Introduction
In rural and urban areas three sources of fresh water resources for human consumption may be available: (a) rivers and water reservoirs; (b) porous aquifers (i.e. soil and sedimentary units); and (c) fractured rock aquifers (Freeze and Cherry, 1979; ANA, 2005). In regions where basement rocks outcrop, groundwater accumulates in opened planar structural features (i.e. joints, faults, foliation, veins, etc.), which serve as a complex conduit-barrier system.
Fractured rock aquifers are controlled by fracture properties such as aperture, spacing (or fracture density), orientation, infillings (e.g. sand, clay, cemented, etc.), roughness (particularly for fractures with small apertures), connectivity, state of stress and nature of host rocks (Costa, 2008; Fernandes, 2008; Singhal and Gupta, 2010; Bense et al., 2013). Fracture network facilitates chemical weathering and dissolution of host rock increasing hydraulic conductivity and groundwater storage (Hiscock, 2005; Brassington 2007).
Unlike fractured aquifers, the literature about the hydrogeological characteristics of rock aquifers related to Precambrian shear zones in Brazil is deficient (Neves and Morales, 2006). Shear zones are tabular regions of concentrated deformation across which adjacent undeformed rock units are offset (Alsop and Holdsworth, 2004). They are linear to curvilinear structures whose length is much greater than width. They are recognized at all scales and can be classified into brittle, ductile and brittle-ductile. Brittle shear zones are characterized by an array of parallel to subparallel fractures and veins, formed under fracturing, brecciation and cataclastic processes in the upper Earth’s crust (< 10 km). Ductile shear zones are characterized by the presence of mylonitic rocks, where crystal-plastic deformation processes take place. Typically, such processes occur in the middle to lower Earth’s crust. Brittle-ductile shear zones comprise features from both endmembers (Hobbs et al., 1976).
Metamorphic rocks exposed in the southern region of the state of Espírito Santo (Brazil) are cut by the Guaçuí and Batatal NE-trending shear zones (Figure 1). These outstanding structures are tens of kilometers long and can be easily traced from remote sensing and aerogeophysical data (Silva, 2010). From 2014 to 2016 the Espírito Santo state faced the worst drought in the last 80 years according to government agencies, which resulted in water supply problems and economic losses. Some important locations lay over these features, such as the Guaçuí, Marechal Floriano and the tourist town of Domingos Martins. Population of the three towns combined reach 81,000 inhabitants and land has been extensively used for cattle raising and coffee and eucalyptus plantations (https://cidades.ibge.gov.br/).
In this sense, the aim of this paper is to investigate the hydrogeological significance of these shear zones for groundwater prospecting and management. The use of remote sensing data for the analysis of landforms associated to subsurface geophysical surveys can elucidate the hydrogeological complexity of fractured aquifer system in regions where basement rocks outcrop (Fernandes, 2008; Singhal and Gupta, 2010). Besides, it can assist the conservation of areas of aquifer recharge and the identification of groundwater reservoirs (Dottridgea & Jaber, 1999; Rubin and Hubbard, 2005; Yabel et al., 2015; Salles et al., 2018; Sandoval & Tibujan Jr., 2019). In view of this, we integrate geophysical, remote sensing and geological and structural data.
Study area
Regional geology and tectonics
The Araçuaí orogen, located in southeastern Brazil, and its African counterpart, the West Congo belt, constitute an orogenic system that developed during the Brasiliano-Pan-African orogeny between the margins of the São Francisco and Congo cratons (Alkmim et al., 2006). The Araçuaí-West Congo orogen (A-WC orogen) was formed during the amalgamation of Western Gondwana in Late Neoproterozoic time and split into two parts by the opening of the South Atlantic Ocean in the Cretaceous. The Araçuaí orogen comprises rift-related to distal passive margin sequences, ophiolitic remnants of the precursor Macaúbas basin, Rio Doce magmatic arc-related rocks and pre-collisional to post-collisional intrusions - G1 to G5 Supersuits (Pedrosa-Soares et al., 2011). Paleoproteroic to Archean basement rocks include high-grade metamorphic rocks included in the Caparaó, Juiz de Fora, Ipanema, Guanhães and Serra do Valentim complexes. These represent reworked rocks related to the São Francisco Craton (Vieira et al. 2015; Alkmim et al. 2006).
