Introduction
The Mexican Long-nosed bat (Leptonycteris nivalis) is one of the three nectarivorous migratory species in Mexico and the largest bat within the guild in the American continent. It is considered a relatively rare and scarce species (Arita and Humphrey 1988), and presents a complex biology, therefore, it is a species difficult to study. Despite the lack of information regarding some basic ecological and biological characteristics of the species, there is relevant information that has been generated in the last decades about foraging behavior, migratory movements, diet, habitat use, and genetics (Hayward and Cockrum 1971; Easterla 1972; Moreno-Valdéz et al. 2000; Téllez 2001; Sánchez and Medellín 2007; Ammerman et al. 2009; Toledo 2009; Galicia 2013). For instance, a general migration pattern has been proposed by several authors (Baker and Cockrum 1966; Barbour and Davis 1969; Hayward and Cockrum 1971; Easterla 1972; Humphrey and Bonnacorso 1979; Wilson 1985; Arita 1991; Schmidly 1991; Hoyt et al. 1994; Rojas-Martínez et al. 1999; Moreno-Valdéz et al. 2000; Rojas-Martínez 2001; Téllez 2001; Moreno-Valdez et al. 2004; Ammerman et al. 2009; Medellín et al. 2009). While females spend summers in the northern part of the range, in the Chihuahuan Desert, in Mexico, where they give birth and establish maternity roosts (Easterla 1972; Arita and Humphrey 1988; Moreno-Valdez et al. 2004; Ammerman et al. 2009), males supposedly move along an altitudinal gradient throughout the year in the center of Mexico (Rojas-Martínez et al. 1999). Eventually, females and young seem to move towards southwestern United States, to the Big Bend National Park, in Texas (Easterla 1972; Schmidly 1991; Ammerman et al. 2009; Adams and Ammerman 2015) and to the Romney Cave, in the Big Hatchet Mountains, in New Mexico (Hoyt et al. 1994). By the late summer and early autumn, females and sub-adults migrate to the southern part of their distribution, in Central Mexico, where they meet with the males (Arita and Humphrey 1988; Hoyt et al. 1994). It is in this stage of the cycle when mating occurs (Téllez 2001; Toledo 2009). By the end of winter and early spring, only the pregnant females return to the north of México (Moreno-Valdez et al. 2004).
Mating behavior of L. nivalis has been reported only in one roost called “La Cueva del Diablo” (Téllez 2001; Toledo 2009; hereafter CDMR for Cueva del Diablo Mating Roost). The CDMR is located in a highly threatened region in the state of Morelos, in Central México. This cave maintains a big population of the species throughout the winter season every year, providing a promising start for the reproduction cycle of the colony.
The region in which CDMR is located is under severe anthropogenic pressure from accelerated urban development. Additionally, the cave is accessible and regular human activity represents a significant detriment on the bat population. Although CDMR consists of one of the most important roosts for the species and have historically maintained a relatively big population, in the last decade, population size has been consistently low with some oscillations. For instance, in 1996 population size was estimated around 5,000 individuals; in 2008 between 8,000 and 10,000 individuals (Medellín 2003; López-Segurajáuregui et al. 2006); in December 2012, approximately 4,000 individuals were present (personal observation, December 2012), and from 2011 to 2016 this colony size has remained this size and stable (USFWS 2018).
Leptonycteris nivalis is considered ‘Endangered’ by both the IUCN (Medellín 2016) and the United States Endangered Species Act (USFWS 1994) and as ‘Threatened’ by the México (SEMARNAT 2010). Among the underlying factors for the risk categorization are: the lack of information regarding the species´ population status in its entire distributional range; the general small size of colonies (Arita and Humphrey 1988); the low number of known roosts (USFWS 1994; Téllez 2001); the high dependence on food availability across seasons and range (Easterla and Whitaker 1972; Humphrey and Bonnacorso 1979; Moreno-Valdéz et al. 2000); and the high risk observed in the CDMR (Medellín 2003; López-Segurajáuregui et al. 2006; Galicia 2013).
