information) using the BLAST (Basic Local Alignment Search Tool) program. Subsequently, the sequences were deposited to acquire their respective accession numbers (Zhang et al., 2000). These sequences have been deposited in the Sequence Read Archive (SRA) under the accession numbers SRR24660263, SRR24660264, SRR24660265, SRR24660266, and SRR24660267. These samples are associated with the BioProject accession number PRJNA974074.
Results and discussion
Field observations. Trunks and branches were discovered to be partially and fully infested with a biofilm (Figure 2). The presence of a green, powdery covering on trunks, branches, and leaves was used to identify the infestation, which originated at the base and extended upwards. In samples taken from Billebén Estate and Valle Alto Estate, branches exhibited a more pronounced biofilm with gray necrotic areas (Figure 2E), indicating an advanced stage of infestation. This occurrence can be attributed to the fungal infestation within the biofilm, as previously reported by Sochting (1997) in Picea abies leaves. Additionally, an increased occurrence of fungi on conifer leaves has been observed as the branches age (Carroll et al., 1977; Terhonen et al., 2011).

Figure 2 Pinabete trees (Abies guatemalensis) infested with algal and fungal biofilm. A and B) Trunks covered by the biofilm. C and D) Branches with partial biofilm coverage. E) Branch with total biofilm coverage. F) Infested pinabete branch with leaf buds free of infestation.
Even in branches completely infested, no biofilm coverage was observed on new leaf shoots (Figure 2F). This observation was made in October, while the onset of the rainy season is in May, when new shoots begin to emerge, suggesting that these shoots can remain biofilm-free for up to five months. Similar findings have been reported in Picea abies, where it was observed that new leaves become colonized by algae up to six months after bud formation, with older branches exhibiting a denser biofilm coverage (Sochting, 1997).
Traces of mosses (Figure 3B), foliose lichens (Figure 3C), mites, diatoms, pollen, and insect exoskeletons were also detected within the biofilm. This accumulation of debris and organic matter in the biofilm further hampers photosynthesis in the leaves and hastens senescence (Reuther, 1978; Sochting, 1997).

Figure 3 Pinabete branches and leaves infested with the algal and fungal biofilm seen under stereoscope. A) Pinabete bark 20 X. B) Pinabete branch 20 X. C and D) Pinabete branches 10 X. E) Pinabete leaf covered by biofilm with necrotic section 20 X. F) Pinabete leaf underside 45 X.
The field observations revealed that the biofilm of algae and fungi is not exclusively limited to Guatemalan fir. Leaf litter from other tree species exhibiting similar algae coverage was discovered in two plantations (Figure 4). This leaf litter was found in the canopy of affected Guatemalan fir trees, whereas the litter on the ground did not display any signs of the biofilm. This suggests that the biofilm adhered to the leaves due to their proximity to the source of inoculum.

Figure 4 Angiosperm leaves found in the canopy of pinabetes infested with algae. A and B) Location: Finca Billeben, Guatemala 15 X. C and D) Location: Finca Valle Alto 15 X.
The reasons why the Guatemalan fir is affected by the algae and fungi biofilm while other conifers in the same region, such as Pinus maximinoi or P. oocarpa, do not exhibit this phenomenon, are still unknown. According to Hoffman (1989), the growth of epiphytic algae is more influenced by physical factors such as temperature, light, and humidity than by the species of the host plant. However, other factors like bark pH and tree species can also influence algae colonization (Alwi et al., 2015; Stifterova and Neustupa, 2015), suggesting that Guatemalan fir trees may possess specific characteristics that facilitate the growth of these organisms.
Algae identification. In the 120 leaves of the 15 analyzed trees (5 trees per sampling site), four species of algae were found on the pinabete leaves (Table 1). The alga Desmococcus sp. was present in all three locations (Figure 5), two different species of the genus Klebsormidium were found in two locations (Finca Valle Alto and Finca Billeben), and a unicellular alga belonging to the class Trebouxiophyceae was found in one location (Finca Billeben). However, due to the lack of observable diagnostic characteristics, the genus of this alga could not be identified. Genetic analysis of the algae was infeasible due to the absence of pure cultures. Hence, there is a need to optimize the method of plant material disinfection by employing different concentrations of antifungal agents and disinfection durations that effectively eliminate fungi and bacteria while preserving algal growth (Laezza et al., 2022).
Table 1 Comparison of algae and fungi groups found in pinabete plantations in Chimaltenango, Guatemala and San Marcos, Guatemala.