The Guaçuí and Batatal NE-trending shear zones are one of the most strikingly geological features encountered in the southern region of the Espírito Santo State and can be easily traced from satellite images and aerogeophysical data for tens to hundreds of kilometers (Figure 1). These geological structures were formed in the final tectonic stages of the A-WC orogen (Pedrosa-Soares et al., 2001; Alkmim et al. 2006).
The Guaçuí and Batatal shear zones cross paragneisses belonging to the Paraíba do Sul Complex and pre to late-tectonic granitoids (Vieira, 1997). The Guaçuí Shear Zone (GSZ) is 350 km long and 5 km wide on average and is comprised of mylonites (outer margins) and ultramylonites (core region), showing subvertical mylonitic foliation. The GSZ is a right-lateral ductile shear zone developed under high-grade metamorphism (Cunninghan et al., 1998; Horn, 2007). The Batatal Shear Zone (BSZ) is 70 km long and comprise mylonitic foliation, being interrupted to the south by the post-collisional Iconha pluton (Féboli, 1993; Silva, 2010). According to Silva (2010), the BSZ is a brittle-ductile shear zone originated under low to medium-grade metamorphism.
After a long period of tectonic stability, the Araçuaí-West Congo orogenic belt was broke apart due to the opening of the Atlantic Ocean in the Cretaceous. This tectonic event was responsible for the intrusion of mafic magmatism, uplift of the Serra do Mar mountain range (to the south), reactivation of inherited geological structures and the development of marginal sedimentary basins, such as the Espírito Santo and Campos basins (Almeida, 1976). The Meso-Cenozoic brittle tectonics in the Brazilian southeastern margin was investigated by many authors (Riccomini, 1995, Ferrari, 2001, Zalán & Oliveira, 2005, Silva & Melo, 2011). In the Espírito Santo State, Ribeiro (2010) and Hartwig and Bozzi (2019) recognized three brittle deformation phases responsible for landform development and deformation of the semi-consolidated sediments of the Barreiras Formation (Miocene). Calegari et al., (2016) recognized a NW-trending Lineament in the Espírito Santo southeastern margin, named as the Alegre Fracture Zone, originated in the Cambrian Period and reactivated in the Cenozoic Era. Lourenço et al. (2016) obtained similar results for the Piúma lineament, a 70 km long and N50W-trending brittle shear zone. Maizatto et al. (2009) apud Chemale Jr & Hadler (2005) based on apatite fission tracks analysis, recognized an important tectonic event during 80-60 Ma in the south-central Espírito Santo State, which caused a regional uplift that reached up to 3,000 m.
Few stress data are available for the southern Brazil (Lima et al., 1997). According to the authors, measurements obtained from breakout for 145 wells in the Campos, Santos and Espírito Santo offshore basins are too scattered to define a regional SHmax orientation.
Soils and Landforms
Landforms in the study area are included in the Mantiqueira Northern Plateau geomorphological unit (Gatto et al. 1983). They are structurally controlled by NE to NNE-trending lineaments inherited from basement rocks as well as NW-trending lineaments. Hilly landforms with rounded summits and gentle to steep slopes predominate. Landscape is marked by a regional plateau around 700 m.a.s.l amid which rocky domes emerge, reaching elevations up to 1,700 m.a.s.l. These are usually associated to post-collisional igneous rocks.
Alluvium deposits are associate to the drainage system. They are made of a mixture of gravel, sand and clay (Vieira, 1997). Soil profile can reach tens of meters in regions where elevations are moderate to gentle and gneissic rocks occur. Soil is described as red and yellow latosols, podzols and cambisols (Panoso et al. 1978). Colluvium soils and talus are observed close to rock domes, which are very common in landscape.