In order to identify the main threats for the conservation of L. nivalis, to detect the most urgent information to obtain, and the main recovery actions to take, in 1994, a Recovery Plan for the species was developed as part of the Endangered Species Act process (USFWS 1994). Later, in 2015, specialists met to review and update the species status assessment (USFWS 2018). In both evaluations, the location and protection of new roosts was recognized as an urgent need.
In this study, we aimed to identify additional potential mating roosts of L. nivalis by using Ecological Niche Modeling (ENM) and Euclidian Multidimensional Distances Analysis (EMDA) to assess habitat suitability, by finding ecologically similar areas to the site where the CDMR is located. For doing this, we incorporated environmental variables that could be influencing their presence and, we conducted field surveys to the predicted areas to confirm the presence of the species. The use of these techniques represents the first effort to locate specific bats ‘roosts, and particularly for L. nivalis.
Materials and methods
Study site. With the aim at focus our study to the region where the species has been recorded during winter, our study was held including the entire central region of México and considering the distribution of the main ecosystems used by the species. In terms of biogeographical criteria, this region encompasses the Trans-Mexican Volcanic Belt province and part of the Balsas Basin province. We used the entire area, composed by both physiographic provinces, for the calculation of the environmental Euclidian distances between the CDMR location and the rest of the study area and for the development of Ecological Niche Models. The Trans-Mexican Volcanic Belt is a physiographic province spanning more than 880 km east to west and is characterized by the presence of mountains with peaks above 5,000 meters (Morrone 2001), from which one is the type locality of the species (Saussure 1860). The climate is largely temperate with most of the rainfall occurring in summer C(w) (Köppen classification and modified by García 1998). The vegetation type includes tropical dry forest, pine-oak forest, shrublands, and in a lesser extent, cloud forest and alpine vegetation (Rzedowski 1978; Instituto Nacional de Estadística y Geografía; INEGI 2016). The Balsas Basin province is bound on the north by the Trans-Mexican Volcanic Belt and to the south by the Sierra Madre del Sur province. It is composed mainly of pine-oak forests and tropical dry forests. The predominant climate is tropical with dry winters (Aw; Rzedowski 1978).
The CDMR is located in the Santo Domingo locality, approximately 4 km north of the town of Tepoztlán, in the state of Morelos, at 1960 masl. (Hoffman et al. 1986; López-Segurajáuregui et al. 2006). It is in the buffer zone of the “Corredor Biológico del Chichinautzin”, a federally protected area under the category of Protection of Flora and Fauna, decreed by the National Commission of Protected Areas (Comisión Nacional de Áreas Naturales Protegidas; CONANP 2016) and is part of the Trans-Mexican Volcanic Belt province (Figure 1).

Figure 1 Study site. Top: Location of the Balsas Basin and the Transvolcanic Belt provinces. Bottom: Location of the cave “La Cueva del Diablo”.
Euclidian multidimensional distances analysis (EMDA). Euclidian Multidimensional Distance Analysis are developed by using Geographic Information Systems (GIS) to identify cells or pixels with the least ecological Euclidian distance within a map of an established area according to some ecological variables. For this analysis, we defined ecological Euclidian distance through ecological and environmental variables that we assume are characterizing the site where the CDMR is, and we aim at finding the most ecologically similar areas. For doing that we followed Rice et al. (2003) and Ferreira de Siqueira et al. (2009) methods, which calculated Euclidian distances in an environmental space between two geographical points. By using ArcGis (ESRI 2011), we set CDMR as a reference point in an environmental space to calculate the multidimensional Euclidean distance between all pixels of the area and the CDMR. For this analysis, we used 19 environmental variables from Worldclim (Hijmans 2005) and three topographical variables: elevation, topographic index, and aspect (U. S. Geological Survey 2001). All variables were z-standardized and combined in ArcGis. Finally, we classified the resulting ecological distances in five unitless categories (0 to 2,500, 2,500 to 5,000, 5,000 to 10,000, and 10,000 to 20,000). Lower values represent greater ecological similarity to CDMR.
Ecological niche modeling (ENM). Ecological Niche Modeling identifies non-random associations between environmental conditions and known species presence (Nix 1986; Guisan and Zimmerman 2000; Soberón and Peterson 2004; Peterson et al. 2011) to identify potential distribution of the species across a delimited area. To generate the models, biological data from species records and data on environmental variables that characterize the ecological niche are required as inputs.
