Introduction
Digestibility is a way to determine the utility of foods; that is, the ease with which these are converted in the digestive system into useful substances for nutrition (Yoon et al., 2019). It comprises two processes, the digestion that corresponds to the hydrolysis of complex food molecules and the absorption of small molecules (amino acids, fatty acids) in the intestine (Ye et al., 2018). Microbiota is essential in these processes since the malfunction of these microorganisms is associated with metabolic diseases (Avolio et al., 2020).
A current tendency of the world population is focused on consuming natural foods derived from plants with high nutritional value (Román-Cortés et al., 2018; Orozco-Sifuentes et al., 2019; Ramírez-Jaspeado et al., 2020) and prebiotic properties (Talebi et al., 2020). Stenocereus queretaroensis (Cactaceae) is a plant endemic to Mexico (Campos-Rojas et al., 2011). There are different types, but the red one has a higher production and impact on the Mexican economy (Quiroz-González et al., 2018). The Mexican population consumes the pulp (Gaytán-Andrade et al., 2019; Quiroz-González et al., 2018) and the pigmented juice is used to prepare traditional ice drinks (Soto-Castro et al., 2019). Mexico produced 5807 t in the year 2019 (SIAP, 2020).
Stenocereus queretaroensis presents total soluble solids content of 16 ºBrix and total sugars of 10 to 11 %. The titratable acidity percentage (citric acid) ranges from 0.15 to 0.50 % and the pH from 3.9 to 5.2, depending on the pitayo type (Gaytán-Andrade et al., 2019).
The S. griseus fruit has a pH higher than 4.5 and contains 9.9 % of total sugars (Chuck-Hernández et al., 2016). To date, no extraction methods have been described for the fruit, but ultrasound extractions have been used to extract active ingredients from Cactaceae (Ramli et al., 2014); however, these methods can modify the integrity of the chemical structure of the components (Sasidharan et al., 2011). On the other hand, the methods for evaluating in vitro digestibility of food depend on the type of sample (Bornhorst and Singh, 2014; Brodkorb et al., 2019). Digestibility data of plant extracts are scarce, including those for S. queretaroensis; however, simulatedin vitrooral (using saliva), gastric, and intestinal (small intestine and large intestine) digestibility tests are commonly used for this purpose (Chen et al., 2018).
To date the nutritional and functional information of S. queretaroensis extracts is not completely known (Soto-Castro et al., 2019), even so, it could represent a source for development of new functional foods (Gaytán- Andrade et al., 2019). For this reason, the objective of this study was to characterize and to evaluate digestibility and prebiotic property of the extract of red S. queretaroensis fruit.
Materials and methods
Plant material and extraction
Red fruits of S. queretaroensis (FAC Weber) Buxbaum were harvested in Amacueca, Jalisco, México (coordinates: 19° 56’ N, 103° 04’ W, altitude of 1361 masl) between May and June 2017 at physiological maturity suitable for consumption. A sample of the plant was identified and deposited at the herbarium of the Antonio Narro Autonomous Agrarian University with the registration number 99186. The extract of the fruit (2.5 kg) without pericarp was carried out in an extractor (Turmix, Mexico City) inside a cold room (4 ºC) to guarantee the integrity of the compounds. The extract was centrifuged at 2200 × g for 30 min, filtered with Whatman # 4 paper, and clarified with Whatman G/FA; then, it was dried with a lyophilizer (Labconco, Freeze dryer 5, Kansas, EUA). The yield percentage Y (%) was calculated by gravimetric analysis from the fruit weight (g) used for the extraction as described in the formula:
The extract was stored at -20 °C until use (Hernández et al., 2017).