Climate and Surface Hydrology
According to Köppen climate classification, Guaçuí town has an Aw (tropical savanna) climate type and Domingos Martins town has a Cfa (humid subtropical) climate type (https://pt.climate-data.org/). Guaçuí and Domingos Martins towns have average annual temperature around 21 degrees Celsius and average precipitation around 1,200 mm/year, with rainfalls occurring preferentially between October and March. The driest month occur in June and the rainiest month occur in December.
Drainage network is structurally controlled (Vieira, 1997). Itabapoana and Jucu rivers are the regional base level in the Guaçuí and Domingos Martins areas, respectively. Domingos Martins municipality has currently two small hydroelectric power stations in operation (< 30 MW) in the Jucu river and Guaçuí municipality has one (55 MW) in the Itabapoana river (www.annel.gov.br). River levels oscillate few meters along the year as a function of rainfall and seasons.
Materials and Methods
The methodology of this work involved the integration of remote sensing, structural and electrical resistivity data (Madrucci et al., 2005; Fernandes, 2008; Francese et al. 2009). Cartographic data were georreferenced in ArcGIS 10.4 (ESRI, 2015) using the horizontal Datum WGS-84 and the UTM map projection (Zone 24S). All data surveyed in this work was overlapped and integrated in the ArcGIS software.
The first step consisted in the interpretation of aerial photographs of the extinct Brazilian Coffee Institute - IBC (scale 1:30,000) freely available for the state of the Espírito Santo at the GEOBASES portal (https://geobases.es.gov.br/imagens-es-ibc-gerca-1970). The ST4 WILD Heerbruggg stereoscope was used for photogeological analysis. 11 stereo-pairs were studied and integrated for the GSZ and 5 stereo-pairs were used for the BSZ. Photogeological interpretation followed the procedures described in Soares and Fiori (1976) and Arcanjo (2011). The focus of the photogeological analysis was the identification of lineaments, which represent favorable features for groundwater storage. The term lineament used in this work followed the definition presented by O’Leary et al., (1976). Aerial photos were georeferenced based on the Divino de São Lourenço and Domingos Martins topographic maps - scale 1:50,000 (IBGE, 1977 and 1978) and satellite images from GoogleEarth.
Fieldwork included the description and classification of lithologies (Fettes & Desmons, 2014) and description and collection of structural data (McClay, 1991). Structural data orientation was interpreted based on Schmidt-Lambert stereograms (Hobbs et al. 1976). For the study of the GSZ, eight outcrops distributed in 16 km were described along the roads ES-185 and ES-387, between the district of Celina and the small town of Ibitirama (Figure 1). For the study of the BSZ, 7 outcrops distributed in 9.7 km were described along the road BR-262.
The electrical resistivity method has been largely used for the survey of groundwater in fractured aquifers (Gallas, 2003; Braga, 2016; Singh et al. 2019; Briški et al. 2020). Usually, fractures represent permeable zones filled with water and/or soil/chemically weathered rock. Thus, they tend to present lower values of resistivity in comparison with adjacent intact rock mass. The electrical resistivity prospecting consisted in the use of the DC Resistivity method through the electrical resistivity tomography technique in a Schlumberger electrode array, distributed along six profiles with 10 meters of electrode spacing. Six resistivity profiles with a total length of 2,480 m were surveyed. A total of 385 measurements were determined for the profiles 380 m long and 527 measurements for only one profile 580 m long. The maximum investigation depth reached up to 130 m. Geophysical survey lines were preferentially setted up in the outer margins and inner portions of the GSZ and BSZ. They are oriented parallel and perpendicular to these structures.
The geophysical equipment used was the Terrameter LS resistivity meter, manufactured by ABEM Instrument (Sweden), which consists of a single automated signal transmission and reception module, with 250 W of power, 1 μV resolution and maximum electric current of 2.5 A (ABEM 2012).
