Highlights:
Stoichiometry of Pinus hartwegii was analyzed in Jocotitlán (JO) and Tláloc (TL) mountains.
Needle litterfall was higher (11.2 Mg∙ha-1∙year-1) in the less productive forest (Jocotitlán).
N, P, K, Ca and Mg flux was, respectively, 77, 54, 12, 70 and 19 % higher in JO than in TL.
Nutrient flux in needle litterfall was significantly seasonal.
Mg dynamics and N:Mg and N:K ratios in TL were more suitable for tree growth.
Introduction
Leaf litterfall in forests represents the main pathway for nutrient transfer and return to the soil (Sayer et al., 2020); in turn, leaf mass on forest floor (litter) represents the key reservoir for gradual release of nutrients into mineral soil. Seasonal physiological activity of trees, leaf longevity and climate influence leaf fall (Gavinet, Ourcival, & Limousin, 2019), which results in a seasonal flux of nutrients, characteristic for each ecosystem and its successional phase. However, alteration of carbon and nitrogen cycles can lead to fertilization processes by increasing atmospheric CO2 in forests, stimulating growth of leaf mass and modifying its chemical composition, but its effect will depend on the progressive limitation of nutrients (González de Andrés, 2019; Silva, Gómez-Guerrero, Doane, & Horwath, 2015). Although locally, soil fertility and forest microclimate influence the levels of leaf fall and its nutrients (Cheng, Lee, Lee, Lee, Chen, & Menyailo, 2020; Wood, Lawrence, & Clark, 2006), indirectly, global climatic events such as El Niño-Southern Oscillation (ENSO) and the North Atlantic Oscillation (NOA) geographically affect the mass and stoichiometry of litterfall (González de Andrés et al., 2019).
The study of nutrient flux via litterfall is limited and requires further research worldwide (Neumann et al., 2018). Mexico has few studies on the dynamics of leaf mass and nutrient concentration in forests. Some have been conducted in the central and northern mesa of Mexico, in pine-oak forests and shrublands with semi-dry climates and elevations lower than 2 800 m (González-Rodríguez et al., 2018; Pérez-Suárez, Arredondo-Moreno, Huber-Sannwald, & Vargas-Hernández, 2009); however, information in alpine forests, ecosystems particularly vulnerable to climate change, is still missing. Consequently, we rely to the experience of studies carried out in other parts of the world to explain the behavior of our forest areas. Due to the above, and considering that climate variation influences each region differently, studies in representative ecosystems of the country are needed to know how forest ecosystems will respond to these changes (Gómez-Guerrero & Doane, 2018).
As a result of climate change, variations in leaf mass production and nutrient flux in forests are expected (Gómez-Guerrero & Doane, 2018). The predicted increase in temperature could result in water stress and changes in nutrient distribution in vegetation reservoirs (Neumann et al., 2018). Therefore, it is important to have baseline studies on nutrient dynamics in forest ecosystems to assess their future changes (Ge & Xie, 2017).
The objective of this study was to evaluate the annual needle production, concentration and mass of nutrients (N, P, K, Ca and Mg) in alpine forests of Pinus hartwegii Lindl. of Jocotitlán (JO) and Tláloc (TL) mountains in Estado de México. This study fills the lack of information on nutrient dynamics in alpine forests and establishes a baseline to measure changes that may occur as a response of ecosystems to climate change. The information generated is also useful for modeling biogeochemical processes in forest vegetation.
Materials and Methods
Areas of study
This study was carried out in Jocotitlán (JO) and Tláloc (TL) mountains in Estado de México (Figure 1). These mountains are part of the Transmexican Volcanic Belt geologically composed of an andesite-dacite complex and pyroclastic deposits (Arce et al., 2019). The climate is humid semi-cold type C(w2)(w), with summer rainfall and less than 5 % of the rainfall occurs in winter, with precipitation/temperature ratio greater than 55 (Garcia, 2004). Mean annual precipitation varies from 900 to 1 200 mm with mean annual temperature between 5 and 10 °C. The forest stand in JO is composed on average by 180 trees∙ha-1 with a basal area of 14 m2∙ha-1 (Núñez-García, Gómez-Guerrero, Terrazas-Salgado, Vargas-Hernández, & Villanueva-Díaz, 2020), while in the case of TL, it is formed by 250 trees∙ha-1 and 27 m2∙ha-1 (Acosta Mireles, Carrillo Anzures, Delgado, & Velasco Bautista, 2014). The increment in basal area of trees in TL is on average 2.5 greater than in JO (Correa‐Díaz et al., 2020; Núñez et al., 2020).