Biological data. We modeled the potential distribution of the Mexican Long-nosed bat during the autumn and winter seasons in the center of México. We gathered information on the presence records of this species from published literature and scientific collections to obtain different and independent locations for the ENM (Supplementary material 1). When possible, we visited the museum collections to assess the taxonomic identification of every specimen. We examined both skulls and specimens.
We used only museum records of the species corresponding in space and time with the mating season of the species (i. e. locations in central México and from late autumn to early spring). To avoid bias in our analyses we only use one record per locality. We generated three stacks of biological data of different length: 1) from August to March, hereafter “8 - M” for the eight months comprised, 2) from September to February, hereafter “6 - M” for the six months comprised, and 3) from November to February, hereafter “4 - M” for the period of four months.
Environmental data. For the ENM we used three monthly environmental surfaces: highest temperature, lowest temperature, and monthly total precipitation (Cuervo-Robayo et al. 2013), and the three aforementioned topographical variables. We used the Cuervo-Robayo and collaborators surfaces because they consist in the most up to date high-resolution climate surfaces for México, and they cover a wider and recent period. We consider these variables as appropriate for this analysis not only because of the higher quality of the data, compared to the other three available climate surfaces for the country (Sáenz-Romero et al. 2009; Téllez-Valdés et al. 2011; Hijmans et al. 2005), but also because they represent the base for other derived climate variables that consider annual patterns, and for this study we aimed at model only the winter ecological niche of L. nivalis. We generated three environmental data stacks corresponding temporally with the biological data stacks (i. e. “8-M”, “6 - M” and “4 - M”).
Ecological niche models- We generated ecological niche models for the three-time stacks with Maxent 3.3.3k (Phillips et al. 2006; Phillips and Dudik 2008), GARP (Stockwell and Noble 1992), and Bioclim (Nix 1986). For GARP and Bioclim we used the OpenModeller platform using the GARP with best subsets mode (Anderson et al. 2003; Muñoz et al. 2009). We built models corresponding to each period using the three algorithms. As a result, we obtained a total of nine models. We created a binary map for each model by defining a threshold value based on the prediction characteristics of the algorithms (Table 1). For Bioclim a threshold was established considering omission error of 0, for Maxent less than 10 %, and for GARP less than 20 %. For each period, the three binary maps corresponding to each algorithm were overlapped, and those areas that were predicted by the three algorithms were -again- converted to a binary map, generating a period consensus map. For doing this, we established an identifier value for each period model (4, 60, 800 for the 4 - M, 6 - M, 8 - M, respectively). Finally, we constructed a final agreement map by overlapping the predicted area of the three resulting period consensus maps using the merge function of the ArcGis (Figure 2).
Table 1 Criteria used for each model to establish threshold values.
Period | Algorithm | Threshold criteria |
---|---|---|
August - March | Maxent | Logistic threshold value: 0 - 0.199 (absence) and > 0.199 (presence) |
GARP | Consensus of eight models | |
Bioclim | Area containing 100 % of the records | |
September -February | Maxent | Logistic threshold value= 0 - 0.249 (absence) > 0.249 (presence) |
GARP | Consensus of seven models | |
Bioclim | Area containing 100 % of the records | |
November - February | Maxent | Logistic threshold value= 0 - 0.404 (absence) > 0.404 (presence) |
GARP | Consensus of nine models | |
Bioclim | Area containing 100 % of the records |
Most of the museum records that were used for our analyses came from very few unique locations (34); therefore, all models used all data as training data. Additionally, to evaluate the predictive capacity of each model, we generated models using 75 % of records as training data and 25 % as testing data. Furthermore, using the omission error values of the testing data we assessed all model predictions using the area under the ROC curve and a Chi-Square Goodness of Fit test (Supplementary material 2).