Data acquired in the field were processed in the RES2DINV computational program (Geotomo, 2003). The results comprise resistivity profiles of distance versus depth presented in logarithmic scale in which values are interpolated and displayed in intervals of colors. This program automatically determines a two-dimensional subsurface model, from resistivity or chargeability data obtained in electrical profiling tests (Griffiths & Barker 1993).
The 2D model used in the program divides the pseudo-section into rectangular blocks, which will represent the pseudo-section by adjusting the field measurements. This optimization aims to reduce the difference between the apparent resistivity values calculated and measured in the field by adjusting the resistivity of the block model. The difference between the values is expressed by the root mean squared error (RMS) and its product is represented as inversion models (Loke & Barker 1996).
In order to validate the results, an attempt was made by comparing geoelectric profiles with groundwater well production data freely available in the CPRM-SIAGAS portal at http://siagasweb.cprm.gov.br/layout/visualizar_mapa.php.
Results
Photogeological analysis of aerial stereo-pairs
Structural lineaments interpreted from aerial stereo-pairs for the GSZ are displayed in Figure 2. 1,148 lineaments were interpreted and are distributed in 120 km2. Lengths varied from 52 m to 3,866 m; average value equals to 386 m. NW and NE-trending lineaments predominate, respectively. NE-trending lineaments are concentrated in the eastern and southern portions of the study area (Figure 2), which coincides with the main trace of the GSZ. The NW portion of Figure 2 shows a distinct structural pattern in terms of orientation and lineament density (aerial photos ES-23 - 4505 and ES-23 - 4506).
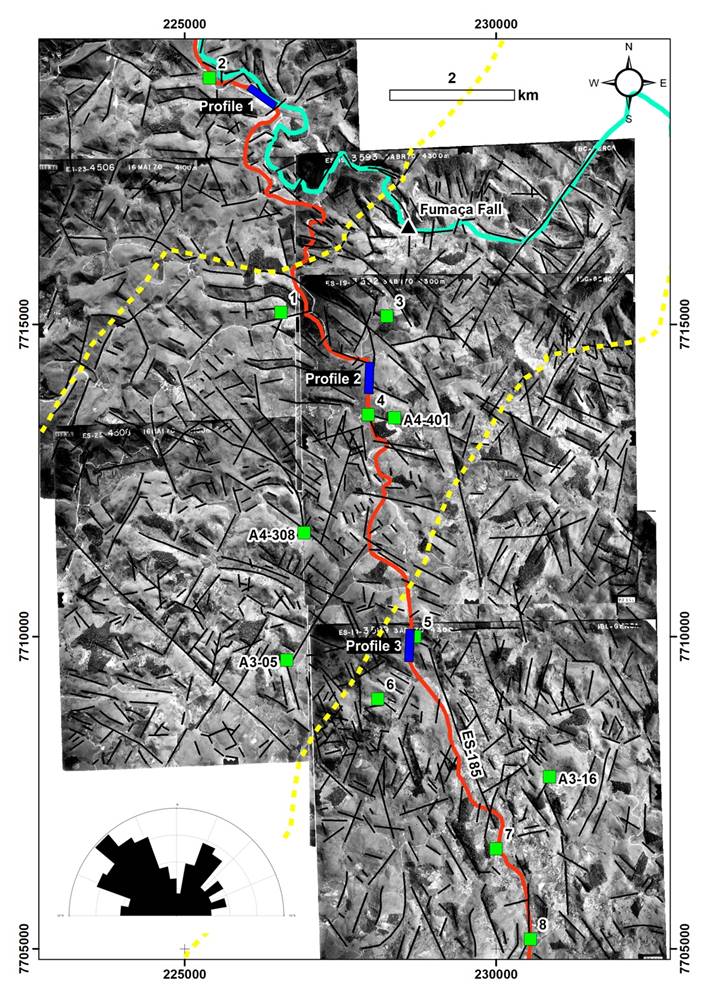
Figure 2 Structural map interpreted from aerial stereo-pairs for the BSZ with indication of the studied outcrops (labeled green boxes) and resistivity profiles (labeled blue rectangles). In the lower left corner, rose diagram display lineament orientations. Red line - roads and yellow dashed line - GSZ (after Horn, 2007).