Physicochemical and proximal analyses
The total soluble solids (TSS, ºBrix) were measured using a refractometer (Atago®, PAL-1, Tokyo), pH was determinded with a potentiometer (Hanna, pH 209, Plopis, Romania) and color (L*, a* and b*, using the CIELAB scale) was measured by using a colorimeter (PCE instrument, CSM-2, Albacete, Spain). These parameters were evaluated in a 1 mg mL-1 solution prepared from the lyophilized extract. ºBrix were measured with 100 µL, while pH and color with 50 mL. Additionally, titratable acidity (TA) was measured as the % of citric acid using method 942.15 (AOAC, 2000; Sadler and Murphy, 2010). The test was carried out by titrating 5 mL of a dilution of the lyophilized extract to 0.2 mg mL-1 with 0.1 N NaOH, using 1 % phenolphthalein in ethanol as indicator. The proximal analysis was performed using the AOAC official methods (AOAC, 2000). The moisture and ash content were measured by methods 925.09 and 923.03 using 1 g of lyophilized extract. Ash content was determined by incineration in a muffle at 550 ºC. The fat, crude fiber and protein contents were determined by methods 920.39 (Soxhlet), 962.09 and 979.09 (Kjeldahl, N × 6.25) in 10 g of lyophilized extract. Protein content was measured from the previously defatted lyophilized extract. The percentage of the nitrogen-free extract calculated as % Nitrogen-free extract = 100 - (% humidity + % ash + % fat + % crude protein + % crude fiber). The parameter corresponds to the total content of digestible carbohydrates, vitamins and soluble non-nitrogenous organic compounds (Dick et al., 2019). All measurements were on dry weight (DW) basis, except the moisture.
Digestibility
Oral digestibility was measured using the method described by Chen et al. (2018) with some modifications. Three healthy 30 to 40-year-old volunteers, without receiving any antibiotic treatment for at least three months, supplied saliva and it was used immediately without any treatment. The test was done in two tubes, in the first one 1 g of extract was placed and in the other 1 g of distilled water was used as a control; then, 2 mL of saliva were added to each tube, mixed for 10 s and incubated at 37 °C for 2 min. Right away, the amylase was inactivated in a boiling water bath for 5 min. The tubes were centrifuged at 5000 × g for 10 min to remove the supernatant and the precipitate was dried at 50 ºC for 3 h. Finally, the precipitate was weighed and the percentage of oral digestion calculated using the formula:
Gastric digestibility percentage was measure using a combination of the methods described by Chen et al., 2018) and Mkandawire et al. (2015) with some modifications. Solution A was prepared by mixing an electrolyte solution (3.1 g of NaCl, 1.1 g of KCl, 0.25 g of CaCl2 and 0.6 g of NaHCO3 in 1 L of distilled water) with a solution of 0.1 mol HCl L-1 at 1:1 ratio. Solution B was prepared with 1.5 mg mL-1 pepsin dissolved into 0.1 mol L-1 sodium phosphate buffer, pH 3. All solutions were dissolved at 37 °C. Gastric digestibility was tested in a tube containing 1 g of the lyophilized extract along with 1 g of distilled water as a control. First, gastric fluid was prepared with 7 mL of solution A and 3 mL of solution B, adjusted to pH 3 with 0.1 mol L-1 HCl; then, 10 mL of gastric fluid were added to each tube to start digestion and stirred for 2 h at 37 °C. Pepsin was inactivated in a boiling water bath for 5 min, the tubes were centrifuged at 5000 × g for 10 min, and the supernatant removed.
The precipitate was washed with 20 mL of 0.1 mol L-1 sodium phosphate buffer (pH 7) and recovered using centrifugation. The precipitate was dried for 3 h at 50 °C and weighed. The percentage of gastric digestion was calculated using the formula
Digestion in the large intestine was investigated by in vitro fermentation with the DaisyII Method as described by Coblentz et al. (2019) with some modifications. Before starting the test, solutions A, B, and fecal inoculum were prepared. Solution A was prepared with 10 g of KH2PO4, 0.5 g of MgSO4 • 7H2O, 0.5 g of NaCl, 0.5 g of urea, and 0.1 g of CaCl2 • 2H2O in 1 L of distilled water. Solution B was prepared with 15 g of Na2CO3 and 1 g of Na2S • 9H2O in 1 L of distilled water. The human fecal inoculum solution was prepared from a mixture of equal amounts of stools donated by two healthy volunteers without antibiotic treatment. The solution was prepared with 200 g of feces dissolved in 2 L of sterile 0.9 % saline solvent at 37 ºC. The solution was homogenized using a glass rod and filtered through a 0.15 mm pore mesh. The tests were carried out in 4 × 4 cm polyester-polyethylene bags (filter bag F57) with a pore size of 25 µm. The bags were rinsed using acetone and air-dried. The empty bag was weighed, and then 1 g of extract was placed inside. In addition, an empty bag was weighed and used as a control and all bags were heat-sealed. All the glassware and the digestion jar were kept at 37 ºC.