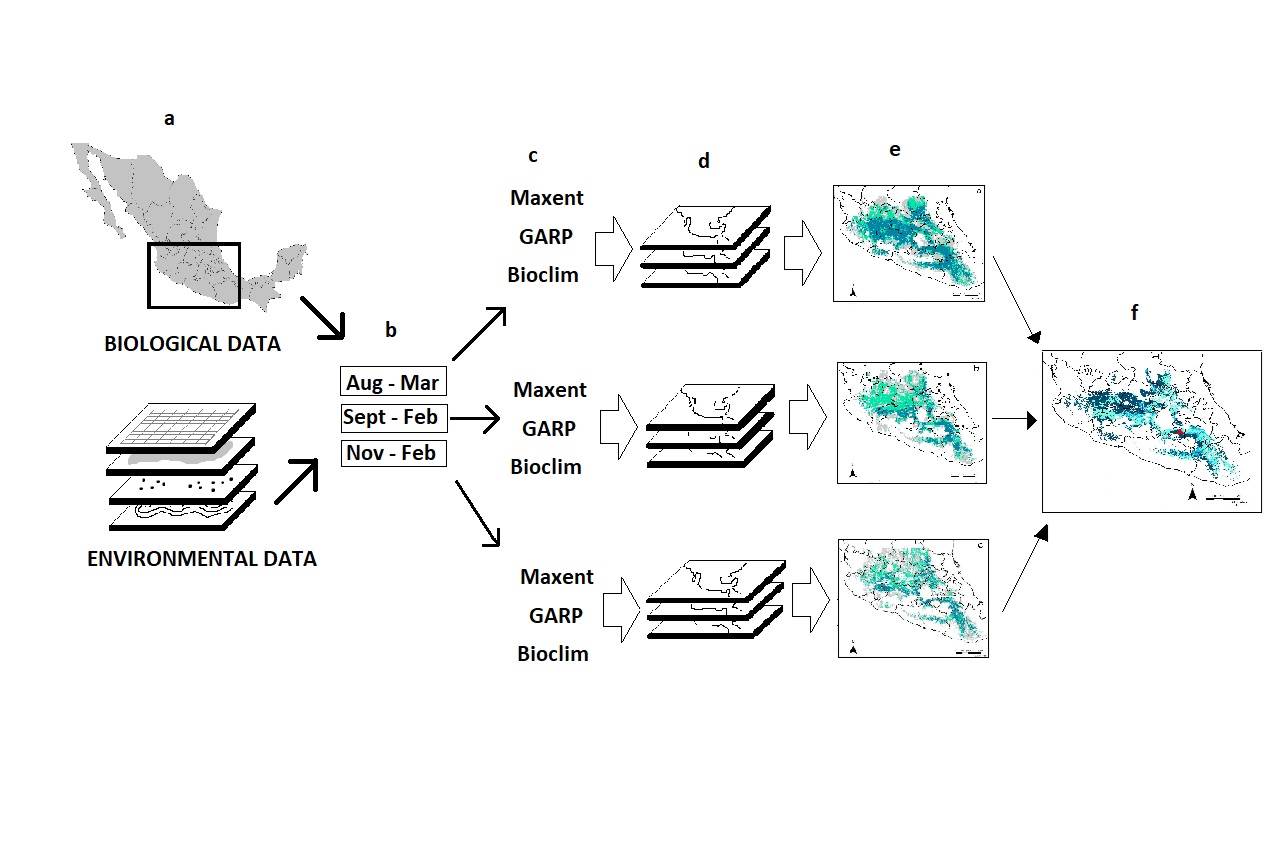
Figure 2 ENM method used in the study. Biological and environmental data (a) were divided into three periods of time (b). Maps were generated with three different algorithms (c), for each period (d). A consensus map of the three algorithms for each period was generated (e). We obtained a final agreement map of all time periods for all algorithms (f).
Euclidian Multidimensional Distances Analysis and Ecological Niche Modeling. The ENM final map was overlapped with the map obtained from the EMDA. Finally, we used a land cover and vegetation map (Series IV) generated by the National Institute of Geography and Statistics (INEGI 2016) to selected areas with low natural vegetation impact.