After open-field surveys and review of cartographic and satellite material, four periodic collection sites for needle litterfall were established on each mountain. To capture the variation of each mountain, we included collection sites contrasting in elevation and aspect, but with pure stands of P. hartwegii. Site locations are shown in Table 1.
Table 1 Sites used in periodic collection of Pinus hartwegii needles in the mountains of Jocotitlán and Tláloc, Estado de México.
Mountain | Site | Elevation (m) | Exposure | Latitude (°) | Lenght (°) |
---|---|---|---|---|---|
Jocotitlán | NO-3800 | 3 800 | Northwest | 19.7423 | -99.7645 |
SO-3800 | 3 800 | Southwest | 19.7412 | -99.7662 | |
NO-3700 | 3 700 | Northwest | 19.7427 | -99.761 | |
SO-3700 | 3 700 | Southwest | 19.7397 | -99.768 | |
Tláloc | NO-3900 | 3 900 | Northwest | 19.424 | -98.7245 |
NO-3500 | 3 500 | Northwest | 19.4161 | -98.7258 | |
SO-3900 | 3 900 | Southwest | 19.7255 | -98.7255 | |
SO-3500 | 3 500 | Southwest | 19.4045 | -98.7419 |
Needle mass
Needle mass (kg∙ha-1) was quantified in a 20 m transect with three 30 cm diameter litter traps, placed at ground level and delimited with 127 mm thick polyduct, at each site. The distance between litter traps was 10 m, placed along the contour line of the terrain. A total of 12 litter traps were established on each mountain (three replicates x four collection sites).
To identify freshly deposited material, all the mulch within the circle was removed until mineral soil was exposed, allowing the contrast between the black color of the soil and the brown color of the needles to facilitate the identification of recently fallen material. All litter traps were placed under tree canopy, aiming for coverages of at least 60 %. The cumulative needles were collected during 9 (JO) and 10 (TL) visits from June 2017 to June 2018. Needles were dried using a forced circulation air oven (Sheldon Fx14-S, USA) at 70 °C for 72 h and weighed on a digital balance (Ohaus Scout Pro, USA) with an accuracy of 0.01g.
Leaf litterfall nutrients
A total of three leaf litterfall samples were taken at each site (12 per mountain) and concentrations of N, P, K, Ca and Mg were analyzed to estimate the nutrient reservoirs in the forest floor, at the beginning of the experiment. In the same way, and to correlate nutrient content of litterfall with that of the forest floor and the mineral soil, concentrations of N and P and exchangeable cations K+, Ca+2 and Mg+2 of the surface soil (0 to 10 cm) were determined, taking 36 samples in JO and 40 in TL during the year of observation.
An electronic temperature and relative humidity recorder (Datalogger, HoBo®, USA) was installed at each site to obtain data at 4 h intervals and to know the variation under climate conditions of each mountain.
Sample analysis
Dry samples of litter and needles were ground for nutrient concentration analysis. N in plant tissue was determined by the semi-micro-Kjeldahl method (Bremmer, 1965). P, K, Ca and Mg concentrations were determined in extract resulting from digestion with HNO3:HClO4 (2:1, v:v) of dried and ground plant tissue with an inductively coupled plasma atomic emission spectrophotometer (ICP-OES 725 Series, Agilent) (Alcántara & Sandoval, 1999). The pH of the mineral soil samples was determined with a potentiometer in a 1:2 soil-water ratio. Total nitrogen was also determined by the micro-Kjeldahl method, P by the Bray and Kurtz (1945) method and exchangeable cations with 1 N ammonium acetate pH 7.0.
Statistical analysis
The Shapiro-Wilk test was used to verify that the data complied with the normality assumption, and when this was not the case, logarithmic, square root or Box-Cox transformations were used. When normal distribution was not possible by transformations, the nonparametric Wilcoxon test (Zar, 2010) was used for comparisons between mountains. Nutrient mass (kg∙ha-1), concentration (%, ppm or cmolc∙kg-1) and fluxes (kg∙ha-1∙year-1) were the response variables for intermountain comparison. Ratios of nutrients to N were also used, which is a common reference for this type of study (Sardans et al., 2016). For fluxes, a statistical analysis of trends was performed, because samples were repeated over time at each site; therefore, measurements are correlated over time. A longitudinal analysis of variance was performed using linear mixed models regarding time, mountain and time*mountain interaction as fixed factors (Fitzmaurice, Laird, & Ware, 2004). We tested whether time trends followed a linear, quadratic or cubic pattern (P > 0.05). Analyses were performed using the statistical packages SAS (SAS Institute Inc., 2011) and R (3.6.3) (R Core Team, 2020).