Localidades | |||
---|---|---|---|
Clasificación | Finca Billeben, Guatemala | Finca Valle Alto, Chimaltenango | Finca Buena Vista San Marcos |
Algas | |||
Desmococcus sp. | X | X | X |
Klebsormidium sp1. | X | ||
Klebsormidium sp2. | X | ||
Trebouxiophyceae | X | ||
Hongos | |||
Alternaria sp. | X | ||
Aspergillus sp. | X | ||
Fusarium equiseti | X | ||
F. graminearum | X | ||
Fusarium sp. | X | ||
Mucor hiemalis | X | ||
Mucor sp. | X | ||
Trichoderma koningiopsis | X | ||
Trichoderma viride | X | ||
Trichoderma sp. | X | ||
Ulocladium sp. | X |
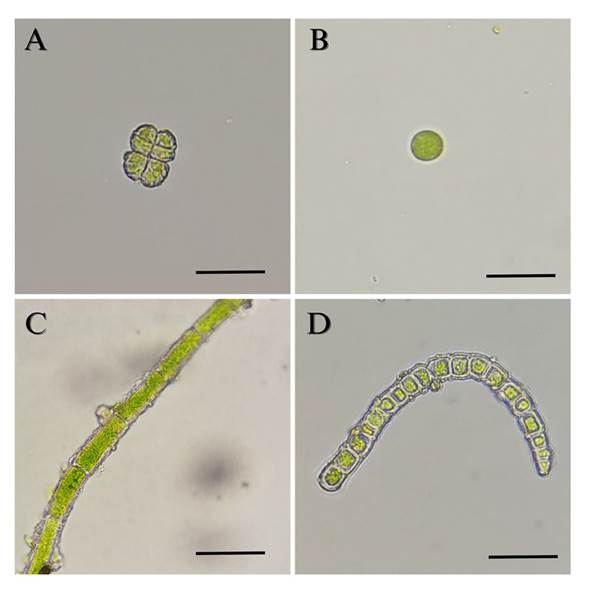
Figure 5 Algae isolated from pinabete leaves infested with algal and fungal biofilm. A) Desmococcus sp. 400 X. B) Trebouxiophyceae 400 X. C) Klebsormidium sp1. 400 X. D) Klebsormidium sp2. 400 X. Scale bar equals 10 μm.
The algae identified in this study belong to genera that have been documented as photosynthetic biofilm-forming organisms on both natural and artificial surfaces, including soil, plants, rocks, and structures (Ljaljević-Grbić et al., 2010; Rindi and Guiry, 2004; Stifterova and Neustupa, 2015). None of the identified algae have been reported as parasites or pathogens of plants. Instead, they utilize the bark and branches as suitable substrates for growth, often coexisting with other epibiotic organisms such as lichens or mosses (Neustupa and Albrechtova, 2003; Wahl, 2009).
The species composition of algae within the biofilm was found to vary across different locations, likely influenced by the maturity and age of leaf coverage. Similar to forest ecosystems, a succession of microorganism biofilms occurs, resulting in fluctuations in algae abundance and species richness over time (Neustupa and Albrechtova, 2003; Pogio, 2002). The alga Desmococcus sp. was consistently present in all locations, indicating its role as an early colonizer in the biofilm (Schnurr and Allen, 2015). In contrast, filamentous algae such as Klebsormidium tend to colonize the biofilm at later stages (Sochting, 1997). Consequently, the Finca Billebén and Finca Valle Alto locations harbor trees with more developed and mature biofilms. Additionally, these locations exhibited branches with necrotic patches, indicating an advanced fungal infestation.
The variability in species richness can also be attributed to factors such as bark pH, humidity, light exposure, and nitrogen and phosphorus concentration (Novakova and Neustupa, 2015). Forest management practices can also influence the species composition of the biofilm, as forests with higher frequencies of thinning and pruning exhibit reduced canopy coverage but higher species richness of algae (Glaser et al., 2018; Stifterova and Neustupa, 2015). These environmental and anthropogenic factors should be further investigated in future research to elucidate their impact on the development of biofilm on pinabete leaves.
Identification of fungi. From the analysis of 120 leaves collected from 15 trees, a total of 11 fungal species were isolated from Pinabete leaves (Table 1), representing six genera (Alternaria, Aspergillus, Fusarium, Mucor, Trichoderma, and Ulocladium). These genera comprise organisms with diverse ecological roles, including pathogens, saprophytes, and beneficial species. Through ITS region sequencing, five of the fungal species were identified at the species level (Table 2). When comparing the sequences of this study with those of the NCBI gene bank, homology ranged from 93.3 to 97.6 % (Table 2). This low homology may be due to other variants or species. Therefore, further morphological and molecular studies are considered pertinent.