Simultaneously, a digestion solution was prepared with 400 mL of human fecal inoculum solution, 1.2 L of solution A and 250 mL of solution B, and maintained at 37 °C. The samples were evenly distributed in the jar in which the digestion solution was added; then, the jar was covered, purged, supplied with CO2 for 30 s and incubated for 24 h at 37 °C in the DaisyII incubator. At the end of the incubation, the jar was removed and the liquid drained. The digested bags were recovered, rinsed with distilled water until the water was clean; then, the microbial residues and any remaining soluble fractions were removed with a neutral detergent solution. Subsequently, they were dried for 3 h at 50 ºC and weighed. Calculation was performed as follows:
Prebiotic evaluation
Commercial strains of B. coagulans, L. sporogenes, B. clausii, L. fermentum and L. delbrueckii were used in the assay. The strains were activated using MRS 55 g L-1 broth at 37 °C for 12 h. The prebiotic effect was evaluated through bacterial growth, by combining the McFarland technique and the growth kinetics of each strain during the exponential phase (De et al., 2014; Jurado-Gámez et al., 2014). The growth of each strain at 24 h was compared to evaluate the prebiotic effect. The absorbance of the McFarland 0.5 scale was used to adjust the bacterial suspension of the initial inoculum (1 × 108 CFU mL-1) used in each growth kinetics. The growth kinetics of each strain was performed in tubes with 9 mL of MRS, 1 mL of S. queretaroensis extract at 1000 µg mL-1 and 300 µL of bacterial suspension as initial inoculum. A blank without inoculum was used to adjust the absorbance. Tubes were incubated at 37 °C for 24 h in anaerobiosis, and absorbance was measured every 2 h. All absorbances were measured in a quartz cell at 625 nm in a UV-visible spectrophotometer (Thermo spectronic, Genesys 5, New York, EUA). The bacterial growth was estimated in CFU mL-1 employing an absorbance adjustment with the McFarland 0.5 scale, as follows: CFU mL-1 = (absorbance of medium with extract inoculated) (1.5 × 108) / absorbance of the 0.5 McFarland scale.
Statistical analysis
All the experiments were conducted in triplicate (n = 3) and results were expressed as a mean ± standard deviation. The digestibility and prebiotic effect data were analyzed by ANOVA and Tukey mean comparison test (P ≤ 0.05). Graph Pad Prism-7® software was used for the statistical analysis.
Results and discussion
Plant material and extraction
There was no previous information on the yield percentages of the S. queretaroensis fruit, but in this study, a Y (%) of 91.23 ± 0.1 % was estimated. The yield of S. queretaroensis was similar to ultrasonic extraction from the pulp of Hylocereus polyrhizus (Cactaceae) of 90.08 % (Ramli et al., 2014); therefore, the extraction yield percentage depends on the conditions used for each extraction; however, the main advantage of the present procedure is that compounds of S. queretaroensis maintain their integrity, as the conditions used do not modify their chemical structure (Sasidharan et al., 2011).
Physicochemical and proximal analyses
The S. queretaroensis extract from the red fruit possesses an acidic pH of 3.78 (Table 1), similar to that of other types of S. queretaroensis (pH 3.9 - 5.2) such as yellow fruit (Gaytán-Andrade et al., 2019). Furthermore, the extracts from S. griseus and Opuntia ficus-indica have pH values of 4.2 and 6.04 ± 0.0 (Campos-Rojas et al., 2011; Ochoa and Guerrero, 2012). It is clear that although all these extracts are acids, variation exists in acidic values among them. The difference in pH could be due to the concentration of chemical constituents (Harris, 2016). Furthermore, the pH can vary depending on the phenological conditions of the plant and the maturity of the fruit because its composition is related to the conditions of harvesting, post-harvest storage, biosynthesis, geographical region, season and age of the plant (Hernández et al., 2017).