Results
According to Table 2, forest floor chemical characteristics (N, P, K and Ca) between both mountains were similar, except for Mg concentration which was 37 % higher for TL (P > 0.0001). Ca concentration was significantly higher by 11 % in TL (P < 0.09). Although mean K concentration is 54 % higher for TL, the difference was not significant between mountains.
Table 2 Forest floor nutrient concentrations in two alpine Pinus hartwegii forests in central Mexico.
Site | TN | P | K | Ca | Mg |
---|---|---|---|---|---|
(%) | --------------------(ppm)-------------------------- | ||||
Jocotitlán | |||||
Mean | 0.8 | 535.5 | 722 | 2 292.4 | 491 |
SD | 0.2 | 94.6 | 205.3 | 335.6 | 106.6 |
CV (%) | 21 | 17.7 | 28.4 | 14.6 | 21.7 |
n | 12 | 12 | 12 | 12 | 12 |
Tláloc | |||||
Mean | 0.9 | 548.5 | 1 112.4 | 2 540.6 | 674.9 |
SD | 0.2 | 81.1 | 575 | 417.8 | 88.1 |
CV (%) | 27.8 | 14.8 | 51.7 | 16.4 | 13.1 |
n | 12 | 12 | 12 | 12 | 12 |
P< | ns | ns | ns | 0.0941 | 0.0001 |
TN = total nitrogen; SD: standard deviation of the mean; CV: coefficient of variation. P< value indicates statistical difference between mountains according to the Wilcoxon test; ns = not significant.
Table 3 shows that the mineral soil is very acid (pH ≈ 5.2) in both mountains. Total N, extractable P and exchangeable K+ are statistically equal between mountains. Statistical differences were reflected in Mg, Ca and the sum of cations (K+ + Ca+2 + Mg+2) with higher values in TL (P < 0.021).
Table 3 Surface soil nutrient concentrations (0-10 cm) in two alpine Pinus hartwegii forests in central Mexico.
Mountain | pH (1:2) | TN (%) | P (ppm) | Exchangeable cations (cmolc(+)∙kg-1) | |||
---|---|---|---|---|---|---|---|
K+ | Ca+2 | Mg+2 | Sum | ||||
Jocotitlán | |||||||
Mean | 5.3 | 0.4 | 3.3 | 1.2 | 2.5 | 0.2 | 3.9 |
SD | 0.9 | 0.2 | 1.8 | 1.3 | 2 | 0.1 | 1.3 |
CV (%) | 16.5 | 42.1 | 53.1 | 106.8 | 81.2 | 55.2 | 34.1 |
n | 36 | 36 | 36 | 36 | 36 | 36 | 36 |
Tláloc | |||||||
Mean | 5.1 | 0.4 | 3.4 | 1.4 | 3.6 | 0.24 | 5.2 |
SD | 0.5 | 0.1 | 2.2 | 1.6 | 2.1 | 0.3 | 1.3 |
CV (%) | 10 | 32.8 | 64.7 | 108.1 | 60.2 | 129.9 | 25.4 |
n | 40 | 40 | 40 | 40 | 40 | 40 | 40 |
P< | ns | ns | ns | ns | 0.0001 | 0.0211 | 0.0001 |
TN = total nitrogen; SD: standard deviation of the mean; CV: coefficient of variation. P< value indicates statistical difference between mountains according to the Wilcoxon test; ns = not significant.
Table 4 shows that both the mass of the forest floor and the mass of its nutrients were statistically higher in JO (P < 0.04). Forest floor mass in JO (46.5 Mg∙ha-1) was 175 % higher than that in TL (16.9 Mg∙ha-1). The mass of nutrients of forest floor was greater in JO with higher values of 164, 181, 60, 164 and 118 % for N, P, K, Ca and Mg, respectively.
Table 4 Forest floor (mulch) and nutrient mass in two alpine forests of Pinus hartwegii in central Mexico.