Structural lineaments interpreted from aerial stereo-pairs for the BSZ are displayed in Figure 3. 490 lineaments were interpreted and are distributed in 78 km2. Lengths varied from 79 to 4,155 m;average value equals to 418 m. NW-trending lineaments predominate but are scattered between N295 to N335. Structural domains are not evident.

Figure 3 Structural map interpreted from aerial stereo-pairs for the BSZ with indication of the studied outcrops (labeled green boxes) and resistivity profiles (labeled blue rectangles). In the lower right corner, rose diagram display lineament orientations. Red line - road BR-262. According to Féboli (1993), the BSZ follow the Batatal river and passes through P4 and P5 rock outcrops.
Field geological and structural data
Fieldwork reveled three main lithotypes for the Guaçuí Shear Zone. In outcrop 8 occurs metagranites and in outcrops 1, 2 and 7, orthogneisses (Figure 2). In all the other outcrops are exposed mylonitic rocks (3, 4, 5, 6, A03-05, A03-16, A04-308 and A04-401). Besides, in one outcrop very close to Point 4 (UTM 24S 227154E/7713215S) a stromatic metatexite was also described. It shows a subvertical and penetrative foliation oriented parallel to the GSZ. Metagranites are coarse-grained and leucocratic rocks with weak foliation defined by mm-sized biotite crystals. Orthogneisses are course to fine-grained rocks and show remarkable cm-sized gneissic banding. Deformed mafic enclaves, few decimeters in size, and sparingly migmatized zones are also observed. Mylonitic rocks show typical cm to mm-sized mylonitic banding and numerous dextral kinematic indicators.
Figure 4 depicts stereograms of the planes of foliation along the cross-section studied. The results indicate that predominates strikes ranging from NNW to NNE and moderate to steep dip angles (45 - 64°) dipping predominantly to the east. Field observations across the GSZ revealed slightly fractured rock masses.
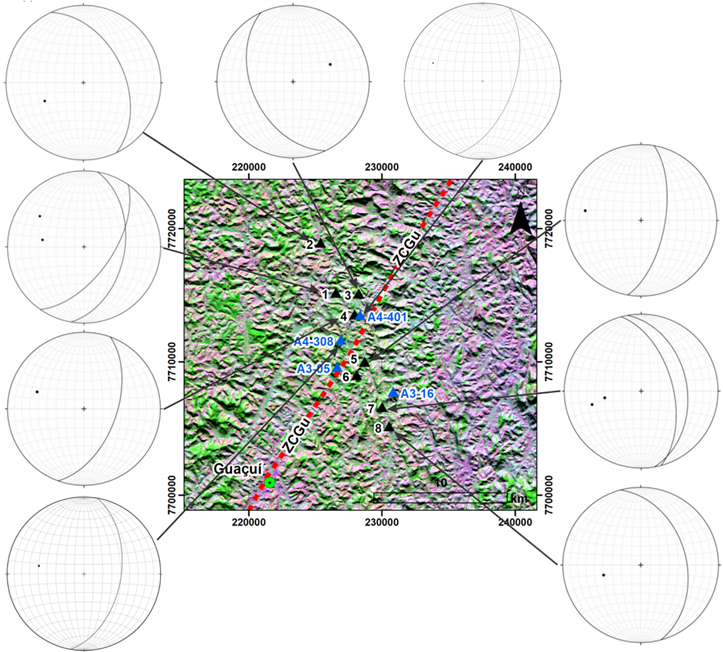
Figure 4 Stereographic projection of the planes of foliation across GSZ. Schmidt-Lambert net, lower hemisphere (equal area). Black triangles studied outcrops (this study) and blue triangles (Hartwig & Melo, 2019). Background image: Landsat-5 5R4G3B colored composition.