Fieldwork. During the winters of 2012 and 2013 we conducted visits to several caves (both in private and protected lands) within the high-suitability areas identified in our analyses. During the day, we entered the caves to look for evidence of the presence of L. nivalis (the species’ characteristic smell and traces of guano of a nectarivorous bat). During nights, we set mist nets at cave entrances to capture and identify the species present, following the Medellín et al. (2008) identification key. For all individuals that we captured, we registered the species and took standardized measurements of body mass and forearm length. For all L. nivalis individuals we estimated age, determined the sex, and performed a first assessment of the reproductive state of the individuals. For females, we observed characteristics associated to gestation or lactation, such as the presence of enlarged nipples and milk-filled mammary glands, and the detection of palpable embryos (in small mammals’ embryos tend to be palpable after one week; Kunz et al. 1996). This allowed us to distinguish between apparently inactive, lactating, and pregnant females. For males, we assessed whether the testes were scrotal or abdominal (Kunz and Parsons 1988).
Additionally, to identify females in their earliest stages of gestation, as well as sexually receptive females, we performed cytological tests following Goldman et al. (2007). We collected vaginal smears to identify the structure of the vaginal epithelial cells. This involved the introduction of 2 µl of physiological solution into the vaginal orifice using a micropipette (vol.= 0.5-10 µl) and collecting the wash fluid that carried epithelial cells. We only conducted one trial per female, even if that trial failed. Afterwards, we observed the epithelial cells using an optic microscope (40x and 100x). Furthermore, as an exclusive event, to corroborate the reproductive status of a pregnant female in its earliest stage, in addition to the cytological assessment, we collected one pregnant female of L. nivalis. We euthanized this individual by administering an intraperitoneal injection of a lethal dose of Sodium Pentobarbital with a very small-gauge needle two hours after it was collected. We followed protocol described in the Euthanasia Reference Manual (The Human Society of United States 2013). Captures and animal management were conducted following the American Society of Mammologists (ASM) guidelines (Sikes et al. 2016) and using the License of scientific collector (Research permit SGPA/DGVS/06361/16) provided by the Secretary of the Environment and Natural Resources (Secretaría de Medio Ambiente y Recursos Naturales in Spanish; SEMARNAT). All procedures were made ensuring all bats’ welfare and the least suffering.
Results
Euclidian Multidimensional Distances Analysis and Ecological Niche Modeling. From 347 individuals that we examined, belonging to five scientific collections: Escuela Nacional de Ciencias Biológicas (ENCB), Colección Nacional de Mamíferos (CNMA), Universidad Autónoma Metropolitana (UAMI), Colección de Mamíferos del Museo de Zoología de la Facultad de Ciencias (MZFC-M), and the Colección Osteozoológica del Laboratorio de Arqueozoología M. en C Ticul Álvarez Solórzano del Instituto Nacional de Antropología e Historia (INAH), 73 % were correctly identified as L. nivalis; the rest corresponded to L. yerbabuenae. Furthermore, considering four more scientific collections: Colegio de la Frontera Sur (ECOSUR Chiapas), Centro Interdisciplinario de Investigación para el Desarrollo Integral Regional, Unidad Oaxaca and Unidad Durango (CIIDIR), and the Universidad Autónoma de Aguascalientes (UAA), we obtained 51 different localities in central México for the 8 - M period, 26 for the 6 - M period and 16 for the 4 - M period.
The EMDA showed that the region with the most similar environmental characteristics to CDMR is transversally distributed throughout the north of Morelos, across the “Tepozteco” formation, in the south of the State of México, in the northwest region of Puebla, and, scarcely, in the north of Guerrero (Figure 3).
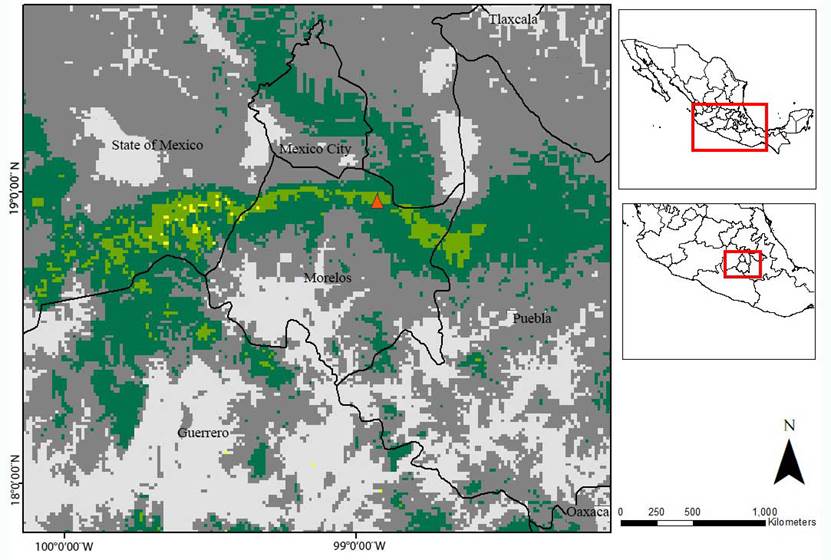
Figure 3 EMDA to “La Cueva del Diablo”, Tepoztlán, Morelos, Mexico. Yellow: region with the lowest ecological distance, hence, the most ecologically similar region to CDMR (distance of 2,500). Light green: the next most environmentally similar region (2,500 - 5,000). Dark green: third distance class (5,000 - 10,000). Dark gray: regions with an ecological distance between (10,000 - 15,000). Light gray: the most ecologically different region to CDMR (> 15,000).