Table 1 Physicochemical and proximal analysis of the S. queretaroensis fruit extract.
Parameter | Value | Parameter | Value (%) w/w |
pH | 3.78 ± 0.1 | Moisture | 9.50 ± 0.7 |
Total soluble solids (° Brix) | 11.30 ± 0.6 | Ash | 0.00 ± 0.0 |
Titratable acidity (citric acid %) | 0.03 ± 0.0 | Crude fat | 0.00 ± 0.0 |
Color | Crude fiber | 0.77 ± 0.0 | |
L* (Lightness) | 17.21 ± 0.1 | Crude protein | 1.36 ± 0.0 |
a* (+ red, - green) | 38.61 ± 0.13 | Nitrogen-free extract | 88.82 ± 0.8 |
b* (+ yellow, - blue) | 10.60 ±0.23 |
Values are means ± standard deviation, n = 3.
The extract of the red fruit had a lower TSS content (ºBrix) (Table 1) than the fruit of the yellow one, which has 16 ºBrix; however, its content is similar to that of Hylocereus spp. juice that contains 10-12 °Brix (Chuah et al., 2008). The extract from S. queretaroensis fruit presents lower titratable acidity percentage (Table 1) than other fruits, including some species of Stenocereus. Since other types of S. queretaroensis have percentages of 0.15 to 0.50 % (Gaytán-Andrade et al., 2019) and fruits of Stenocereus spp. from 0.25 to 0.28 % (Campos-Rojas et al., 2011).
The production of citric acid corresponding to titratable acidity is due to the metabolic pathway of the plant and its phenological condition; therefore, its content varies according to the degree of maturity of the fruit. Furthermore, the citric acid content is a better indicator of flavor than pH (Sadler and Murphy, 2010); hence, the content detected in the S. queretaroensis fruit extract confers it a pleasant taste due to its low acidity. TSS and titratable acidity play a role in the final flavor and quality of the extract; for this reason, they are relevant parameters in the food industry, thus, they are used as a base in the formulation of products (Campos-Rojas et al., 2011).
The S. queretaroensis fruit extract displayed a reddish color and less luminosity (Table 1) than the Hylocereus spp. fruit pulp (L* = 51.35 ± 0.7, a* = 74.57 ± 0.4, b* = -1.34 ± 0.5) (Ochoa-Velasco et al., 2012). The luminosity index makes the S. queretaroensis fruit extract more attractive, and the color is associated with the composition and maturity index of each fruit (Ortiz and Takahashi, 2015). The parameters a* and b* of S. queretaroensis slightly point out toward red and yellow, respectively. This coloration is linked to the content of betalains in some Cactaceae (Monroy-Gutiérrez et al., 2017). At present, the color parameters of the extract of S. queretaroensis had not been described, but other studies showed that the whole fruit has L* 24.44 ± 0.6 to 34.44 ± 0.9, a* 5.82 ± 1.3 to 18.82 ± 0.5 and b* 8 ± 0.035 to 16.75 ± 1.2, which also correspond to shades of red and slight yellow (Soto-Castro et al., 2019).
On the other hand, the moisture of the lyophilized extract from the S. queretaroensis fruit was lower (Table 1) than that of the extract of S. queretaroensis fruits and other non-lyophilized Cactaceae fruits. Moisture of 97.52 ± 0.1 to 98.88 ± 0.0 % has been determined in S. queretaroensis (Soto-Castro et al., 2019), while is was 87.30 to 89.05 % in Opuntia joconostle (Contreras et al., 2011), 78.4 to 88.27 % in Opuntia ficus-indica and 81.68 % in Opuntia dillenii (Díaz et al., 2007). Nevertheless, lyophilization is a necessary step to standardize the extract on dry weight and to determine its retained moisture to establish the formulation of new products. Furthermore, knowing the moisture content allows establishing storage conditions to prevent microbiological damage (Qadri et al., 2015).