Table 2 Percentage of sequence similarity of ITS region sequences of fungi isolated and identified from pinabete leaves
ID | Especie | Cobertura (%) | Homología (%) | Referencia de BLAST | Accesión |
---|---|---|---|---|---|
ITS seq of Fusarium b | Fusarium equiseti | 99.5 | 97.65 | F. equiseti isolate Anna6 small subunit ribosomal RNA gene, partial sequence; internal transcribed spacer 1, 5.8S ribosomal RNA gene, and internal transcribed spacer 2, complete sequence; and large subunit ribosomal RNA gene, partial sequence | MN498032.1 |
ITS seq of Fusarium R | F. graminearum | 99.65 | 96 | F. graminearum isolate PAK-14 small subunit ribosomal RNA gene, partial sequence; internal transcribed spacer 1, 5.8S ribosomal RNA gene, and internal transcribed spacer 2, complete sequence; and large subunit ribosomal RNA gene, partial sequence | MH054924.1 |
ITS seq of Mucor h | Mucor hiemalis | 99.31 | 93.55 | M. hiemalis isolate SL71_64a_Y2 internal transcribed spacer 1, partial sequence; 5.8S ribosomal RNA gene and internal transcribed spacer 2, complete sequence; and large subunit ribosomal RNA gene, partial sequence | MN105537.1 |
ITS seq of Trichoderma k | Trichoderma koningiopsis | 98.32 | 96.14 | T. koningiopsis isolate XXTF7 small subunit ribosomal RNA gene, partial sequence; internal transcribed spacer 1, 5.8S ribosomal RNA gene, and internal transcribed spacer 2, complete sequence; and large subunit ribosomal RNA gene, partial sequence | MN602642.1 |
ITS seq of Trichoderma v | T. viride | 97.23 | 94.23 | T. viride strain CTCCSJ-A-SD33116 internal transcribed spacer 1, partial sequence; 5.8S ribosomal RNA gene, complete sequence; and internal transcribed spacer 2, partial sequence | MF632117.1 |
Three species of Trichoderma (Trichoderma sp., T. koningiopsis, T. viride) were identified in Finca Billeben (Guatemala) and Finca Buena Vista (San Marcos). These species are known for their antagonistic effects against pathogens, particularly Fusarium, and have previously been isolated from Abies and other conifer leaves in Europe (Hanackova et al., 2015; Hayes, 1965; Tokumasu et al., 1994). Additionally, the fungi Alternaria sp. and Ulocladium sp. were found in Finca Billeben (Guatemala). These fungi have been associated with leaf necrosis and have been reported to impact various tree species (Danelia et al., 2021; Vannini and Vettraino, 2000).
In Finca Valle Alto, two species of Fusarium were identified: F. equiseti and F. graminearum. The latter is known to cause stalk rot in maize and primarily affects grasses, although it has been isolated from various plant families. Fungal spores are deposited and accumulate on leaves, serving as a potential source of inoculum for grasses (Miller et al., 2007). In this particular case, the spores of this fungus were likely transported from neighboring maize plantations, as maize is commonly associated with pinabete in an agroforestry model (INAB, 2019).
No fungal genus was found to be shared across all locations. However, the genera Mucor and Trichoderma were observed in two out of the three locations, and these genera have previously been co-isolated from decomposing conifer leaves (Hanackova et al., 2015; Hayes, 1965; Tokumasu et al., 1992).
Under microscopic observation of the biofilm scrapings, a close association between green algae and fungi was evident. Fungal mycelium with the accumulation of unicellular algae (Trebouxiophyceae) surrounding it was observed (Figure 6A and B), as well as fungal hyphae and spores adhering to filaments of the alga Klebsormidium sp. (Figure 6C and D). Terrestrial filamentous algae, including this genus, produce an extracellular matrix of polysaccharides that serve as attachment sites for other algae, cyanobacteria, and fungi (Warren et al., 2019). Additionally, fungal hyphae adhere to other components of the biofilm, such as soil particles, contributing to their aggregation and compaction (Belnap et al., 2003). The exact nature of the relationship, whether mutualistic, commensalistic, or parasitic, among the microorganisms comprising the biofilm remains to be elucidated and should be the focus of future investigations.
Conclusions
The biofilm affecting pinabete plantations in Guatemala is composed of epiphytic algae, fungi, and organic matter. Algae from the genera Desmococcus, Klebsormidium, and the class Trebouxiophyceae were found on 15 pinabete trees across three locations. These algae do not act as parasites but rather utilize pinabete as a substrate, akin to lichens and other epibionts. Fungi belonging to the genera Alternaria, Aspergillus, Fusarium, Mucor, Trichoderma, and Ulocladium were also present, exhibiting a range of pathogenic, saprophytic, and beneficial characteristics. Furthermore, the species richness of both algae and fungi demonstrated variability among the locations, which could be attributed to environmental or anthropogenic factors.