Regarding the ENM, due to a higher number of localities used for the 8 - M period, the predicted area by this approach was considerably larger than the other models (6 - M and 4 - M). This area consisted in a broad band located mainly in the Trans-Mexican Volcanic Belt encompassing of Jalisco, Michoacán, Guanajuato, Querétaro, San Luis Potosí; as well as a more defined area located between México City and a small part of the State of México, in the Balsas Basin province. Finally, this model also predicted a considerable region located between the Balsas Basin and the Southern Sierra Madre provinces, throughout most of Morelos, south of Puebla, and northeastern and northwestern of Guerrero and Oaxaca, respectively (Supplementary material 2).
Furthermore, the ecological niche modeling agreement maps from the three periods that were analyzed identified a few common areas of potential distribution, which -although not entirely- corresponded to the regions predicted by the more restricted model (corresponding to the 4-M period). These areas encompass a relatively narrow strip that cross from the southeast of the state of Jalisco, through the north of Michoacan, south of the State of México, north of Morelos, western and south of Puebla, down to the north of Oaxaca, and a small area in the northeast of Guerrero. Additionally, a reduced area located in the north of Guanajuato and Querétaro, and the south of San Luis Potosí was predicted by the three models too (Figure 4).
In addition to differences and similarities found in the areas predicted by the three time periods, variations in performance values, according to the algorithm were also observed. For instance, Bioclim showed 0 omission error, and the highest X 2 values, but it also predicted the wider potential areas. In that sense, a trade-off between omission error and percentage of area predicted by the algorithms was observed. In general terms, GARP predicted a more constrained area (allowing a more precise field search), but it also showed de highest omission error. This was expected because we established a higher threshold for this algorithm because GARP tends to overpredict. Specifically, for the 4 - M model, Maxent showed the best performance, showing the lowest omission error (12.5 %), and the more constrained area (17.6 %). All the results of the chi-square tests that were performed were significant (P < 0.005; Supplementary material 3).

Figure 4 Agreement map of potential distribution of L. nivalis generated by three periods of training data (8 - M, 6 - M and 4 - M). Dark blue: Region predicted by the model 8 - M. Light blue: Region predicted by the model 6 - M. Aqua green: Region predicted by the model 4 -M. Red triangle: Location of “La Cueva del Diablo”.
Finally, the map resulting from the overlapping of the EMDA analysis and the final agreement map of the ENM show that a narrow, but well defined, strip crossing transversely the south oh the State of México, north of Morelos and southwestern Puebla, holds the most suitable habitat for the location of additional mating roosts for L. nivalis (Figure 5A). Nevertheless, this particular region is under severe anthropogenic pressure, therefore, a great proportion of the predicted region is highly disturbed. In that sense, according to the land cover information, the most conserved area of potential suitable habitat consists of a narrower corridor located in the boundary between the south of the state of México, and the northeast of Morelos (Figure 5B).
Field results. In fourteen nights of sampling effort, we visited a total of seventeen caves in eight unique sites in central México (Supplementary material 4). We found L. nivalis individuals in only one cave: “La Cueva de los Coyotes,” located in the Municipality of Tonatico, south of the State of México, near the boundary with Guerrero.