The proximal analysis showed that the lyophilized extract of S. queretaroensis fruit does not contain minerals (Table 1); however, the ashes of the fruit could be retained in the pulp during the filtering process since the ash content is found at considerable levels in the fruits of other Cactaceae; for instance, 6.67 % in S. griseus (Gaytán-Andrade et al., 2019) and 0.49 to 0.65 % in O. joconostle (Contreras et al., 2011). The fat analysis showed that the lyophilized extract of S. queretaroensis does not contain fat (Table 1). The fruits of other Cactaceae have low-fat contents, thus, the fat from the lyophilized extract could be retained in the fruit pulp during filtration; Opuntia dillenii contains 0.71 % (Díaz et al., 2007) and red O. ficus-indica contains less than 0.10 % fat (Repo de Carrasco and Encina, 2008). The lyophilized extract of S. queretaroensis showed a content of raw fiber (Table 1) lower than that of whole fruits from other Cactaceae. Because most of the fiber was retained during filtration, fiber content detected corresponds to the soluble fiber. The fruits of other Cactaceae have higher fiber contents; for example, O. joconostle contains from 2.35 to 4.28 % and O. ficus-indica from 0.38 to 3.9 % (Contreras et al., 2011). The fiber content detected in the freeze-dried extract of S. queretaroensis fruit has the potential to improve gastrointestinal function, as fiber improves the digestion of nutrients and chemicals.
The lyophilized extract of S. queretaroensis fruit showed a protein content higher or similar to that of other Cactaceae fruits; therefore, it represents a source of nutrients. Opuntia joconostle showed a protein content of 0.71 to 1.56 % (Contreras et al., 2011), O. ficus-indica of 0.9 to 1.5 % (Díaz et al., 2007; Repo de Carrasco and Encina, 2008) and O. dillenii of 0.52 ± 0.12. In addition, the S. queretaroensis extract showed a higher content of nitrogen-free extract (Table 1) than other Cactaceae as O. joconostle that contains 5.71-7.88 %, without considering the carbohydrate content, which is from 5.81 to 7.98 % (Contreras et al., 2011), while O. ficus-indica (red variety) contains 16.7 % of the nitrogen-free extract, without including the carbohydrate content, corresponding to 20.2 % (Repo de Carrasco and Encina, 2008).
The fruits of different crops of S. queretaroensis contain 10-11 % of total sugars, but total solids, suspended solids, and vitamin C have not been detected (Gaytán- Andrade et al., 2019). Stenocereus griseus fruit contains 9.9 % of total sugars (Chuck-Hernández et al., 2016); consequently, it is believed that a large proportion of the nitrogen-free fraction of the lyophilized extract of S. queretaroensis corresponds to carbohydrates, vitamins and other compounds not determined in this study.
Digestibility
Digestibility tests of edible plant extracts are scarce because the methods for theirin vitroevaluation are specific (Bornhorst and Singh, 2014; Brodkorb et al., 2019). In this context, no methods have been described to evaluate the digestibility of S. queretaroensis; nevertheless, simulated tests of oral in vitro digestibility (using saliva), gastric, and intestinal (small intestine and large intestine) are used for this purpose (Chen et al., 2018); hence, this study focused on establishing the conditions for enzyme consumption (amylase), solubility (in gastric fluid simulated with pepsin), and microbial consumption of the S. queretaroensis extract (Chen et al., 2018; Mkandawire et al., 2015). By this way, we could infer the content of nutrients in the residue that remains from each digestive process (Gargallo et al., 2006, Giraldo et al., 2007) by using simulated processes where oral, gastric, and intestinal (large intestine) digestibility were measured based on the weight of the lyophilized extract consumed. The extract had an oral digestibility proportion of 89.42 ± 0.1 %, while the gastric showed 72.80 ± 4.2 % and the intestinal 97.45 ± 1.1 % (Figure 1); that is, the digestibility of the extract is different because the release of nutrients is different in each digestive process, and depends on the nature of the food matrix (Guerra et al., 2012), as well as the mechanisms of degradation involved in each digestive process (Boland, 2016).