Site | Mass | N | P | K | Ca | Mg |
---|---|---|---|---|---|---|
(Mg∙ha-1) | -------------------------(kg∙ha-1)------------------------- | |||||
Jocotitlán | ||||||
Mean | 46.5 | 360.6 | 24.7 | 34.5 | 106.8 | 23.8 |
SD | 21.4 | 194.4 | 11.5 | 18.3 | 53.2 | 12.7 |
CV (%) | 46 | 53.9 | 46.5 | 53.2 | 49.8 | 53.4 |
n | 12 | 12 | 12 | 12 | 12 | 12 |
Tláloc | ||||||
Mean | 16.9 | 136.7 | 8.8 | 21.5 | 40.5 | 10.9 |
SD | 19.1 | 151.7 | 10.5 | 31.7 | 43.4 | 12.6 |
CV (%) | 113.2 | 111 | 119.5 | 147.8 | 107.1 | 115.3 |
n | 12 | 12 | 12 | 12 | 12 | 12 |
P< | 0.0014 | 0.0001 | 0.001 | 0.0404 | 0.0003 | 0.0141 |
SD: standard deviation of the mean; CV: coefficient of variation. P< value indicates statistical difference between mountains according to the Wilcoxon test.
According to Table 5, needle mass and nutrient flux was statistically higher in JO (P < 0.04). The needle mass transfer from the canopy to the forest floor was 67 % higher in JO than in TL. In terms of mass nutrient flux, differences were 77, 54, 12, 70 and 19 % higher in JO with respect to TL, for N, P, K, Ca and Mg, respectively.
Table 5 Annual needle and nutrient mass flux via litterfall in two alpine forests of Pinus hartwegii in central Mexico.
Site | Mass | N | P | K | Ca | Mg |
---|---|---|---|---|---|---|
(Mg∙ha-1∙year-1) | -----------------------(kg∙ha-1∙year-1)------------------- | |||||
Jocotitlán | ||||||
Mean | 11.2 | 98 | 5.2 | 8.7 | 24.6 | 5.6 |
SD | 3 | 20.9 | 1.1 | 2.6 | 6.8 | 1.9 |
CV (%) | 26.8 | 21.3 | 23.1 | 30.7 | 27.7 | 33.4 |
n | 12 | 12 | 12 | 12 | 12 | 12 |
Tláloc | ||||||
Mean | 6.7 | 55.3 | 3.4 | 7.8 | 14.4 | 4.7 |
SD | 3.4 | 28.3 | 1.8 | 6.6 | 5.8 | 2.2 |
CV (%) | 50.7 | 51.2 | 54.3 | 85.2 | 40.2 | 46.9 |
n | 12 | 12 | 12 | 12 | 12 | 12 |
P< | 0.0027 | 0.0001 | 0.001 | 0.0404 | 0.0003 | 0.0141 |
SD: standard deviation of the mean; CV: coefficient of variation. P< value indicates statistical difference between mountains according to the Wilcoxon test.
Seasonal trends
The most common seasonal trend was cubic. K concentrations showed no definite trend, except when the N:K ratio was considered. Table 6 indicates that, in all cases where a trend could be fitted, the factor time was significant (P < 0.001), while the mountain effect was not statistically significant for P, Ca concentrations and the N:Ca ratio. The mountain*time interaction was statistically significant for N and the N:K and N:Mg ratios.
Table 6 Statistical significance of longitudinal analysis of variance of temporal trends of needle mass and nutrient concentration in two alpine forests of Pinus hartwegii in central Mexico.
Variable | Trend | Time | Mountain | Time*mountain interaction |
---|---|---|---|---|
Needle mass | Quadratic | <.0001 | <.0001 | 0.0443 |
N | Cubic | 0.0002 | 0.0002 | 0.0153 |
P | Quadratic | <.0001 | 0.8172 | ns |
K | No trend | --- | --- | --- |
Ca | Quadratic | <.0001 | 0.7372 | ns |
Mg | Cubic | 0.0322 | <.0001 | ns |
N:P | Cubic | 0.0007 | 0.0058 | ns |
N:K | Cubic | <.0001 | <.0001 | 0.0007 |
N:Ca | Quadratic | <.0001 | 0.2002 | ns |
N:Mg | Cubic | <.0001 | <.0001 | 0.0024 |
For this research, during the one-year cycle, the fact that the trend of nutrients or proportions is quadratic indicates that there is a low point for a given season, while when it is cubic there is a seasonal maximum and minimum; that is, two changes of slope in the trend.