In four visits conducted to “La Cueva de los Coyotes”, we captured a total of 18 individuals of the Mexican Long-nosed bat. Due to our “one trial” procedure for cytological tests we obtained epithelial cells from only one female in January 2014. We found nucleated cells as the predominant cell type and a small number of leukocytes in the smear (Supplementary material 5). This result indicates that the female was probably in a transition process between diestrous and a proestrous stages. In addition, on January 28th of 2013, we collected a female for the corroboration of the reproductive status, and she was carrying a 15 mm embryo.
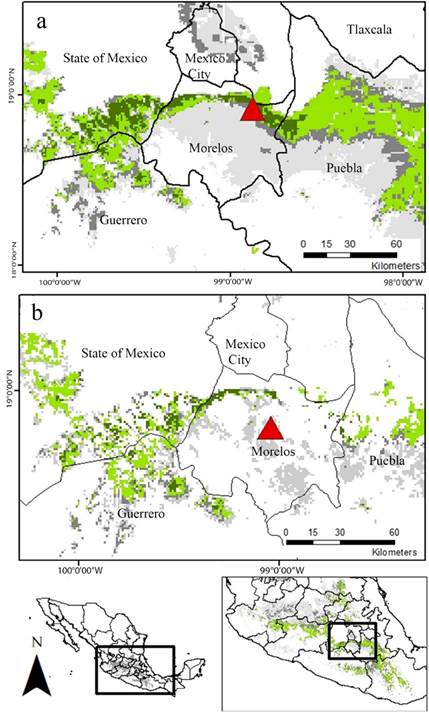
Figure 5 Overlap of EMDA and ENM maps. a. Potential distribution area. b. Conserved patches of the potential distribution area. Dark green: ecologically more similar region to “La Cueva del Diablo” and area predicted in the three periods for ENM analyses. Light green represents regions that were predicted by three of the four analyses we carried out. Dark gray: represents regions that were predicted only by two of the resulting maps. Light gray: areas predicted by only one analysis. Red triangle: Location of “La Cueva del Diablo”. Red triangle: Location of “La Cueva del Diablo”.
Discussion
Our results show that the most suitable area for the location of additional mating roosts of L. nivalis is located in a narrow strip running from southwestern State of México to northern Morelos and southwestern Puebla, in Central México. Within this area, we identified a cave called “La Cueva de los Coyotes”, in which for two consecutive years we found 18 individuals of L. nivalis and at least one pregnant female. Usually, colonies of L. nivalis are composed by thousands of individuals (López et al. 2006; Sánchez and Medellín 2007; Toledo 2009), therefore, finding this small number of individuals makes and interpretation of the type and use of this roost difficult. However, the presence of the species for two years may indicate that the area, and specifically, the roost, is being used somehow. It is possible that roosts located among ecological similar areas to CDMR are being used sparsely for parturition and births, and not specifically for the mating. It is also plausible to think that additional mating roosts are distributed along ecologically similar areas, but they are not used by colonies as large as that of the CDMR. Previous studies have suggested that northern colonies of L. nivalis are more numerous than the southern ones (Easterla 1972; Easterla and Whitaker 1972). Additionally, the spreading into smaller colonies following a decreasing latitudinal gradient pattern has already been documented in the Brazilian Free-Tailed bat (Tadarida brasiliensis) maternity colonies (López-González and Best 2006).
Furthermore, the finding of individuals of L. nivalis may indicate that their presence is not a fortuitous event, and questions may arise regarding the reproductive dynamics of the species. For instance, is the presence of a pregnant and a sexually receptive female enough evidence to consider “La Cueva de los Coyotes” a mating roost? or, is “La Cueva de los Coyotes” a transitional roost to reach other, -more suitable- caves? Is the occupation of this type of roost a consistent and normal process, or does it constitute a consequence of the perturbation of “La Cueva del Diablo”, and therefore, a reflection of the observed decline in size population in this cave? Despite all possible scenarios, all previous information collected for L. nivalis indicates that CDMR represents the most important roost in the southern part of the species distribution range due to the large population it holds yearly during winter, and the consistent mating behavior that has only been documented there (López-Segurajáuregi et al. 2006).