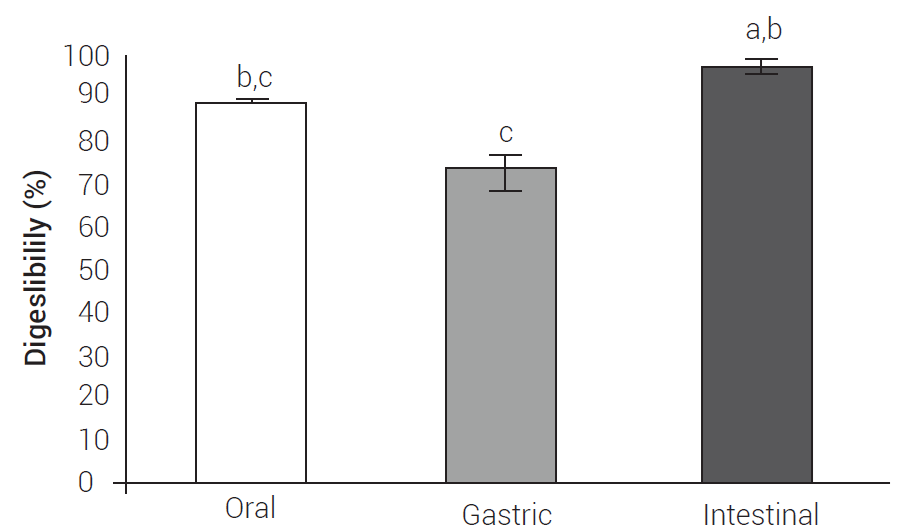
Figure 1 Fractions of the oral, gastric, and intestinal digestibility of the fruit extract of S. queretaroensis. Data represent the mean + standard deviation (n = 3). Bars with the same letters show non-significant difference between each other (Tukey, P ≤ 0.05).
Currently, no oral digestibility tests have been described for this fruit or any similar one; however, results show that the amylase in the saliva consumes the carbohydrates contained in the extract since, according to the proximal analysis, it contains 88.82 ± 0.8 % of the nitrogen-free extract, which in this case corresponds mainly to sugars. The components of the extract are dissolved or hydrolyzed by salivary amylase and turn into smaller digestible carbohydrates (Boland, 2016), which could be studied. Additionally, there are many aspects of extract digestion that must be addressed to properly understand how the components of this food interact when ingesting it, to create new foods in the future (Boland, 2016). The gastric digestibility of the extract was performed by combining two methods; the procedure was established according to the composition of the extract as determined in the proximal analysis since no digestibility methods have been described for this plant.
In the assay, pepsin was used to hydrolyze the proteins in the extract and to measure the total content of insoluble compounds that persist as the remnant of digestion. Although the main content of the extract, according to the proximal analysis, corresponds to carbohydrates calculated as the nitrogen-free extract, the residue could contain non-digestible carbohydrates, crude fiber, insoluble phytochemical compounds and amino acids from protein hydrolysis (Gargallo et al., 2006, Giraldo et al., 2007). Also, the gastric fluid contains pepsin that solubilizes its main component (carbohydrates). As a result, high digestibility values are obtained. The large intestine digestibility was adapted for humans by combining the DaisyII and Chen methods (Chen et al., 2018; Coblentz et al., 2019). Until now, only the DaisyII method has been described for ruminant digestibility. This method is efficient, fast, economical, and allows the simultaneous incubation of different samples in a jar (Giraldo et al., 2007).
Results revealed that the percentage of intestinal digestibility is larger than the sum of the content of crude fiber, crude protein and nitrogen-free extract (90.95 ± 0.8 %) because the intestinal microbiota has the enzymatic capacity to degrade these components and the phytochemical compounds in the extract, which would correspond to around 6.50 ± 1.4 %. Therefore, intestinal digestibility was estimated as the extract digested by the human microbiota of the fecal inoculum (Gargallo et al., 2006). As in the digestive process, the nutrients reach the intestinal microbiota to favor their growth and metabolite production (Ercolini and Fogliano, 2018); consequently, the microbiota protects the host from different diseases (Avolio et al., 2020).