Figure 2 shows that the trend of needle litterfall was higher during summer and lower in winter. The trends by nutrients and ratios regarding N are shown in Figures 3 and 4, respectively. N concentration was higher in mid-fall (October 17), while for P and Ca it was higher in summer (June 20 to July 7). For Mg, concentrations were higher in spring (March 16 to May 5) (Figure 3). Except for the N:Ca ratio, most of the nutrient ratios (N:P, N:K, N:Mg) had maxima at the end of summer (August 28) and autumn (October 17) (Figure 4).

Figure 2 Temporal trends in Pinus hartwegii needle litterfall mass with estimated values and 95 % confidence intervals in two alpine forests of central Mexico. Lowercase and uppercase letters indicate months of 2017 and 2018, respectively.
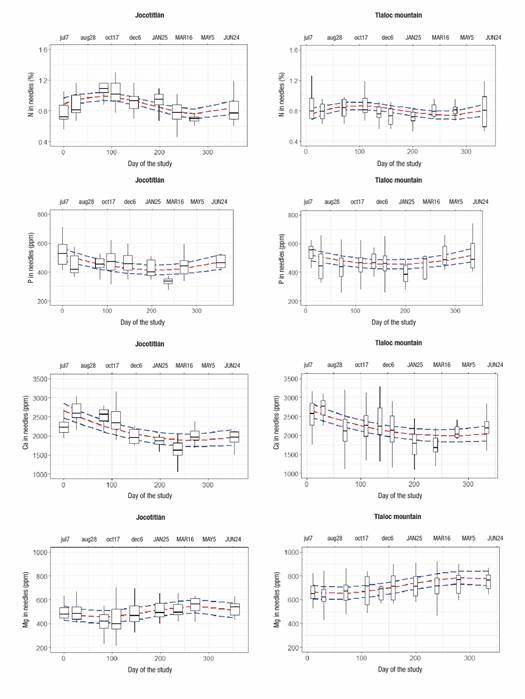
Figure 3 Trends in needle litterfall nutrient concentration of Pinus hartwegii with 95 % confidence intervals in two alpine forests of central Mexico. Lowercase and uppercase letters indicate months of 2017 and 2018, respectively.
Discussion
Forest floor and soil concentrations
Compared to JO, the forest floor in TL had higher concentrations of Mg (P < 0.001) and Ca (P < 0.009). That difference was also reflected in mineral soil concentrations, confirming the close relationship of soil fertility and needle chemistry (Wood et al., 2006). A higher sum of cations (K + Ca + Mg) in TL indicates better fertility and reinforces the relationship between the amount of potentially available nutrients and the chemical composition of needles, considering that there is the same volcanic soil (Andisol) in both mountains. In these, the low concentration of the main cations (<6 cmolc∙kg-1) is expected in forest soils of P. hartwegii forests in central Mexico (Marín, Escolero-Fuentes, & Trinidad-Santos, 2002).
Needle mass
The fact that JO had a larger amount of mulch mass also translated into larger reservoirs of all nutrients (P < 0.001); however, it is important to note that even with larger reservoirs in JO, TL forest has higher productivity. Trees in JO have a mean annual increase in basal area of 6 cm2, while in TL it is 15 cm2 (Correa‐Díaz et al., 2020; Núñez-García et al., 2020).
The results indicate that a high proportion of the net productivity goes to leaf mass production in JO. Depending on forest structure and age, needle production can account for more than 70 % of canopy litter (Schlesinger & Bernhardt, 2013). Annually, greater amounts of nutrients flow from the canopy to the forest floor in JO, but there does not seem to be a priority in the production of timber components. In fact, the synchrony between photosynthetic activity at the canopy level (through vegetation indices- NDVI) and annual growth ring formation is higher in TL than in JO (Correa-Díaz, Gómez-Guerrero, Vargas-Hernández, Rozenberg, & Horwath, 2020).
Other authors have also found no correlation between radial growth and needle production in Pinus silvestris L. forests (Lehtonen, Lindholm, Hokkanen, Salminen, & Jalkanen, 2008), but have found pulses of needle production every four years, possibly associated with global climatic circulation events (González de Andrés et al., 2019). As for larger forest floor reservoirs, what is known is that litterfall accumulation is rather correlated with decomposition processes than with the forest yield (Frank, Pontes, & McFarlane, 2012). The results of this study suggest that there may be other factors of needle quality and decomposition, such as N:lignin ratio, that could explain this result (Schlesinger & Bernhardt, 2013).