The transect course across the BSZ revealed coarse-grained garnet biotite gneiss, garnet metagranodiorite to metatonalite and calcisilicatic rocks. Gneissic banding is made of alternating and parallel mafic and felsic banding. Banding thickness ranges from 0.1 to 3.0 cm in hand specimens. Mafic bands are essentially made of biotite and garnet crystals and felsic bands are made of quartz, K-feldspar and plagioclase grains. A fine-grained banded calcisilicatic rock was identified in outcrop 3. Deformed mafic enclaves and sinistral kinematic indicators were observed in outcrop 6. A course-grained sillimanite-garnet gneiss was described in outcrop 7. Garnet metagranodiorites to metatonalites were recognized in outcrops 1, 2 and 5 and show a metamorphic foliation characterized by undulose films of biotite flakes (< 1 mm in diameter on average).
Figure 5 depicts stereograms of foliation along the cross-section studied. NE to NNE-trending foliation with dip angles ranging from 10 to 88° predominates. In outcrops 1, 2 and 3, a NW-trending foliation is observed with dip angles ranging from 20 to 60°. Outcrops described across the BSZ revealed slightly fractured rock masses.
Electrical resistivity tomography
Geophysical investigation depths ranged from 80 to 130 m for the Guaçuí Shear Zone (Figure 6). Resistivity profiles 1 and 3 are located in the west and east outer margins of the GSZ and resistivity Profile 2 is located within it (Figure 2). Resistivity profiles allow interpreting two geoelectrical layers. A superficial one regarded as soil/recent sedimentary deposits (containing groundwater or not) and a competent, unfractured and fresh rock mass (sound rock).
Resistivity profiles 1 and 2 show increasing values of electrical resistivity with depth ranging from approximately 117 to 16,070 Ohm.m. Resistivity profiles 1 and 2 show a saturated and discontinuous horizontal aquifer between elevations 710 and 660 m.a.s.l, exhibiting very low electrical resistivity values (< 117 Ohm.m). Resistivity Profile 3 shows a horizontal layer of very high electrical resistivity values (> 16,070 Ohm.m) between elevations 675 and 645 m.a.s.l, surrounded by moderate values of resistivity (~ 1,372 Ohm.m). The distribution pattern of the electrical resistivity isovalues under this layer may indicate the presence of a subhorizontal fracture. Lineaments interpreted from stereo-pairs are not evident in the resistivity sections of Figure 6 as disturbances in the distribution and magnitude of resistivity values are not discernable.
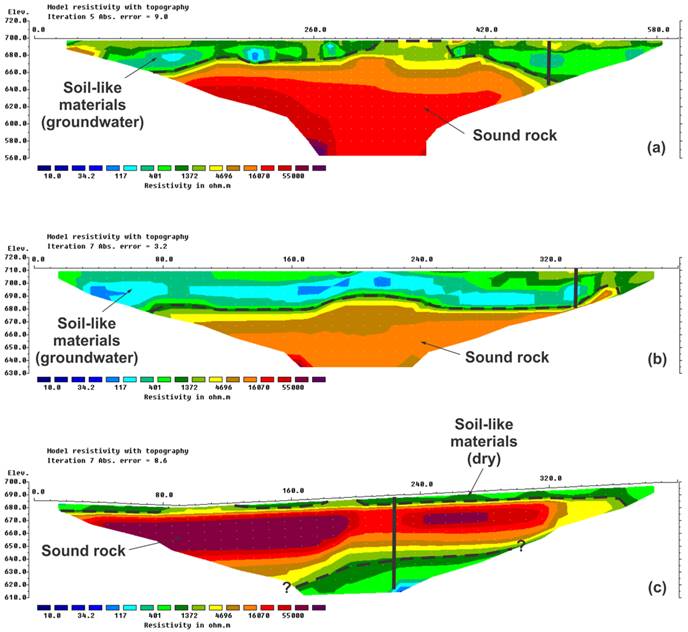
Figure 6 2D eletrical resistivity imaging pseudosections for the GSZ in Ohm.m: (a) Resistivity Profile 1; (b) Resistivity Profile 2; and (c) Resistivity Profile 3. Dashed lines refer to geological contacts and fractures interpreted directly from resistivity sections, while continuous lines refer to lineaments based on the interpretation of stereo-pairs.