Prebiotic evaluation
The growth of B. coagulans, L. sporogenes and L. delbrueckii was higher in the medium supplemented with the fruit extract of S. queretaroensis, where they had 10.88 × 1010 ± 0.0, 10.83 × 1010 ± 0.0 and 10.46 × 1010 ± 0.0 CFU mL-1 at 24 h, while the growth of these microorganisms on MRS medium was 9.97 × 1010 ± 0.0, 10.36 × 1010 ± 0.0, and 9.38 × 1010 ± 0.0 CFU mL-1, respectively.
The extract did not modify the growth of B. clausii and L. fermentum; these strains presented an exponential phase from 0 to 10 h, and then entered the stationary phase (Figure 2). During the exponential phase, MRS alone or added with extract contains the nutrients necessary for bacterial growth, which leads to cell division at a constant rate; however, when the microbial culture is consumed, it enters the stationary phase, so the microorganisms slow down their replication and die (Rolfe et al., 2011). Nevertheless, some strains have different growth requirements and have enzymatic mechanisms (Boll et al., 2014) to metabolize other carbon sources, such as the S. queretaroensis extract.
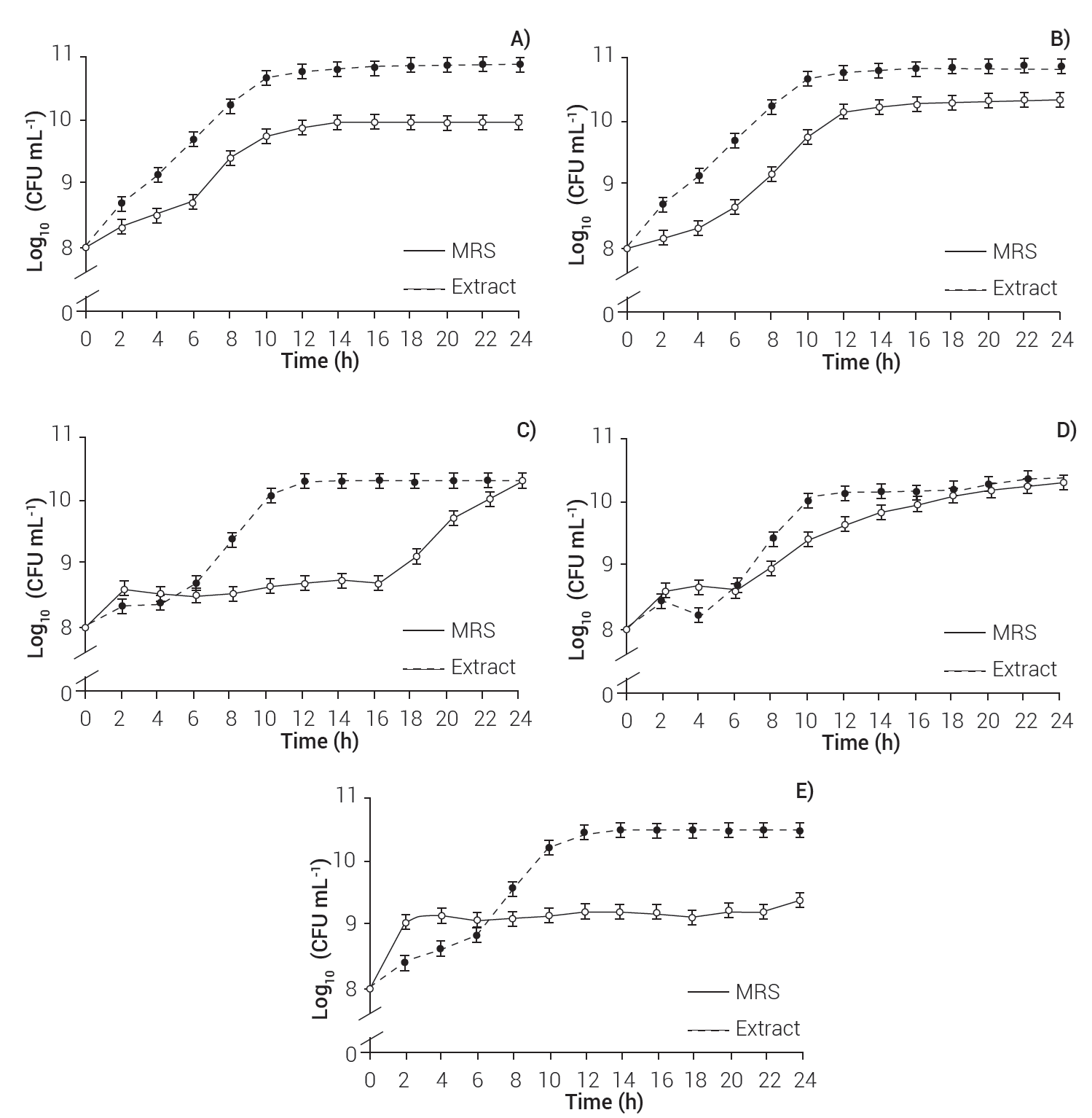
Figure 2 Kinetics of B. coagulans (A), L. sporogenes (B), B. clausii (C), L. fermentum (D), and L. delbrueckii (E) growth in MRS medium enriched with extract of S. queretaroensis and MRS medium as control.
Furthermore, the bacterial growth depends not only on nutrients but also on factors such as enzymes, temperature and incubation periods (Dalié et al., 2010). B. coagulans and L. sporogenes presented an exponential phase from 0 to 10 h, both in enriched medium and with MRS. L. delbrueckii presented an exponential phase from 0 to 12 h with the enriched medium and from 0 to 2 h with MRS; therefore, this strain accelerated growth because it quickly consumes nutrients. Accordingly, the enriched medium prolonged its exponential phase, as it provides an additional carbon source; however, B. coagulans and L. sporogenes (10 h) grew more in the enriched-medium than in MRS. These results suggest that extract from S. queretaroensis shows a prebiotic effect on B. coagulans, L. sporogenes and L. delbrueckii.