Our ENM showed that, besides the region described above, an additional area was identified as a potentially suitable area for the location of roosts of the species. It is possible that this outcome is the result of a more complex association of L. nivalis with the environmental variables used for the models than the one that was assumed for this study. For instance, records of L. nivalis that were used for the construction of the ENM could be reflecting a relative ecological plasticity in the species since the models identified potential regions that are not ecologically similar to the CDMR (at least not through the environmental variables used in this study). This region includes the Mixteca region, located in northern Oaxaca and southern Puebla. The capacity of the species to use a variety of habitats, such as tropical dry forest, pine-oak forest, and shrublands (Arita 1991), could be the result of a relative broad tolerance spectrum of L. nivalis to different environmental factors. Additionally, a foraging compensatory capacity has been documented for this species (Ayala-Berdon et al. 2013; Galicia 2013). By incorporating this information into further analyses, it could be possible to obtain more accurate estimates of potential areas of distribution of L. nivalis as well as the identification of potential mating and maternity roosts.
Limitations of the methods followed in this study include that ecological niche models are very sensitive to the quality and quantity of records. Therefore, the lack of information of the species, along with its complex biology and its ability to take long flights could limit model performance (it has already been documented that the smaller sister species L. yerbabuenae can make flights of more than 100 km per night; Medellín et al. 2018). Furthermore, since L. nivalis is a species that shows a strong cave roosting affinity, it could be also informative to know microclimate requirements of their roosts, in addition to habitat requirements through a landscape perspective. Therefore, future studies could consider not only macroscale habitat characteristics, but also some of the geological and topographical features that may influence roost selection.
Moreover, our study identified regions that met environmental conditions associated with mating roosts during winter season. These predictions could be complemented with a landscape characterization of the area of distribution of L. nivalis through the identification of potential foraging patches and assessing their use by the species. In addition, foraging areas can be associated with known roosts, and consequently, information regarding daily movements, energy and environmental requirements can be estimated, providing powerful tools for the conservation of the species.
Although additional refinements of our analyses are required, and the identification of relevant climatic variables defining ecological niche of L. nivalis were not within our goals, we consider that precipitation and temperature acted as appropriate variables to evaluate the habitat suitability for the species because we located a delimited geographical area that corresponded to the distribution of the main ecosystems used by the species (i. e. tropical dry forest, pine-oak forest, and the transition zones between them; Arita 1991). Moreover, nectar-feeding bats depend on the availability of blooming flowers and nectar production; thus, they depend on the phenological patterns of the plant species that conform their diet. In that sense, precipitation and temperature patterns are intimately related to food availability, as they consist in the main environmental triggers for regulating phenological processes (Lyndon 1992; Marqués et al. 2004; Diaz and Granadillo 2005; Stevenson et al. 2008). Hence, these variables should be influencing the foraging ecology of L. nivalis too. Despite there is much to be learned regarding flight ecology, flight daily distances, and foraging ecology for this species, feeding resource distribution and availability appears to influence their distribution and migratory movements (Fleming et al. 1993). Furthermore, physiological characteristics of the species could also be influencing the distributional patterns observed for the species by giving the species the faculty of inhabiting colder environments relative to other close related species (Ayala-Berdon et al. 2013). For instance, Espinosa (2008), found that minimum temperature is the variable that remains constant across all localities of L. nivalis throughout the entire year. In contrast, precipitation varies considerably between winter and summer localities.
The conservation of the endangered migratory species L. nivalis depends on the protection of multiple key elements throughout its entire range of distribution (USFWS 1994). Within these elements, the temporal mating roosts used by the colonies each year are essential to the long-term conservation of the species. The “Cueva del Diablo” mating roost (CDMR), located in central México, is the only mating roost known for this species and is under threat from disruptive activities in and around the cave; therefore, it is of capital importance to locate additional mating roosts, and to increase our understanding of the population dynamics of this species.
This study represents the first effort to find such a specific target as mating roosts for a bat species. The main potential regions predicted by our analyses covered the “Chichinautzin” corridor (located in the north of Morelos), southwestern Puebla and southeastern State of México. We recommend further exploration of this area to identify more mating roosts of Leptonycteris nivalis. Additionally, future monitoring of “La Cueva de los Coyotes” is important to elucidate its role in the reproductive dynamic and the conservation of this species.