Geophysical investigation depths for the Batatal Shear Zone ranged from 75 to 85 m (Figure 7). Resistivity profiles are oriented parallel and perpendicularly to the main structural trends. Resistivity profiles 1 and 3 are located in the east and west outer margins of the BSZ while resistivity Profile 2 is within it (Figure 3). Resistivity Profile 1 shows in general average values of electrical resistivity (~ 1,372 Ohm.m) that indicate highly weathered rock masses (saprolite). Between electrodes located from 160 to 210 m, a huge elliptical spot of very high electrical resistivity is observed (55,000 Ohm.m) from elevations 515 to 485 m.a.s.l. Very high values of electrical resistivity indicate sound rock. Resistivity Profile 1 also indicates an irregular shaped layer (probably dry soil) dipping gently to the left.

Figure 7 2D eletrical resistivity imaging pseudosections for the BSZ in Ohm.m: (a) Resistivity Profile 1; (b) Resistivity Profile 2; and (c) Resistivity Profile 3. Dashed lines refer to geological contacts and fractures interpreted directly from resistivity sections, while continuous lines refer to lineaments based on the interpretation of stereo-pairs.
Resistivity Profile 2 shows two regions of low values of electrical resistivity that indicate saturated soil/recent sedimentary deposits, separated by a central portion of high electrical resistivity values (> 4,696 Ohm.m). The vertical lineament interpreted from the stereo-pairs in the central portion of the section does not produce significant changes in the distribution pattern of resistivity isovalues.
Resistivity Profile 3 shows a superficial horizon of saprolite (1,372 Ohm.m) underlaid by sound rock (4,696 Ohm.m). Two spots of very high electrical resistivity values are observed (> 16,070 Ohm.m) between electrodes located at 180-210 m and 300-370 m. The central portion of resistivity Profile 3 also exhibits a distribution pattern of electrical resistivity values that suggests the presence of subvertical geological structures.
Discussions
The geological interpretation of remote sensed data revealed that NW and NE-trending lineaments are the most important structures. NE-trending lineaments coincides with the orientation of ductile structures of the Precambrian crystalline basement rocks. NW-trending structures are associated to the opening and evolution of the South Atlantic Ocean (Calegrari et al., 2016; Lourenço et al., 2016). Hartwig and Bozzi (2019) recognized more than one hundred NW and NE-trending fractures in the semi-consolidate Miocene sediments of the Barreiras Formation in the southern margin of the Espírito Santo State showing that both sets were reactivated in recent times. For BSZ, the lineaments orientations are more scattered in comparison with the GSZ (Figures 2 and 3). Remote sensed data have also shown that the GSZ (9.5 lineaments/km2) is more structured than the BSZ, (6.3 lineaments/km2). Moreover, the GSZ is more strongly controlled by NE-trending lineaments.
Geological and structural field data revealed poorly fractured rock masses. Some fractures identified in the field are related to rock blasting and does not have hydrogeological significance. Foliations are predominantly oriented from NNW to NE for both shear zones. The GSZ shows a penetrative milonitic foliation while for the BSZ rocks show a weak metamorphic foliation with significant variations in dip angles.
Geophysical surveys clearly revealed a superficial porous aquifer in both shear zones, which is associated to low values of resistivity. Although the GSZ present numerous geological lineaments when viewed from stereo-pairs, resistivity sections have not confirmed their subsurface expression. One possible explanation for that may be due to their limited lengths. The low values of resistivity that appear bellow elevation 640 m.a.s.l. in Figure 6c seems to be related to exfoliation joints. These are widely regarded as forming in response to removal of overburden, associated to landform evolution (Hartwig, 2006; Hobbs et al. 1976, Bahat et al. 1999).