Bacillus coagulans and L. esporogenes showed growths of 10.67 × 1010 ± 0.0 and 10.49 × 1010 ± 0.0 CFU mL-1 with the enriched medium, and of 9.75 × 1010 ± 0.0 and 9.76 × 1010 ± 0.0 CFU mL-1 with MRS. Additionally, L. delbrueckii presented a growth of 10.42 × 1010 ± 0.0 CFU mL-1 (12 h) with the enriched medium and 8.40 × 1010 ± 0.0 CFU mL-1 with the MRS (2 h). These biomasses are superior to that of Lactobacillus casei produced in the MRS medium (7.3 × 108 CFU mL-1) (Jurado-Gámez et al., 2014); thus, growth depends on the nutritional requirements of each strain. Stenocereus queretaroensis extract possesses prebiotic properties and high potential to be used as food matrix to develop new functional foods.
Conclusions
According to the results, the method developed for the extract from the S. queretaroensis fruit showed higher yields than other methods reported for cacti. The extract has an acidic pH, titratable acidity, TSS, and color that make it pleasant for consumption. Lyophilized drying removes moisture, thus helping to prevent deterioration, very common in the fresh fruit of S. queretaroensis. The nutritional contribution of this fruit focuses on soluble crude fiber, protein content, and low nitrogen extract, which is related to high carbohydrate content; however, to strengthen knowledge about its nutritional value, it is necessary to deepen studies that include the profile of amino acids, vitamins, minerals and energy content. Furthermore, its nutritional composition is highly digestible at the oral, gastric and intestinal levels and gives it a prebiotic effect because its main component is a nitrogen-free extract, which is related to the content of highly soluble or degradable carbohydrates. Also, the developed methods could be useful to evaluate primary and secondary metabolites of the extract that are of interest to the food industry.