Being an isolated volcano and not part of a mountain range like TL (Figure 1), it is likely that JO may have more extreme humidity and temperature events and higher wind speed that cause more needle litterfall, less decomposition and less tree growth (Zhu et al., 2021). Dataloggers data showed that temperature is slightly lower and relative humidity is higher in JO than in TL (6.83 vs. 7.25 °C; 73.13 vs. 69.74 %).
Annual nutrient flux
Both mountains have clear trends in needle and nutrient mass between June of the previous year (2017) and June of the subsequent year (2018), with lower values in early winter. Total pine needle input was 11.2 Mg∙ha-1∙year-1 for JO, while for TL it was 6.7 Mg∙ha-1∙year-1 (Table 5). These data are high compared to those reported in other ecosystems; for example, 7.6 Mg∙ha-1∙año-1 for total litterfall (leaves, branches and reproductive structures) in pine-oak forests of Chiapas (Rocha-Loredo & Ramírez-Marcial, 2009); 5.9 Mg∙ha-1∙year-1 in pine-oak forests of Durango (Moreno Valdez et al., 2018) and 7.4 and 10.6 Mg∙ha-1∙year-1 in oak forests of Nuevo León and Morelos, respectively (González-Rodríguez et al., 2011; Mantero-García, Gómez-Guerrero, Gavi-Reyes, Zamora-Morales, & Ramírez-Ayala, 2019). According to a global review, Zhang, Yuan, Dong, and Liu (2014) determined that total litterfall in forest ecosystems ranges from 3 to 11 Mg∙ha-1∙year-1, although the upper extremes extend to 13 Mg∙ha-1∙year-1.
In years with moisture and years with strong winds, leaf litterfall can be more than twice as high compared to average climate years, which particularly affects coniferous forests with higher needle litterfall (Cheng et al., 2020). Due to the lack of meteorological stations, it was not possible to know if in the study year there was higher leaf litterfall due to abiotic factors. Leaf litterfall values represent sites with good tree cover (>60 %), but in sites with lower cover the values will be lower. Although all sites have 100 % understory cover, it is also likely that due to the location of the trap at ground level some needles may be captured by the effect of gravity.
Maximum needle accumulation was found in the months with the highest precipitation (Figure 2), for both mountains, when growth activity is higher, trees replace old foliage and mobile nutrients such as N and P are transported from old to new tissue (Schlesinger & Bernhardt, 2013). These results contrast with those of other studies, where higher leaflitter production is associated with the driest season of the year (López-Escobar et al., 2018); however, a bimodal trend, including dry and wet season was reported for oak-pine forests (Quercus potosina Trel. and Pinus cembroides Zucc.) in San Luis Potosí, with total leaflitter production of 5.9 Mg∙ha-1∙year-1 (Pérez-Suárez et al., 2009). The results of this study indicate a single peak of needle production occurring in summer (Figure 2). Thus, in spite of developing in cold climates, the results show that the forests studied are very productive in needles and dynamic in nutrient transfer.
Nutrient use efficiency
Using the information in Table 5, nutrient use efficiency in needle litterfall can be estimated at the ecosystem level. Such an estimate is the ratio of the annual flux of needle mass to the annual flux of each nutrient (kg∙ha-1∙year-1). The efficiencies in JO are 114, 2 153, 1 287, 485, 2 015 for N, P, K, Ca and Mg, respectively, while for TL they are 121, 1 971, 859, 465, 1 425 in the same order of nutrients. These results indicate that N use efficiency is higher in TL mountain, because the same unit of nutrient (kg) translated into higher amounts of leaf mass (kg). But in terms of K and Mg there was more efficiency in JO, which is particularly consistent with a higher sum of cations in the TL soil (Table 3). Regardless of locality, these efficiency levels are higher for the bases K, Ca and Mg than those found in a stand of Pinus pseudostrobus Lindl. mixed with deciduous species (González-Rodríguez et al., 2011) and, except for P efficiency, also exceed the nutrient efficiency of the tropical rainforest (Kotowska, Leuschner, Triadiati, & Hertel, 2016).