Abrupt changes in the orientation of the resistivity isolines indicate a fractured rock mass for the BSZ (Figure 7c). However, these structures seem to be related to sealed (closed) fractures as high resistivity values (> 4,696 Ohm.m) predominate elsewhere. Telford et al. (1990) present examples of electrical resistivity values for common rocks and minerals. Considering geological fieldwork data, it is possible to interpret that the distribution and magnitude patterns of resistivity values observed in Figure 7c may be related to faciological differences in crystalline basement rocks.
According to Costa (2008) and Fernandes (2008) not only the degree of fracturing, but also the principal horizontal stress orientation control groundwater storage and flow in fractured aquifers. Ribeiro (2010) studied the brittle tectonics of the Barreiras Formation in the southern margin of the Espírito Santo state and recognized NW-oriented sinistral strike-slip faults. According to the author, they were originated by extensional stress-oriented NE-SW, associated to a Quaternary E-W-trending dextral strike-slip tectonic regime. Therefore, if this state of stress was still active, groundwater would be more likely retained in NW-trending lineaments.
Groundwater production data from tube wells is available only for the BSZ (Table 1). Ten groundwater wells are distributed in Domingos Martins urban area (Figure 5). Well production data is available for only five of them. Flow rates for these water wells ranged from 0.4 to 19.8 m3/h. It is worth mentioning that all groundwater wells lay over a long NW-trending lineament (Figure 5). However, information regarding the geology crossed by these wells and filter positions was not provided.
Table 1 Well production data for the BSZ. Source: CPRM-SIAGAS.
Well codes | Locality | E (m) | N (m) | Flow rate (m3/h) |
---|---|---|---|---|
3100016741 | Campinho- Domingo Martins | 326,741.2 | 7,747,191.8 | 19.8 |
3100016744 | Campinho-Domingos Martins | 326,971.61 | 7,747,347.9 | 0.4 |
3100016745 | Campinho-Domingos Martins | 326,970.99 | 7,747,409.45 | 6 |
3100016746 | Campinho- Domingos Martins | 327,03028 | 7,747,287.05 | 2.8 |
3100016747 | Campinho-Domingos Martins | 327,001.87 | 7,747,225.2 | 3 |
Figure 8 summarizes rock mass conceptual models for groundwater prospection for both the GSZ and the BSZ. A potential superficial porous aquifer is regarded to unconsolidated sedimentary deposits and/or soil cover (regolith). This are concentrated in the first 30 meters below surface and is well developed in the GSZ (Figures 6a, b). Although it is easily exploited for human consumption, it is also very vulnerable to pollution, especially from wastewater (Bertossi et al. 2013). Poorly fractured rock masses are associated to the GSZ and fractured rock masses were recognized for the BSZ (Figure 7c). Nevertheless, discontinuities seem to be sealed (closed) as high resistivity values (> 4,696 Ohm.m) predominate elsewhere.
Conclusions
The aim of this paper was to investigate the hydrogeological significance for groundwater prospecting of two Precambrian NE-trending shear zones, located in the southern region of the Espírito Santo State (Southeastern Brazil).
Based on the results, a porous near-surface aquifer has good potential for groundwater storage, especially in the GSZ. The GSZ and the BSZ are intercepted by numerous lineaments preferentially oriented to NE-NNE and NW-NNW. The former is associated to ductile Precambrian tectonics (Araçuaí-West Congo Orogen) while the latter to Meso-Cenozoic brittle tectonics. However, integrated data is not conclusive about the hydrogeological significance of brittle and ductile discontinuities for groundwater prospection. The resistivity profiles and available water well data did not confirm the occurrence of groundwater associated with them, which may be due to the amount of surveyed data, state of stress, etc. Authors recommend more investigations in the north and south sectors of the GSZ and the BSZ in order to better understand the hydrogeological significance of these geological features.