Trends over time
Needle production is reduced in the cold months, reflecting low physiological activity in trees, for both mountains. For alpine forests this is an expected result because temperature limits several ecophysiological processes (Körner, 2012). Nutrient concentrations in needle litterfall are the result of concentrations in green needle and resorption (Kobe, Lepczyk, & Iyer, 2005); the lower the concentration of nutrients in needle litterfall, the higher the amount of resorption into trees. Both mountains have a similar pattern with lower concentrations (higher resorption) in the months from March to May (Figure 2); that is, in the dry months when nutrient supply via soil is low due to lower soil moisture (Gómez-Guerrero & Doane, 2018).
P showed a gradual increase in reuse from July to January, i.e., greater demand as the rainy season moved away (Figure 3). This could be because P is a low mobility element absorbed mainly by mass diffusion, requiring soil moisture to favor the presence of soluble forms (Binkley & Fisher, 2020). The similarity of the N:P ratio indicated a higher reuse in the months of June and July for both mountains; subsequently, this reuse decreased in winter and was reactivated in late spring of the following year, which is a trend coupled with the rate of tree growth and denotes the importance of these two macroelements (N and P) for biomass formation (Schlesinger & Bernhardt, 2013).
Ca indicated a progressive demand from July to March, which reflects the process of cell wall formation in needles; that is, unlike other elements, an accumulative process is inferred as leaf expansion is concluded. The variation in the N:Ca ratio indicates that the highest Ca consumption is found during the rainy season, when needles begin to expand. The fact that this ratio remains stable during the rest of the year indicates that Ca has low mobility and that it accumulates in support structures such as the cell wall.
The lower Mg concentrations in JO are consistent with lower soil availability (Table 3). The results indicate that, in JO, the needles that fall to the ground already have low Mg concentrations, due to low availability in the soil, which conditions trees to increase Mg reuse. For TL, on the other hand, the soil has a better supply of Mg, so that needles return to the soil with higher Mg concentrations (Figure 3). Since TL productivity is high compared to JO, the results indicate enough Mg supply and less need for reuse; in addition, the N:Mg ratio is more stable in TL throughout the year. The lower N:Mg ratio in TL indicates a better nutrient balance, supported by higher soil fertility (Table 2). Although at both sites the N:Mg ratio varies during the year, it is evident that changes are greater in JO, where needles fallen from August to October were significantly reduced in Mg (higher N:Mg ratio), indicating that JO needs further modification in nutrient ratio to maintain tree growth.
K alone showed no temporal trend, but when the N:K ratio is considered, the importance of K and its greater amplitude of response is remarkable. N:K ratio was expressed in two phases; the first from August to December (summer-autumn) when proportionally K is low in relation to N, while the second phase occurs in the dry months, from January to May (winter-spring), when K:N ratio increases (N:K decreases). Since K is linked to stomatal regulation, phloem transport and photosynthesis, this result could indicate an increase in K retranslocation in autumn, prior to cold season, while in spring K retranslocation decreases, when new growing and photosynthesis season is about to start. On the other hand, before winter, leaves have already accumulated cellulose and N is diluted, resulting in lower N:K ratios before new growing season starts (Schlesinger & Bernhardt, 2013). The role of K is complex, at an ecosystem level, but a comprehensive review of the subject indicates a strong relationship with N (Tripler, Kaushal, Likens, & Walter, 2006), which is in complete agreement with this study. In this regard, it has been proposed that parallel pulses of K and nitrate in watershed streams indicate the strong relationship of these elements (Tripler et al., 2006). Although the mean temperature of both sites is similar, days with temperatures below freezing are greater in JO (Núñez-García et al., 2020). In fact, there is a more marked pulse of K retranslocation, indicated by N:K ratio for JO, which coincides with higher frequency of frost at this site (Núñez-García et al., 2020).
Conclusions
The results of this study are a useful baseline for detecting changes in future functionality of alpine forests. The forests of Jocotitlán and Tláloc mountains differed in needle production and nutrient flux. The Jocotitlán forest, less productive in timber components (e.g., basal area), had higher needle litterfall. The N:K ratio in needle litterfall is more relevant than previously thought, as it showed more marked seasonal variation, highlighting the importance of the balance of these macronutrients in the canopy. Climate change could lead to nutritional and water stress in forests, therefore, studies on leaf litterfall production and its chemical composition can be important indicators to assess effects on vegetation and propose conservation strategies. The stoichiometry of leaf litterfall provides crucial information for understanding biogeochemistry of alpine forests.